Chapter 14
Emerging Technologies in Root Canal Disinfection
Anil Kishen and Annie Shrestha
Discipline of Endodontics, University of Toronto, Toronto, ON, Canada
Nestor Cohenca
Department of Endodontics and Pediatric Dentistry, University of Washington, Seattle, WA, USA
Introduction
Inactivating biofilm bacteria and their by-product in the root canal systems is one of the main goals in root canal disinfection. In this context, bacteria existing as a biofilm pose a major challenge as they confer them greater immunity against antimicrobials and disinfectants (Figures 14.1 and 14.2). Disruption of the biofilm polymeric structure and killing of the resident microbes would provide a comprehensive approach in improving root canal system disinfection. Different antimicrobials and/or treatment strategies either single or in combination could be applied to eliminate biofilms (Figure 14.3). It includes the application of antimicrobials that (i) produces slow destruction of the biofilm structure, (ii) destroys persister cell or quorum sensing signals in a biofilm, (iii) diffuses into the biofilm structure producing biofilm bacterial killing, (iv) is used in combination with other strategies that enhance its diffusion into the biofilm structure, and (v) destroys both the biofilm matrix and resident bacteria in a biofilm structure (1).
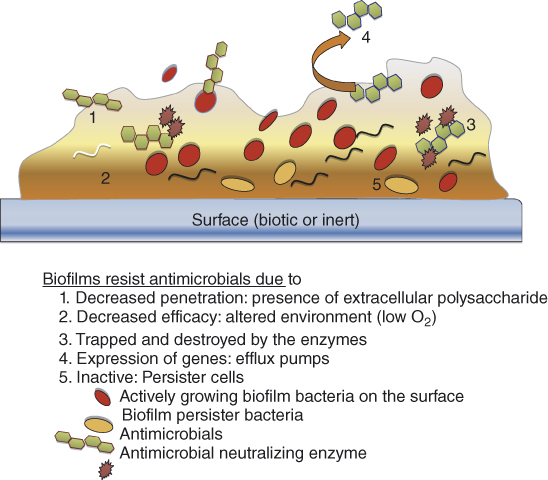
Figure 14.1 Schematic diagram showing different methods by which bacteria in a biofilm gain resistance against antimicrobials.
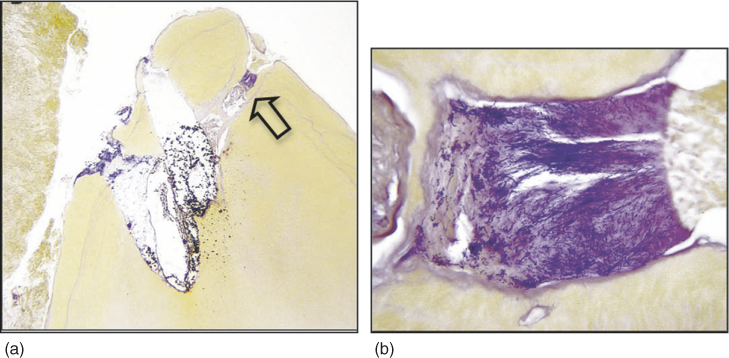
Figure 14.2 Histopathologic evaluation of a root-end section from a retreatment case with symptoms of persistent infection. (a) The overview of the mesiobuccal root tip. Three ramifications are present. Soft tissue does not appear in close contact with the root tip. This is an artifact caused by partial detachment during surgical procedure (Taylor’s modified Brown and Brenn, original magnification ×25). (b) Ramification on the right side in (arrow). Its lumen is filled with a large biofilm arranged against inflammatory cells (Taylor’s modified Brown and Brenn, original magnification×400).
(Ricucci et al. (2). Reproduced with permission of Elsevier.)
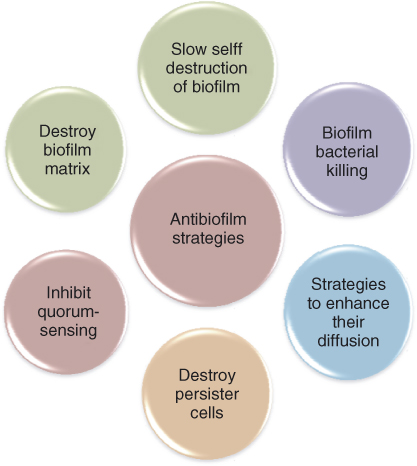
Figure 14.3 Schematic diagram showing different antibiofim strategies.
Recently, antibiofilm strategies are also directed toward preventing biofilm formation. Toward this purpose, biomaterial surfaces are modified with chemicals or surface preparations to hinder bacterial adherence and subsequently biofilm formation (3, 4). Considering the nature of challenges offered by the root canal environment and microbes, ideal disinfection strategies should eliminate the biofilm structure and destroy the resident bacteria completely even from the uninstrumented portions and anatomical complexities of the root canal system. During this process, it is crucial that the therapeutic method does not cause any physical, mechanical, and/or chemical changes to the root dentin. Moreover, inadvertent seepage of antimicrobials into the apical region should not cause cytotoxic effects in the apical tissues. Different emerging disinfection methods that are developed and tested in endodontics are discussed in this chapter.
Antibacterial nanoparticles
Nanoparticles are particles with at least three of its external dimensions in the nanoscale. Typically nanoscale size ranges from 1 to 100 nm.
The quantum size effect of nanoparticles provides them unique physicochemical properties that are different from their bulk or powder counterparts. One of the key features of antibacterial nanoparticles is the broad spectrum of antimicrobial activity and far lower propensity to induce microbial resistance than antibiotics. The antibacterial activity of nanoparticles also depends on the material (i.e., organic/polymeric or inorganic/metallic), size/surface area, and charge density (5). The electrostatic interaction between positively charged nanoparticles and negatively charged bacterial cells and the accumulation of large number of nanoparticles on the bacterial cell membrane has been associated with the loss of membrane permeability and rapid loss of membrane function.
Heavy metal ions are known to have different effects on bacterial cell functions (6–8). Copper ions induced oxidative stresses (9) and affected the redox cycling, resulting in cell membrane and DNA damages. Zinc ions, above the essential threshold level, inhibited bacterial enzymes including dehydrogenase, which in turn impeded the metabolic activity (10). Silver ions inactivated proteins and inhibited the ability of DNA to replicate (11). Metallic nanoparticles synthesized from powders of silver (Ag), copper oxide (CuO), and zinc oxide (ZnO) are currently used for their antimicrobial activity (12).
The initial interaction of microorganism with a tissue or biomaterial surface is adherence and is recognized to be an important step in the establishment of biofilm-mediated infections (13–15). Bacterial adherence experiments have highlighted that endodontic irrigants reduced the posttreatment adherence of Enterococcus faecalis to root dentin. Nevertheless, chemicals that alter the physicochemical properties of dentin may influence the nature of bacterial adherence and adhesion force to dentin. Final irrigation with ethylenediaminetetraaceticacid (EDTA) following sodium hypochlorite (5.2%) produced minimum reduction (33%) in the bacterial adherence to root dentin. EDTA (17%, pH 7.3) after 5 min application produced 20–30 µm zone of demineralization in dentin (16, 17). The exposed collagen fibers in this demineralized dentin served as an excellent substrate for the binding of E. faecalis. Thus care should be practiced to flush the root canal with sodium hypochlorite as the final irrigant to minimize the propensity of bacterial adherence (18, 19).
The quantum size effect of nanoparticles permits them to exhibit superior interaction with bacteria and dentin substrate. When cationic nanoparticles in an aqueous suspension are allowed to settle onto dentin surface, they adhered to the dentin surface by electrostatic interaction. Although this interaction between nanoparticles and dentin is weak and easily disrupted, they can impede bacterial recolonization and biofilm formation (18). Different studies pertaining to the use of nanoparticles for root canal disinfection has been summarized in Table 14.1 (3, 20–26). Among the polymeric nanoparticles, chitosan has been researched extensively owing to its excellent antimicrobial and antifungal activities. Chitosan is a natural nontoxic polymer derived by the deacetylation of chitin. Though the exact mechanisms of antibacterial action of chitosan and its derivatives are still not vivid, the electrostatic interaction between the positively charged chitosan nanoparticles and negatively charged bacterial cell membrane is believed to alter bacterial cell permeability and loss of function (27). A recent study examined the antimicrobial properties of ZnO and resin based root canal sealers loaded with chitosan and ZnO nanoparticles (3). This study highlighted that the addition of antibacterial nanoparticles in root canal sealers would improve the direct (based on direct antibacterial assay) and diffusible antibacterial effects (based on a membrane-restricted antibacterial assay) in root canal sealers. Studies also showed that the application of chitosan nanoparticles reduced the adherence of E. faecalis to root dentin.
Table 14.1 Summary of relevant studies carried out using antimicrobial nanoparticles in endodontics.
No | Author/date | Objectives and materials | Methodology | Conclusion |
1 | Waltimo et al. (2007) | To test the hypothesis that nanometric bioactive glass 45S5 releases more alkaline species, and consequently has a better antimicrobial effect, than the currently available micron-sized 45S5 bioactive glass | Ionic dissolution profiles were monitored in simulated body fluid. Antimicrobial efficacy was assessed against clinical isolates of E. faecalis (planktonic form) | Micron to nanosize treatment materials afforded a 10-fold increase in silica release and solution pH Substantially increased killing efficacy |
2 | Kishen et al. (2008) | (1) To investigate the physical and antibacterial properties of different nanoparticulates and nanoparticulates-mixed zinc oxide-eugenol-based endodontic sealer (2) To examine the ability of different nanoparticulates-treated dentin to prevent adherence of E. faecalis |
E. faecalis in planktonic Zinc oxide nanoparticulates, chitosan (CS) nanoparticulates, a mixture of zinc oxide and CS nanoparticulates, and zinc oxide nanoparticulates with multilayered coating of CS were tested |
Significant reduction in the adherence of Enterococcus faecalis to nanoparticulates-treated dentin CS-highest antibacterial Combination-highest leaching property |
3 | Waltimo et al. (2009) | To assess the continuous release of alkaline species and antibacterial effect of Bioactive glass-Nano/Micron combination | Antimicrobial effectiveness was tested in extracted human premolars mono-infected with 3 weeks old E. faecalis biofilm | Nano BAG did not show any antibacterial efficacy |
4 | Mortazavi et al. (2010) | To evaluate the antibacterial effect of bioactive glass nanopowders (58S, 63S, and 72S) of different size and compositions | The antibacterial activity was studied using Escherichia coli, Pseudomonas aeruginosa, Salmonella typhi, and Staphylococcus aureus. Cytotoxicity of the samples was evaluated using mouse fibroblast L929 cell line | Antibacterial activity decreased with decrease in size and showed no cytotoxicity |
5 | Pagonis et al. (2010) | To study the in-vitro effects of poly(lactic-co-glycolic acid) (PLGA) nanoparticles loaded with the photosensitizer methylene blue (MB) and light against Enterococcus faecalis (ATCC 29212) | 3 days old E. faecalis biofilm in root canals E. faecalis species were sensitized in planktonic phase and in experimentally infected root canals of human extracted teeth with MB-loaded nanoparticles |
Significant reduction of biofilm Colony forming units (CFU) levels were significantly lower than controls and MB-loaded nanoparticles without light PLGA nanoparticles encapsulated with photoactive drugs may be a promising adjunct in antimicrobial endodontic treatment |
6 | Chogle et al. (2011) | To evaluate two nanoparticle-enhanced polymer root-end filling materials (NERP1 and NERP2) on the initial apical seal as compared to a polymer-based commercial compomer C18 organoclay |
Dual-chamber leakage apparatus and inoculated coronally with Enterococcus faecalis Turbidity of the apical broth was assessed daily for 4 weeks as a sign of initial leakage |
The addition of C18-nanoparticles to a monomer matrix significantly reduced apical microleakage in an in-vitro environment |
7 | Shrestha (Kishen*) et al. (2010) | To test (1) the efficacy of chitosan (CS-np) and zinc oxide (ZnO-np) in disinfecting and disrupting biofilm bacteria and (2) the long-term efficacy of these nanoparticulates following aging | E. faecalis in planktonic and biofilms |
|
8 | Shrestha and Kishen (2012) | CS in the presence of tissue inhibitors | E. faecalis in planktonic | Pulp and bovine serum albumin (BSA) significantly inhibited the antibacterial effect |
Root dentin treated with chlorhexidine and subsequently with nanoparticulate showed the maximum reduction (97%) in bacterial adherence (3). Antibacterial substantivity have been highlighted after using 2% chlorhexidine gel (28); however, chlorhexidine did not entirely remove bacteria from the dentinal tubules of teeth that were infected with E. faecalis (29). Addition of nanoparticles into the root canal sealers showed improved antibacterial property and did not affect the flow characteristics of the root canal sealer. Also, ZnO nanoparticles were able to diffuse out from the sealer and exert antibacterial activity, which is definitely advantageous in a posttreated root canal environment (3). These polymeric and metallic nanoparticles were also able to eliminate bacterial biofilm and withstand the effect of aging for 90 days (conditioning with tissue fluids) on their antibacterial properties. The planktonic E. faecalis were totally eliminated in contrast to the biofilm bacteria. Longer duration of interaction and higher concentration of nanoparticles were required to reduce the biofilm bacteria in significant numbers (Figure 14.4) (22).
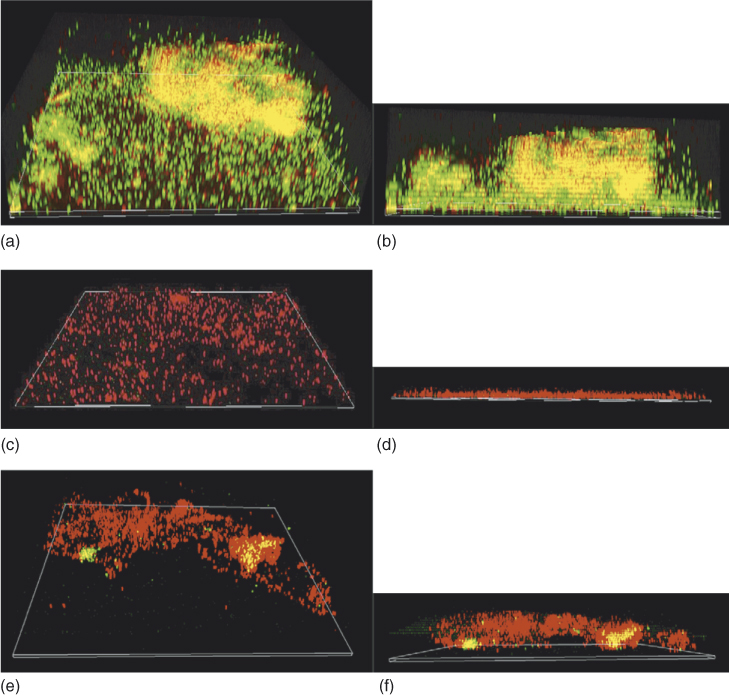
Figure 14.4 The three-dimensional confocal laser scanning microscopy (CLSM) reconstruction of Enterococcus faecalis (ATCC 29212) biofilm (a, b) and after treatment with antibacterial ZnO-np (c, d) and CS-np (e, f). The number of live bacterial cells was reduced significantly, and the three-dimensional structure was also disrupted. (b, d, and f show the sagittal sections of the biofilm structure) (original magnification, 60×).
(Shrestha et al. (22). Reproduced with permission of Elsevier.)
Microparticles and nanoparticles of bioactive glass received considerable interest in root canal disinfection following its antibacterial properties. The bioactive glass consists of SiO2, Na2O, CaO2, and P2O5 at different concentrations. The antibacterial mechanism of bioactive glass has been attributed to a combination of several factors such as (i) high pH, (ii) increase in osmotic effects, and (iii) Ca/P precipitation (30). The feasibility of using bioactive glass for root canal disinfection has been tested in-vitro (31–33). However, bioactive glass showed shortcomings such as significantly less antibacterial effect when compared with calcium hydroxide (32) and ineffective in preventing recontamination of instrumented root canals (31). 45S5 bioactive glass suspensions/slurries for root canal disinfection should combine the ability to induce high pH with the capacity to continuously release alkaline species (21).
The bioactive glass nanometric slurry with a 12-fold higher specific surface area than the micrometric counterpart was expected to possess better antibacterial properties. Nevertheless, the microparticles of bioactive glass produced considerably higher alkalinity and antimicrobial efficacy and this did not support the previous report by the same group that showed higher antibacterial efficacy with the shift from micron- to nanosized treatment materials (20). In another related application, bioactive glass was used to promote mineral deposition within the root canal, which could ultimately replace the use of endodontic sealers. Toward this end, a combination of polyisoprene or polycaprolactone and nanometric bioactive glass 45S5 was employed. Incorporation of bioactive glass fillers into polyisoprene and polycaprolactone rendered the resulting composite material bioactive and permitted improved mineralization (34). These initial observations need further research to apply the composite of polyisoprene, polycaprolactone, and bioactive glass as a “single” root canal filling material.
The studies so far showed that most tested nanoparticles possess high antibacterial properties when compared to their powder counterpart. Though they have the ability to diffuse antimicrobial components deep into the dentin tissue, additional research is needed to study their ability to inactivate bacterial biofilms in the anatomical complexities and uninstrumented portion of the root canal system. Their interaction with host tissues/immune cells also requires further investigations. Nevertheless, the successful application of nanoparticles in endodontic disinfection will depend on both the effectiveness of antimicrobial nanoparticles and the delivery method used within the root canal system.
Antimicrobial photodynamic therapy
Photodynamic therapy applies a photosensitizer, that is, a light sensitive chemical at extremely low and nontoxic concentration, which, when activated with a specific wavelength of light, produces activated oxygen radicals that cause toxic effects on the bacterial cells.
Antimicrobial Photodynamic Therapy (APDT) involves two specific steps for achieving disinfection. Step-1 is the photosensitization of the infected tissue with photosensitizer to allow uptake into the bacterial cells and Step-2 is the irradiation of the photosensitized tissue to result in the destruction of bacteria and infected tissue. The light should be at a specific wavelength, which corresponds to the absorption wavelength of the photosensitizer used. The photosensitizer in the triplet state is extremely reactive, which reacts further by one or both of the following pathways to destroy the cell. (1) Type I reaction: the photosensitizer triplet state can react with a target, other than oxygen by hydrogen or electron transfer resulting in radical ions that can react with oxygen, yielding cytotoxic species such as hydrogen peroxide, superoxide anion, hydroxyl, and lipid derived radicals. (2) Type II reaction: the photosensitizer triplet state can transfer the excitation energy to ground state molecular oxygen to produce excited state singlet oxygen (1O2) (35) (Figure 14.5).
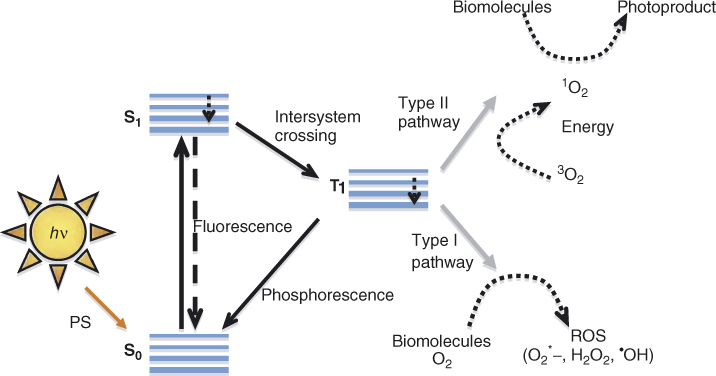
Figure 14.5 Schematic diagram of possible mechanism of photodynamic therapy where the excited photosensitizer (PS) after light irradiation could follow different pathways to return to its stable ground state. The PS on photoactivation absorbs a photon and goes to the excited singlet state (S1). The S1 can come back to the ground state by releasing fluorescence or relax back to excited-triplet PS state (T1). The long-lived T1 state can phosphoresce and come back to S0. Or else T1 can interact with molecular oxygen following two specific pathways Type I and Type II mechanism, resulting in the generation of reactive oxygen species or singlet oxygen, respectively. The singlet oxygen generated in Type II mechanism then interacts with biomolecules to produce the resultant PDT effect (photoproduct).
Singlet oxygen is a strong oxidizing agent and thus highly reactive, with a lifetime of less than 0.04 µs in a biological environment and a radius of action of less than 0.02 µm (36). The reactions of singlet oxygen with the cellular targets lead to cell death. The two basic mechanisms that have been proposed to account for this lethal damage to the bacterial cell are DNA damage and cytoplasmic membrane damage. APDT on gram-positive and gram-negative bacteria have reported breaks in both single and double-stranded DNA and the disappearance of the plasmid supercoiled fraction (37, 38). Previous studies have shown that the photo-oxidative effect caused by phenothiazinium photosensitizer in bacteria could lead to damage of multiple targets in bacterial cells such as DNA (37), membrane integrity (39), protease activity, and lipopolysaccharide (LPS) (40). George and Kishen (41) reported functional impairment of cell wall, extensive damage to chromosomal DNA, and degradation of membrane proteins following methylene blue mediated APDT of E. faecalis. These findings support the hypothesis that APDT can be a feasible alternative to antibiotics as the mode of action is markedly different from that of most antibiotics.
In endodontic literature, Meire et al. (42) and George and Kishen (43, 44) used APDT to enhance the root canal disinfection. They showed that biofilms of E. faecalis could be effectively killed by APDT with photosensitizer such as methylene blue and toluidine blue O (TBO) along with red light. Soukos et al. (45) conducted APDT experiments on a range of endodontic pathogens (methylene blue as photosensitizer) and reported complete elimination of all bacteria except E. faecalis (53%). In yet another study, significant antibacterial effect on suspensions of Streptococcus intermedius, Peptostreptococcus micros, Prevotella intermedia, and Fusobacterium nucleatum were reported by Williams et al. (46) following APDT with TBO and red light. Some of the tissue specific challenging factors in the application of APDT for endodontic disinfection are the penetration of the activating light energy into the infected tissue, penetration of optimum photosensitizer concentration into the infected tissue, limited availability of environmental oxygen in the infected tissue, the ability of excess photosensitizer to induce discoloration of dentin, and reduction or neutralization of antimicrobial activities following the presence of tissue and serum within the root canals.
In an approach to improve the antimicrobial efficacy of APDT in root canal system (tissue specific approach), George and Kishen dissolved methylene blue in different formulations: water, 70% glycerol, 70% polyethylene glycol, and a mixture of glycerol:ethanol:water (MIX) in a ratio of 30:20:50, and analyzed for the photophysical, photochemical, and photobiological characteristics (43). They showed that aggregation of methylene blue molecules was significantly higher in water when compared to other formulations. Other than this, the MIX based methylene blue formulation had effective penetration into dentinal tubules, enhanced singlet oxygen generation, which in turn improved bactericidal action. A significantly higher impairment of bacterial cell wall and extensive damage to chromosomal DNA was observed when methylene blue in a MIX based formulation was used, when compared to water (41). The same group also showed that the incorporation of an oxidizer and oxygen carrier with photosensitizer formulation in the form of an emulsion would produce significant photo-oxidation capabilities, which in turn facilitated comprehensive disruption of matured endodontic biofilm structure (44).
The selectivity of APDT toward microbial cells elimination over host cells, at the suggested photosensitization periods and light fluence, presents itself as a preferable choice among antibacterial alternatives. Soukos et al. compared the effect of APDT using a combination of TBO and red light against Streptococcus sanguis and human gingival keratinocytes and fibroblasts. They reported no reduction in the human cell viability whereas the bacteria were effectively killed (47). Soncin et al. reported the selective killing of Staphylococcus aureus over human fibroblasts and keratinocytes (four to sixfold) when subjected to APDT using cationic pthalocyanine and relatively low light fluencies (48). George and Kishen (49) demonstrated a 97.7% killing of E. faecalis compared to a 30% human fibroblast dysfunction following methylene blue mediated APDT. All these in-vitro studies suggested the targeted killing efficacy of APDT.
Conjugating photosensitizer to various agents or chemical moieties can result in improved photosensitizers for APDT. These modified photosensitizers are expected to bind to the outer membrane of bacteria and on activation generated reactive oxygen species, which then diffused into cells, resulting in cell death (50, 51). Therefore, photogenerated oxidative species is confined to the cell wall and its vicinity, which is a highly susceptible domain for photodynamic action. Soukos and coworkers formed a hypothesis that by covalently conjugating a suitable photosensitizer to a poly-l-lysine chain, a bacteria-targeted photosensitizer delivery vehicle could be constructed that would efficiently inactivate both gram-positive and gram-negative species (51). This was demonstrated by preparing a conjugate of chlorin (e6) and a poly-l-lysine chain (20 lysine residues), which after 1 min incubation and illumination with red light, killed greater than 99% of the gram-positive Actinomyces viscosus and gram-negative Porphyromonas gingivalis (51). Photosensitizer conjugated with positively charged chitosan has also been shown to be highly effective in removing biofilms of gram-positive and gram-negative bacteria (52). Shrestha et al. (53) showed that the rose-bengal conjugated chitosa/>
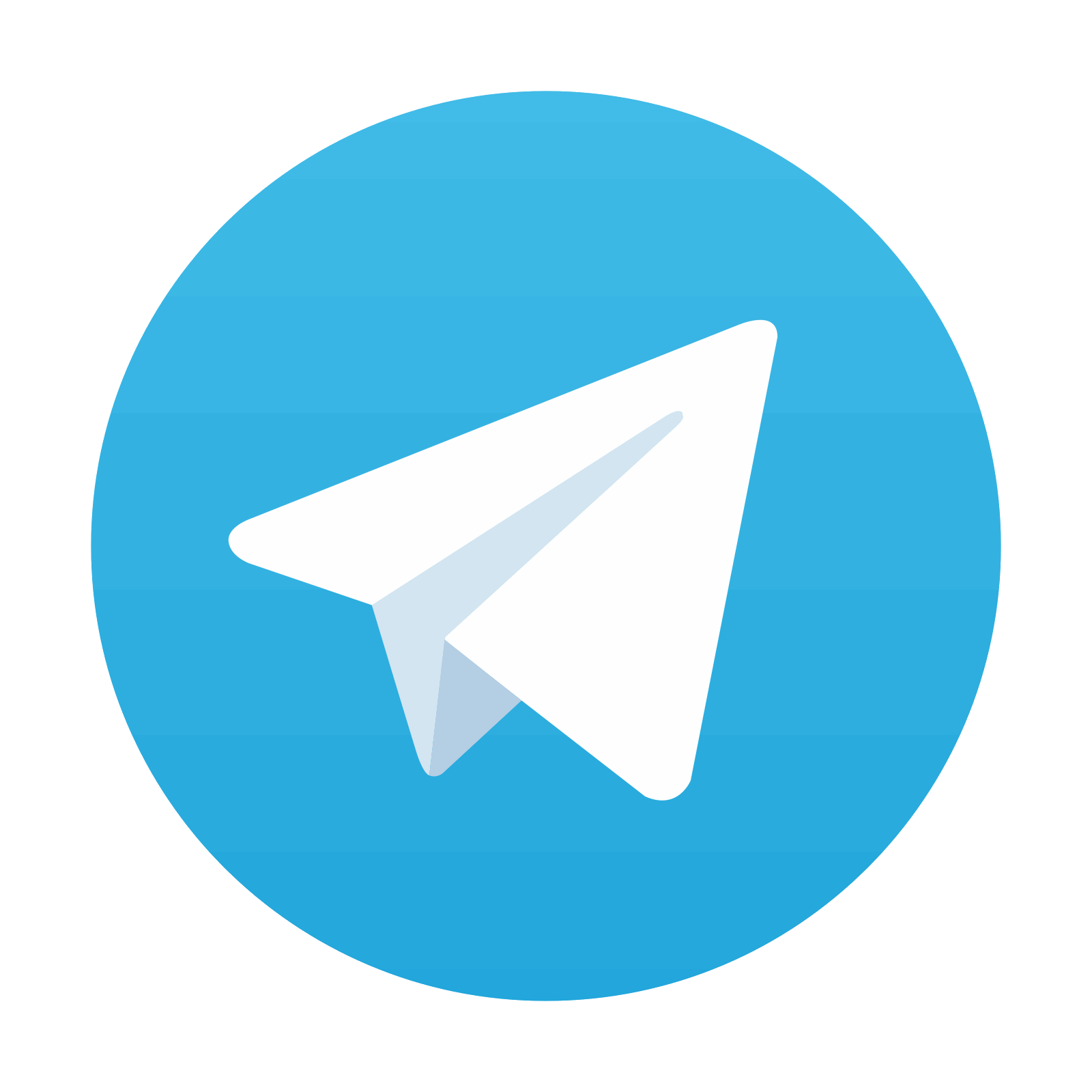
Stay updated, free dental videos. Join our Telegram channel

VIDEdental - Online dental courses
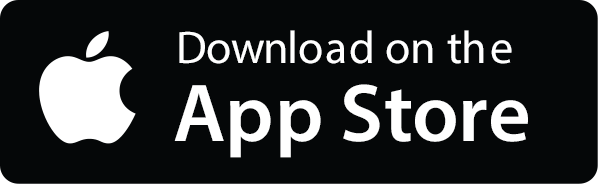
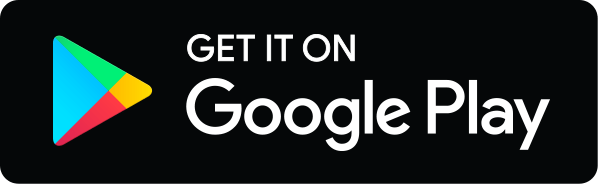