Traumatic Injuries of the Trigeminal Nerve
The use of universally accepted terminology is crucial when discussing nerve injuries. It is imperative that the appropriate terms be used to describe and document each case for communication among clinicians, determination of management strategies, and medicolegal reasons (Table 25-1). In addition, a detailed description, including nerve mapping with illustrations of the region with associated sensory changes, should be documented for each patient and used to quantify the initial presentation and, most importantly, to follow the progress of the area of involvement (Fig. 25-1).
TABLE 25-1
Select Terminology of Sensory Alterations
Term | Meaning |
Allodynia | Pain because of a nonpainful stimulus |
Analgesia | Absence of pain from a painful stimulation |
Anesthesia | Absence of any sensation from a painful or nonpainful stimulation |
Dysesthesia | Abnormal sensation, spontaneous or evoked, that is unpleasant |
Hyperalgesia | Abnormal response to a painful stimulus |
Hyperesthesia | Increased sensitivity to stimulation, excludes special senses |
Hyperpathia | Increased reaction to a (repetitive) stimulus. |
Hypoalgesia | Diminished pain in response to a painful stimulus |
Hypoesthesia | Decreased sensitivity to stimulation, excludes special senses |
Neuralgia | Pain in the distribution of a nerve or nerves |
Paresthesia | An abnormal sensation, spontaneous or evoked, that is not unpleasant |
FIGURE 25-1 Facial photographs with the areas of involvement marked are useful for monitoring progression.
Trigeminal Nerve Anatomy
Macroanatomy
Inferior Alveolar Nerve
The largest of the three branches of mandibular division of the trigeminal nerve (V3) descends between the medial pterygoid muscle and medial ramus and enters the mandible at the mandibular foramen (Fig. 25-2). At the lingula (the bony landmark for the mandibular foramina along the medial surface of the ramus), the inferior alveolar nerve (IAN) has the largest diameter, 2.4 ± 0.4 mm. At the mandibular foramen, the IAN diameter is 2.0 ± 1.1 mm. The mental nerve exits the mandible at the mental foramen and the course of the bony canal appears to be fairly predictable, based on cadaveric and conventional imaging studies.1–3 Proximally within the ramus, angle, and body region of the mandible, between the mandibular foramen, where the IAN enters the mandible to the second premolar region, the IAN follows an S-shaped course. This is where the nerve is most lateral in the third molar region and closest to the buccal cortex. It then approaches the lingual plate in the first molar region. Anteriorly, between the premolars, the IAN returns toward the buccal cortex prior to exiting the mandible through the mental foramen.
According to Cutright et al, the mental foramen was found to be apical to the second premolar in approximately 51% of cases; its distance from the midline was slightly different between whites and blacks, averaging 2.7 and 3.2 cm, respectively. It was noted that in whites the foramen is located between the premolars, whereas in blacks it is located distal to the second molar. In addition, the group noted small but significant differences between males and females for the three foramina (mental, infraorbital, and supraorbital).4 In the sagittal plane, the course of the IAN in the mandible begins superiorly and lingually at the lingula, approximately 10 mm from the sigmoid notch, and it reaches its lowest point at the first molar to second premolar region, with the distance from the inferior border of the mandible of 7.5 ± 1.0 mm, where it splits into the incisive and mental nerve branches. The incisive dental branch continues forward toward the incisors and the mental nerve ascends and loops backward for a variable distance (3 to 7 mm) to exit at the mental foramina through the buccal cortex.5,6
In their study of the mandibular canal, de Oliviera et al analyzed the bidimensional and tridimensional positions of the canal via multiplanar computer tomography (CT) morphometric analysis and provided useful average distances of the canal to the buccal and lingual cortices, inferior border, and alveolar ridge. The locations of the mandible for the measurements were selected to represent areas of commonly performed maxillofacial procedures associated with high risk for nerve injury, specifically extraction of third molars, sagittal split osteotomy, and dental implant placement. They did not find significant differences between the right and left sides, whereas in edentulous patients the typical values were lower in females than males and in older (from 51 to 75 years) than younger individuals (25 to 50 years).7
The mental nerve, the continuation of the IAN after it exits the mental foramen has two branches (range, one to four branches), with the largest being the labial branch running on the orbicularis oris muscle fibers. A smaller mucosal branch runs posteriorly on the buccinator muscle fibers. The labial branch innervates the lip and chin mucosa and overlying skin, but it may reenter the mandible to innervate the mandibular incisors and even cross the midline.8 The mucosal branch innervates the buccal mucosa and gingiva of the mandible as far posteriorly as the first and second molar, where cross-innervation with the buccal nerve may occur. Throughout its course in the mandible, the IAN provides branches for innervation of the mandibular teeth.9 Hu et al,10 in a study of the branching pattern of the mental nerve, identified four branches (angular, medial inferior labial, lateral inferior labial, and mental) and five branching patterns. They believed that this information on the branching could help the clinician to predict the area of sensory disturbance after injury of the mental nerve more accurately, depending on the cause and location.
Lingual Nerve
The lingual nerve (LN) branches from the posterior division of the third division of the trigeminal nerve, medial to the lateral pterygoid muscle. It is located medial to the IAN and supplies sensory and special sensory function to the anterior two thirds of the ipsilateral tongue, floor of the mouth, and lingual mandibular gingiva. The LN is joined by the chorda tympani as it passes through the pterygotympanic fissure, which provides taste (anterior two thirds of the tongue) and carries special visceral afferents (preganglionic parasympathetic fibers from the facial) to the submandibular and sublingual glands. Kim et al,11 in their study of the topographic relationship of the LN and IAN at the infratemporal fossa and paralingual space in 32 cadaveric hemisectioned heads, noted four patterns of furcation between the two nerves in relationship to the sigmoid notch and lingula (Table 25-2). In addition, the group measured the distance of the bifurcation to stable bony landmarks, including the foramen ovale and hamulus. The bifurcation was identified to occur on average 14.3 mm (range, 7.8 to 24.1mm) inferior to the foramen and 16.5 mm superior to the tip of the hamulus (range, 4.9 to 24.3 mm). This variation in the bifurcation patterns between the IAN and LN, as well as the collateral nerve branches at the retromolar region identified in 81.2% of the cases in their study, are thought to contribute to the reported failure rate of mandibular anesthesia.12
TABLE 25-2
Types of Furcation Of Inferior Alveolar Nerve And Lingual Nerve
Type of Furcation Between IAN and LN | Level of Furcation Between Sigmoid Notch and Lingula | No. per Type |
Type I | Separation occurs above notch and inferior to otic ganglion | 65.6% (21/32) |
Type II | Separation occurs below notch and at superior half of distance between notch and lingula | 28% (8/32) |
Type III | Separation occurs below notch and at inferior half of distance between notch and lingula | 3.1% (1/32) |
Type IV | Separation occurs in a plexiform pattern | 6.3% (2/32) |
The LN then passes into the pterygomandibular space between the ramus and medial pterygoid muscle, where it is surrounded by mesoneurial fat throughout its course. It enters the oral cavity over the superior pharyngeal constrictor, styloglossus, and mylohyoid muscles at the anterior border of the medial pterygoid muscle. As the nerve approaches the third molar region, it courses from a more lateral to a more medial position following the oblique flaring of the ramus. The exact location of the LN at the third molar region has been studied extensively, with some controversial findings among cadaveric studies, clinical observation during third molar surgery, and high-resolution magnetic resonance imaging (MRI) with regard to its proximity to the mandible.13–20 Table 25-3 summarizes the reported distances and relationship of the nerve to the mandible at the third molar region. In this location, the nerve has a diameter from 2.0 to 5.0 mm and may be round, oval, kidney-bean shaped, elliptical, or ribbon-like in cross section, with a predominance of one shape versus another varying among studies.11,18,20
At the second molar region, the LN crosses Wharton’s duct from a lateral to medial direction, traversing below the duct and hypoglossal nerve in the submandibular triangle. The nerve in this location gives off the preganglionic parasympathetic fibers from the facial nerve to the submandibular ganglion. These fibers are transected during submandibular gland excision so that the gland can be released from the nerve. It has been reported that the risk of LN injury during submandibular gland excision or sialodochoplasty of Wharton’s duct ranges from 1.4% to 4.8%, and the nerve is in danger from intraoral and extraoral approaches.21–24
After looping under Wharton’s duct, the LN passes upward onto the genioglossus muscle and enters the oral tongue muscular substance. With the exception of one or two large branches that proceed towards the tip of the tongue, all other branches to the tongue and adjacent lingual mucosa and gingiva are of small diameter (generally not >1 to 2 mm). The larger branches provide the greatest density of innervation to the tip and medial portions of the anterior two thirds of the tongue, compared with the posterolateral, dorsal, and ventral portions; this difference is developmentally driven.25
Buccal Nerve
The buccal nerve (BN) is a branch of the mandibular division of the trigeminal nerve that carries sensory fibers to the lower buccal, gingival, buccal sulcus, and cheek mucosa and may contribute to the cutaneous supply of the cheek. The nerve travels between the two portions of the lateral pterygoid muscle, beneath or through the lower part of the temporalis muscle deep to the mandible, and then crosses over the external oblique ridge at the anterior border of the masseter muscle and merges with the same branch of the facial nerve. This is where the nerve crosses over the external oblique ridge that places it at risk for injury, most commonly from third molar surgery. A rare variation that supports the IAN as the origin of the BN has been described by Turner, Singh, and Jablonski et al, who identified the nerve exiting the mandible through a small foramen at the retromolar fossa.26–29 As the nerve reaches the surface of the buccinator muscle, it divides into a superior division that supplies the muscle and an inferior division that provides the sensory innervation of the mucosa, as noted earlier.
Hendy et al,28 in their study of the course and relationships of the BN in 20 cadaveric specimens, noted the following:
• The nerve was found to have four to eight major branches, with a mean number of three branches where it crosses the external oblique ridge.
• The nerve trunk was found to cross the ridge as close as 3 mm from its deepest concavity of the external oblique ridge in 70% of specimens(14 of 20).
• In the remaining cases, the distance ranged between 7 to 12 mm below that point.
The authors postulated that the nerve is at risk from incisions in this region, even if these are placed as far away as 12 mm from the external oblique ridge. The variation of the branching patterns of the nerve, in addition to the high mechanosensory threshold noted on the buccal mucosa, may result in a small area of damage or in the injury not being noticeable by the patient.30,31
Infraorbital Nerve
The infraorbital nerve (ION) is a major branch and is the termination of the second division of the trigeminal nerve that emerges on the face via the infraorbital foramen. The foramen is on average 2.7 cm from the facial midline and 6 to 8 mm from the inferior orbital rim. In males, the foramen is larger than in females, with some differences in the distances from the midline and infraorbital rim identified in anatomic studies.4,32 In the study by Kazkayasi et al, a single foramen was found in 90% of cases, two canals in 5% and three canals in the remaining 5%. The shape of the canal was found to be oval in 30%, round in 40%, and semilunar in 30% of cases.33 The ION enters the orbit via the inferior orbital fissure and transverses the orbital floor in the infraorbital canal (IOC) for 22 mm. The canal may not be complete because the cortical thickness of its roof ranges from 0.1 to 6.0 mm; thus, the nerve may be exposed, without a discrete superior bony wall. The combination of a groove and canal is found in 50% of cases and the remainder demonstrate a complete canal.33 Racial and gender variations have been reported in the location and shape of the foramen and in the length of the canal.32,34,35 After it exits the infraorbital foramen, four branches of the ION have been identified and correspond to the areas of the midface, nose, upper lip, and lower lid innervated by the nerve36 The ION is at risk for injury during approaches for reconstruction of the orbitozygomaticomaxillary complex following traumatic events and during maxillary orthognathic surgery. Orthognathic-related injuries are typically transient and reversible, but sensory changes from entrapment of the nerve encountered in midface trauma may cause more problematic, long-term neurosensory changes.
Microanatomy
The microanatomy of all peripheral nerves is the same, with sensory and motor nerves containing myelinated and unmyelinated fibers in a ratio of 1 : 4. The difference between the two types of fibers is the number of Schwann cells that surround each fiber with nodes of Ranvier that permit rapid saltatory nerve conduction (Fig. 25-3). In the myelinated fiber, the ratio of nerve axons and Schwann cells is 1 : 1, whereas in the unmyelinated fiber the Schwann cell envelops several axons. The membrane, or basal lamina, created by the Schwann cell as it wraps around the axon and runs the entire length of the axon, is called a band of Büngner (laminar myelin sheath) and is crucial for the process of nerve regeneration. Although myelin may be destroyed during nerve injury, Schwann cells survive and play a major supportive role in nerve recovery and repair. The length of the axon surrounded by a single Schwann cell is known as the internode and the small area (0.3 to 2.0 µm) between the internodes, where the axon is not myelinated, is known as a node of Ranvier. In each node of Ranvier, certain ions diffuse, which causes nerve depolarization and repolarization and allows for the conduction of nerve impulses along the nerve fiber. This event—of the conduction occurring only between the nodes of Ranvier (saltatory conduction)—is responsible for the rapid impulse transmission noted in myelinated nerve fibers (up to 150 m/sec) compared with nonmyelinated nerve fibers (2 to 2.5 m/sec). Typically, unmyelinated axons are also smaller in diameter, with diameters ranging from 0.15 to 2.0 µm.
Collagen provides the framework that surrounds the nerve and creates the structural architecture within the nerve (Fig. 25-4). The endoneurium is the first organization of fine collagen fibers around each nerve fiber axon. In the pre–electron microscopy era, this organization was known as the Plenk-Laidlaw sheath or sheath of Key and Retzius.37,38 Several bundles of endoneurial groups, known as fascicles, are grouped together and surrounded by a second layer of collagen fibers (and mesothelial cells), called the perineurium. The outer layer of connective tissue supporting the nerve, along with some elastic fibers, creates the external or extrafascicular epineurium. Another layer of epineurium that invests the fascicles is known as internal epineurium. The epineurium makes up 50% of the cross-sectional area of the nerve and provides some protection against compression. The final layer of connective tissue that is continuous with the epineurium and surrounding tissue bed and is responsible for allowing some freedom of movement of the nerve is known as the mesoneurium, or adventitia of the nerve.37
Peripheral nerves are often distinguished based on their fascicular pattern, which may be of one of the following three patterns—monofascicular pattern with only one large fascicle (e.g., the chorda tympani), oligofascicular pattern, containing only some (two to ten) fascicles (e.g., the labial branch of the mental nerve), and polyfascicular, with more than 10 fascicles of different sizes (e.g., IAN, lingual, and buccal nerves). The mean number of fascicles and cross-sectional area for the trigeminal nerve branches decrease from the proximal to the distal nerve.39–42 Table 25-4 provides the reported observations regarding fascicular number and diameter for each branch of the trigeminal nerve.
TABLE 25-4
Number of Fascicles and Diameter of Branches of Trigeminal Nerve
Nerve | No. of Fascicles (mean) | Cross-Sectional Area (mean) |
IAN | 9.4 to 21 ± 7.05 (third molar region) | 1.64 ± 0.27 mm2 (third molar region) |
LN | 8.5 to 10 ± 4.0 (third molar region) | 1.87 ± 0.38 mm2 (third molar region) |
MN | 10.9 to 12 ± 3.58 | 1.18 ± 0.27 mm2 |
ION | 5 to 6 ( in the IOC) | 2 mm2 (in the IOC) 0.4 mm2 (after it exits the IOF) |
A rich interconnecting vascular network of extrinsic and intrinsic systems provides the blood supply for each neuron. The vessels reach the extrafascicular epineurium via the vasa nervosum after entering the mesoneurium and create a plexus that runs longitudinally at this level. The vessels continue their course toward the axon by passing in an oblique angle through the subsequent connective tissue layers and forming a longitudinally running plexus during their course at each level. This configuration allows for the individual fascicles to have an independent and rich blood supply, analogous to an individual nerve fiber43,44 (Fig. 25-5). This passage of the vasculature, between the layers of connective tissue, may become an area of compromise within the intrafascicular layers; there are no associated draining lymphatics running with the vascular plexuses.
Trigeminal Nerve Imaging
Objective, noninvasive, radiologic imaging modalities for the preoperative assessment of the patient at risk for nerve injury, as well as monitoring for the postinjury and postrepair phases of neurosensory recovery, are highly desirable.45 Radiologic assessment should be categorized with regard to the timing of the imaging period—that is, preinjury, postinjury, and postrepair phases. Preinjury assessment refers to the documentation of the in situ position of a nerve prior to any surgical intervention that places that nerve at risk for iatrogenic injury (e.g., third molar removal). Intraoperative monitoring of nerve function during a surgical procedure (e.g., sagittal split mandibular osteotomy) that involves a specific nerve may also be used, usually by a functional study of nerve conduction and electrophysiologic status, such as somatosensory evoked potentials (SSEPs).
Preoperative Risk Assessment
Panoramic Radiography
The preoperative assessment of the position of the inferior alveolar nerve during patient consultation has been routinely performed with the use of a panoramic radiograph. Obviously, the information gained from this study is extremely limited because of the two-dimensional nature of the image, variable magnification of the bony anatomy (for the inferior alveolar nerve), and complete inability to visualize the position of the lingual nerve. Valuable information can be obtained from the panoramic radiograph as a stand-alone imaging modality in regard to the relationship of the IAN in the vertical plane, but not in the horizontal dimension. The most useful aspect of the panoramic radiograph is for assessing the increased potential for inferior alveolar nerve injury during third molar extraction based on the presence of several radiographic predictors46,47 (Fig. 25-6).
Computed Tomography
The use of CT for the assessment of nerve injuries is limited. An evaluation of bone window attenuation images may indicate violation of the cortical outline of the inferior alveolar canal, either from implant placement or following facial trauma involving the posterior mandible fracture (Fig. 25-7), but yields little information regarding the condition of the nerve or neurovascular bundle.48 The use of soft tissue window images for the lingual or inferior alveolar nerves is compromised by poor-detail resolution, which precludes its routine application for neural assessment. Furthermore, dental artifacts often pose severe limitations in obtaining accurate information regarding the position of the LN to the lingual cortex of the mandible in critical areas, even in the soft tissue windows, and despite current high-resolution image acquisition.
In 1998, CT cone beam (CBCT) technology, until then used only in angiography, was used in the United States as a potential imaging modality for the maxillomandibular complex. The presurgical evaluation of the impacted mandibular third molar relationship to the IAN has gained popularity over CT scans and plain panoramic radiographs among surgeons49–53 (Fig. 25-8). The need for accurate imaging with the lowest possible dose of radiation (following the ALARA rule—as low as reasonably achievable) seems to be well satisfied with this technology. CBCT provides the desired three-dimensional representation of the anatomic location of interest with minimal distortion compared to traditional plain films and by simpler acquisition compared to CT systems.54,55 Similar to panoramic radiography, CBCT can be used for preoperative risk assessment in various dentoalveolar procedures (e.g., third molar surgery or dental implants and preprosthetic surgery).56–58 A major limitation remains the inability to visualize the lingual nerve, because no accurate soft tissue information can be obtained with CBCT.
High-Resolution Magnetic Resonance Imaging
MRI is the method of choice for visualization of all cranial nerves (CNs) and each nerve segment can be seen and examined in detail with specific sequences. Because of the complexity of the course and surrounding anatomic structures, detailed examination of the CNs is made possible only with careful planning and MRI technique selection. The imaging plane, coil selection, slice selection, in-plane resolution, and use of special techniques can be tailored based on the CN and segment of interest so that the highest quality image possible is obtained. The trigeminal nuclei (intra-axial), the cisternal (preganglionic), and Meckel’s cave (intradural) segments, contain the motor and sensory components of the trigeminal nerve and can be visualized with high-resolution T1- or T2-weighted MR images. At the anterior aspect of the gasserian ganglion, the sensory root divides into the ophthalmic, maxillary, and mandibular divisions, and each can be followed and examined separately based on their known course. The course of the lingual and inferior alveolar branches of the mandibular division, after it exits from the oval foramen, can be followed with high-resolution, contrast-enhanced, T1-weighted (T1W) or T1W three-dimensional fast field echo (T1W 3D-FFE) sequences in the axial, coronal, and sagittal or parasagittal planes.59–63 Although detailed information can be obtained with the use of MRI, routine presurgical evaluation of the route and integrity of the LN and/or IAN is not undertaken. Instead, MRI is used as the preferred imaging modality for examination of the status of the CNs in the presence of a disease process or injury.60,64–67
In an attempt to document the in situ position of the lingual nerve in the third molar region directly, without surgical manipulation or tissue distortion artifact, high-resolution MRI (HR-MRI) has been studied. In one study, 10 patients (20 sides) of healthy adult volunteers without prior dental surgery were imaged using an HR-MRI sequence (PETRA [phase-encoded time reduction acquisition]) that enabled visualization of the lingual nerve (Fig. 25-9). This study documented that the lingual nerve position, although variable, is found to be superior to the lingual crest in 10% of cases and in direct contact with the lingual plate in 25% of cases.18
Ultrasonography
Several reports have described the use of ultrasound (US) and high-resolution US technology, mainly for assessment of peripheral nerve lesions. This real-time advanced technology, with the currently available high-resolution probes, can offer compound imaging without radiation and in a relatively inexpensive manner. Although US has not been used nor investigated as a potential preoperative risk assessment tool for the trigeminal nerve, it has been demonstrated to be valuable in identification and safe advancement of the needle in brachial plexus and sciatic nerve blocks.68 It would be reasonable to anticipate limitations with the use of US in the examination of the IAN in the third molar region because of the presence of bone and teeth. Visualization and documentation of the course and integrity of the LN, on the other hand, should be relatively easy, requiring training and familiarity of the operator with the regional anatomy.
Postinjury Assessment
Panoramic Radiography
The postsurgery assessment of the nerve-injured patient usually includes a panoramic radiograph that may demonstrate clinically significant findings. The presence of a foreign body in the region of one or both nerves must be ruled out; these may include metallic foreign bodies from rotary instruments or amalgam particles from neighboring teeth, as well as retained tooth or root fragments following third molar surgery. The presence of an iatrogenic surgical disturbance of the nerves may be indicated by evidence of bone removal in proximity to the inferior alveolar neurovascular bundle or LN (Fig. 25-10). However, a panoramic radiograph or any other plain film is rarely used to monitor progression following injury or repair.
Magnetic Source Imaging
One of the few objective radiologic studies that documents inferior alveolar nerve injuries involves the use of magnetic source imaging (MSI), which combines magnetoencephalography (MEG) and HR-MRI. MEG technology uses magnetic fields to measure electrical brain activity; it is influenced less by intervening soft tissues than electroencephalography (EEG) and therefore produces a more detailed image, with higher resolution. Similar to SSEPs, a stimulus is applied peripherally and a signal is recorded centrally; this enables measurement of signal latency and amplitude. The information obtained from MEG is combined with HR-MRI images to produce a structural and functional magnetic source image of a particular region of the brain. McDonald et al69 used MSI on six patients with unilateral inferior alveolar nerve injury and have shown that MSI technology may be able to differentiate various grades of nerve injury. The findings on clinical and MSI examinations were correlated with surgical findings; neural continuity defects were identified as different radiographically from intact nerves. Despite some limitations of the study design (small n, lack of blinded examiners and surgeons, and other study designs flaws), there is potential for MSI to be applied in the postinjury and postrepair phases to monitor the progression of neurosensory recovery.
High-Resolution Magnetic Resonance Imaging
The application of the HR-MRI modality to the assessment of the nerve postinjury is in its early trial phases. Presumably, with improved resolution, a variety of anatomic changes in the nerve may be visualized. First, a change in nerve diameter may be visualized in cases of nerve injury with Wallerian degeneration of the nerve segment distal to the site of injury with an acute or gradual decrease in nerve diameter. Second, an acute change in nerve position may be seen—for example, where the lingual nerve is retracted into the region of the third molar socket, with the formation of a lateral adhesive or exophytic neuromatous segment. Third, a change in nerve shape may be seen—for example in a case of a fusiform neuroma-in-continuity, where a change in the shape of the nerve for a certain distance with return to normal shape distal to the neuroma may be able to be visualized.70
The application of HR-MRI to postinjury neural assessment is hindered by several factors. The degree of image resolution and magnification significantly limits precise anatomic examination of the neural elements. The ability to image the internal architecture of neural anatomy will require dramatic improvements in resolution from currently available techniques. Also, although the lingual nerve lies within soft tissue and its course is unaccompanied, the inferior alveolar nerve lies within a cortical bony conduit and is joined by an artery and a vein throughout its course. Preliminary studies with HR-MRI have allowed gross visualization of the lingual nerve because it is the sole structure in the area, but examination of the inferior alveolar nerve has been complicated by the presence of the vessels, although attempts to attenuate the image signal can overcome this problem (see earlier section on magnetic resonance neurography). Depending on the plane of image section, HR-MRI may miss several anatomic indicators of nerve injury. A transverse (or coronal, in the case of the lingual nerve in the third molar region) section of the nerve may not visualize a short discontinuity or abrupt alteration in the course of the nerve, depending on the distance between images. This may be avoided with the use of a sagittal or longitudinal image along the course of the nerve. However, this is difficult because the position of the nerve varies normally in the uninjured patient and may change significantly in the injured patient, thereby requiring patient repositioning or redirection of the imaging plane.71
The use of noninvasive, lack of radiation exposure HR-MRI for the nerve-injured patient would provide the advantage of correlating the results of clinical neurosensory testing and subjective patient evaluation with an objective assessment of the anatomy of the injured nerve site. Although it may seem that a frank transection injury (Sunderland grade V; see later) might be visualized easily with HR-MRI, the less severe injuries (Sunderland III and IV) could be extremely difficult to discern radiographically. Future study designs with HR-MRI include an experimental group of postinjury patients who will undergo clinical neurosensory testing and HR-MRI and then microneurosurgical nerve exploration and repair, if indicated. This would allow correlation of postinjury radiologic results and findings at the time of surgery to determine the ability of HR-MRI to predict the actual degree of anatomic nerve injury accurately. HR-MRI might also prove useful in monitoring the progression of anatomic neurosensory recovery (correlated with physiologic clinical signs and subjective symptoms) following nerve injury and/or microneurosurgical repair.45
Magnetic Resonance Neurography
Most research has focused on larger, peripheral motor nerves, including the brachial plexus, sciatic, peroneal, and femoral nerves.61,65,72–75 One report has documented nerve compression and signal hyperintensity of an IAN in a patient with a lymphoma of the pterygomandibular space. MRN has been able to document increased diameter of injured nerves, increased signal intensity, and longitudinal variations associated with nerve injury and recovery. There does not seem to be any correlation between the amount of hyperintensity and degree of neural injury, and its significance has not yet been clearly defined. The finding of signal hyperintensity has been demonstrated for a transient period following neural anastomosis and distal to a nerve graft site. The remarkable ability of MRN to depict fascicular architecture is based on the difference in fluid composition of the neural elements. The fascicles contain a preponderance of endoneurial fluid and axoplasmic water, whereas the interfascicular space is largely composed of fibrofatty connective tissue. In a sense, these images may be able to define radiographically the histologic characteristics of different grades of nerve injuries set forth by Seddon and Sunderland. Similarly, sequential images could be used to monitor nerve recovery at the fascicular level. One of the most advantageous characteristics of MRN images is the ability to image the nerve in a longitudinal plane. In a technique similar to that of MRA to image the anatomy of an abdominal aortic aneurysm, these MRN images can easily be assessed for variations in nerve anatomy, diameter, location, discontinuity, and signal intensity, which may indicate areas of nerve injury and thereby guide surgical intervention, as well as monitor neurosensory recovery.
Postinjury Functional Assessment
The current advances in MR imaging technology with high-resolution, functional, or metabolic-based images (BOLD [blood oxygenation level–dependent]) certainly allow for detailed examination of the neural structures, as well as associated pathology and nerve injury patterns.76 Perhaps the main potential limitations with the routine use of these advanced imaging modalities for the investigation of IAN and LN injury and recovery are the cost associated with these studies and the lack of expertise of neuroradiologists and surgeons regarding their interpretation and clinical significance.60,75,77–80
Classification of Trigeminal Nerve Injuries
Seddon Classification System
The Seddon classification system includes three levels of nerve injury (Fig. 25-11)—neuropraxia, axonotmesis, and neurotmesis.81 In neuropraxic injuries, the integrity of the axon is maintained, and the injury indicates a local conduction block from a transient anoxic event caused by acute vascular interruption of the epineurial or endoneurial vasculature. Neuropraxia is usually the result of a mild nerve manipulation, traction, or compression injury; it is characterized by a reversible conduction block with a favorable outcome, with rapid and complete recovery within days to a few weeks of the event. No axonal degeneration occurs in neurapraxic injuries and da/>
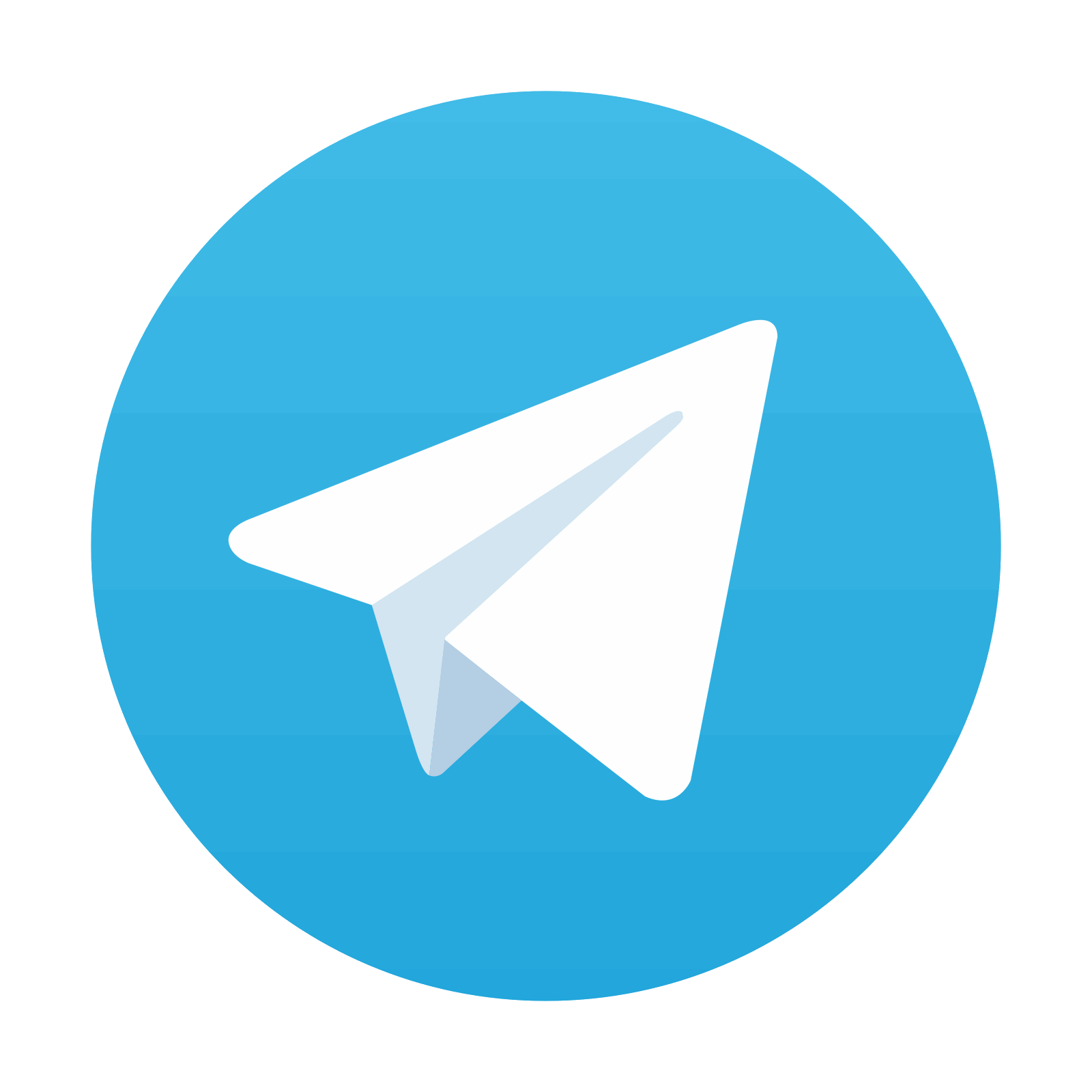
Stay updated, free dental videos. Join our Telegram channel

VIDEdental - Online dental courses
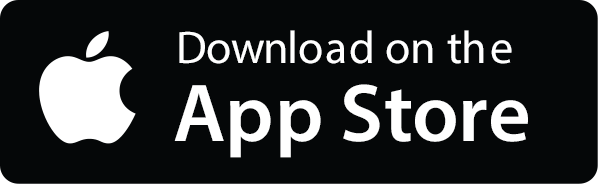
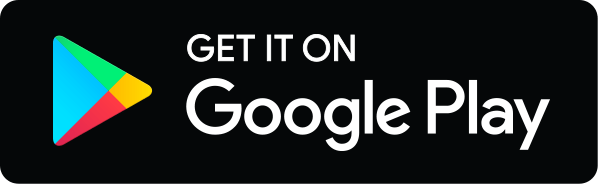