10 Orthodontic Adhesive Resins
Porosity of a two-phase, chemically cured mix of liquid orthodontic resins. Transmitted light, original magnification × 150
Enamel-Adhesive Resin Interface
Classification of Orthodontic Adhesive Systems
Chemically Activated Orthodontic Adhesive Systems
Thermocured or Heat-Activated Systems
Degradation of Polymeric Systems
General Principles and Background
Leaching of Orthodontic Adhesives
Introduction
Bonding of brackets to enamel has been a critical issue in orthodontics research, since the significance of achieving a stable bond between a tooth and its bracket was obvious from the outset. Biomechanical principles required a relatively inelastic interface that would transfer a load applied to the bracket, due to engagement of an activated archwire, to the tooth without exceeding its bond strength. Clinicians soon became aware of the problems related to low bond strength and the resulting necessity of repeating the bonding procedure as treatment progressed.
Early bonding systems consisted of brackets welded onto bands bonded to enamel with zinc phosphate cement. Apart from aesthetic considerations, this approach presented other serious disadvantages:
• The requirement of extensive chair time.
• The necessity of frequent screening for development of caries or decalcification of underlying tooth structure (Chapter 6).
• The pronounced effect on periodontal health due to chemical and mechanical irritation of the gingiva caused by cements and the accumulated plaque.
• The requirement of additional arch space to accommodate placement, thus affecting consideration of extraction in borderline cases and complicating the debonding owing to the interdental spacing present.
Therefore the need was clear for an alternative procedure that would provide retention of the brackets to tooth enamel without the aforementioned drawbacks of brackets welded to bands.
The introduction of an acid-etching technique in the 1950s to bond dental restorations to tooth structure was the breakthrough point in the history of orthodontic bonding. During the mid 1950s there were some reports on direct bonding of orthodontic attachments to teeth with the use of epoxy resins, followed by the introduction of epoxy acrylates in the early 1960s and the utilization of Bis-GMA resin composites in the mid 1960s. Although conflicting evidence has been presented regarding the first use of bonding in orthodontics, the development of new adhesives and the use of known substances to provide a continuous interfacial layer with the acid-etched enamel took place in the early 1950s. The main issue at that time dealt with the extent to which orthodontic bonding temporarily altered the ion exchange between the enamel and the oral environment. This effect, along with the need for a debonding procedure that would not affect the enamel, were the main factors that influenced research trends in the field.
Consequently, the majority of the studies in this area of orthodontics have focused on evaluation of the physical and mechanical properties of the adhesive resins and sealants used for direct bracket bonding, including the early and long-term bond strength and the condition and appearance of the enamel after debonding. The main problem was the elimination of the resin layer that remained adherent to the enamel, with the resin tags penetrating into the enamel being the most important issue. These resin tags were usually subject to some discoloration due to the aging process and absorption of oral fluid components. This tooth discoloration caused some aesthetic problems and obvious patient concerns. Advances in the field have led to the development of new materials with improved properties.
Enamel-Adhesive Resin Interface
The characteristics of the tooth enamel and orthodontic brackets have been described in Chapters 6 and 7, respectively, and Chapter 5 presented an extensive description of studies that have evaluated the bonding of brackets to enamel. The purpose of this section is to reveal new evidence on the enamel morphology and structure following etching and primer application.
It has been documented that the micro-mechanical retention of resin composites to acid-etched enamel may not be due only to formation of resin tags but also to the formation of an interfacial resin-enamel interdiffusion zone within the lateral sites of the remaining enamel protuberances (Fig. 10.1). This is similar to intertubular dentin hybridization in dentin adhesive systems and may explain the high bond strength values obtained from enamel, even when decreased acid-etching times are involved (Fig. 10.2). Incorporation of several hydrophilic monomers into enamel bonding agents facilitates resin infiltration of etched enamel at the prism level, reducing interfacial porosity and thus bonding defects. Based on these concepts, several new orthodontic adhesives have been introduced to improve bond strength and interfacial integrity. However, it must be emphasized that enhancement of bond strength may compromise safe debonding, as previously discussed in Chapters 5 and 7.
Fig. 10.1 Schematic representation of the bonding mechanism between (ortho) phosphoric acid-etched enamel and orthodontic resin adhesives. The original concept of micromechanical retention due to resin tag formation (a) has been revised. Acid treatment of longitudinally-sectioned, etched enamel-resin interfaces removes enamel protrusions, whereas enamel regions below the resin tags are not affected owing to resin infiltration, which encapsulates enamel prisms and makes the region acid-resistant (b)
Fig. 10.2 Secondary electron images of a sectioned interface between an orthodontic adhesive and phosphoric acid-etched enamel. The interface was treated with acid for 20 seconds to dissolve enamel protrusions and to reveal the extent of resin infiltration into etched enamel of the periphery and the bottom of resin tags (arrows). (a) Original magnification ×400. (b) Original magnification ×1000. (c) Original magnification ×3000
Bonding Agents
Unfilled resins have traditionally been utilized as bonding agents in resin composite bonding systems. The basic difference between these fluid bonding resins and the resin composites is the absence of filler particles in the former. The compositions of these systems differ from those of their composite counterparts in the increased proportion of the comonomer (e.g., TEGDMA; see Chapter 9) relative to the monomer. The use of these unfilled resins is based upon their lower viscosity and thus superior diffusion into the enamel rods, resulting in improved interfacial adaptation. However, conflicting evidence exists in the dental scientific literature regarding the necessity of their use in bonding systems. The problem arising from their use pertains to their contribution to bond strength, which has not been clearly defined.
Observations of the polymerization mechanisms and the postcuring properties for the bonding agents have been reported. Since the polymerization shrinkage is directly related to the resin volume, the unfilled resins are expected to experience higher shrinkage, thus potentially reducing the extent of enamel coverage. Also, the incorporation of a higher percentage of comonomer in these materials may result in excessive shrinkage. In addition, these unfilled resins have been found to exhibit increased water sorption, as well as an increased thickness of the oxygen-inhibited layer, because of two factors: the enormous surface-to-volume ratio involved, and the clinical procedure for placement of the resins on the enamel surface.
The first factor is exaggerated because of the period that elapses before these resins are cured. Evidence in the research literature suggests that there is no detrimental effect on bond strength for a wide range of time intervals between sealant application and bracket bonding. However, the preceding discussions in Chapters 2, 5, 7, and 9 indicate that a simple measurement of bond strength cannot adequately describe the complex phenomena occurring in polymers that are in contact with treated enamel surfaces. Moreover, there should always be concern when trying to extrapolate the results of in vitro tests to in vivo conditions.
The second factor, relating to clinical technique, arises because an increased thickness of the bonding agent on the enamel surface results in a weakened interface, owing to greater polymerization shrinkage and thermal expansion of the resin matrix. The absence of filler particles in these materials may further exaggerate these effects. Accordingly, air-thinning has been advocated clinically for decreasing the thickness of the bonding resin. However, it has been shown that with this procedure the bulk oxygen concentration in these resins may reach 60 ppm, introducing defects in the bulk material that contribute to increased water sorption and monomer leaching (in addition to the effect of oxygen on inhibiting polymerization). Since the reactivity of the oxygen-free radical complex is much higher than that of the monomer-free radical complex during the initial stage of polymerization, inhibition of polymerization occurs for the layers exposed to the air (Fig. 10.3).
Oxygen inhibition of polymerization has a detrimental effect on properties of the adhesive, causing a variation in hardness and other physical properties between the surface layers and the bulk resin. Under normal clinical conditions, the extent of inhibition depends upon both the setting time and the concentration of the inhibitor. Reactions with the affected surface may include the following:
• Formation of “oxyplex” compounds, which quench the excited states (free radicals) and subsequently suppress the polymerization process. Quenching of these excited states may lead to the production of highly reactive, single oxygen atoms.
• Scavenging of the free radical species and alteration of the polymerization mechanism.
Two different approaches have been utilized to avoid the diffusion of the oxygen into the polymerizing composite:
• Shortening of the exposure time to the air prior to complete curing. This approach includes the utilization of more powerful curing lights, incorporation of faster-reacting monomers, use of an increased photoinitiator concentration, and introduction of synergists or coinitiators to accelerate the surface cure.
Fig. 10.3 The extent of oxygen inhibition of a visible-light-cured resin (between black and white arrows). This inhibited zone is 20 μm thick. Transmitted light, original magnification × 100
• Use of some means to block the resin from oxygen. Polymerization can be carried out under an inert gas, while petroleum jelly or glycerol coatings have been used to protect the resin composite from oxygen in industrial applications.
Moreover, air-thinning may induce film leveling problems and result in an uneven cured film thickness, which may lead to stress distribution problems. As a practical rule, the application of bonding agents using brushes with long and thick bristles may result in increased amounts of material being placed on the enamel surface, along with increased inclusion of air.
Classification of Orthodontic Adhesive Systems
Based upon the polymerization initiation mechanism, orthodontic adhesives may be classified according into the following groups (Table 10.1):
• Chemically activated (also termed chemically cured, autocured or self-cured): two-paste or one-paste
• Light-cured (also termed photocured)
• Dual-cured (chemically activated and light-cured)
• Thermocured
Chemically Activated Orthodontic Adhesive Systems
Chemically cured orthodontic adhesive systems have been used since the initiation of bonding in modern orthodontic history. Specific products have been utilized since the early 1970s. However, the parameters of their in vitro performance have not been well defined, as noted in a critique of bond strength studies by Fox et al. Moreover, the in vivo behavior of these adhesives is poorly understood and is far from being simulated through ex vivo approaches. Upon reviewing 22 articles on the bond strength of a widely used chemically cured (two-phase) commercial product, Fox et al found that variations in tooth type, storage conditions, method of debonding, analysis of results, and selection of other products for comparison resulted in none of the studies having the same methodology. Consequently, despite the large number of previous publications, these authors concluded that the bond strength of this commercial product had not been properly studied.
As noted in Chapter 9, the chemically activated orthodontic adhesives employ benzoyl peroxide as an initiator, which is activated by a tertiary aromatic amine such as dimethyl-p- toluidine or dihydroxyethyl-p-toluidine. Initiation occurs from mixing of the paste and liquid components of these systems, and free radicals are formed by a multistep process.
Two-Phase (Two-Paste) Adhesive Systems
The two-phase products were the first to be tried by orthodontists in the early days of bonding. Their application involves mixing the paste and liquid components. The manipulative process is problematic, relatively time-consuming, and cumbersome, and these materials are gradually being eliminated from very active orthodontic practices. Mixing of the two components introduces potentially critical defects such as surface porosity and air voids in the bulk material, owing to the prolonged exposure to air and the inevitable entrapment of air bubbles. Studies have shown that photo-cured composites, intentionally mixed as if they were chemically cured materials, also demonstrated severely porous surfaces and air voids in the bulk materials. The pore volume in the bulk composite was found to be of the order of 3%, while the surface porosity reached nearly 50% (Fig. 10.4). The degree of cure (DC) for these materials has been found not to exceed 55% (Fig. 10.5). The disproportionately high amount of monomer leaching compared to the relatively high DC for a chemically cured adhesive observed by Eliades et al is attributed to the mixing process and to the detrimental effect of air entrapment on the carbon double-bond conversion in the vicinity of voids. The sizes of these regions of residual monomer in the cured adhesive are unknown, and their effects on biocompatibility (Chapter 14) require further study.
Fig. 10.4 Porosity of a two-phase, chemically cured mix of liquid orthodontic resins. Transmitted light, original magnification × 150
Fig. 10.5 Multiple internal reflectance Fourier transform infrared (FTIR) spectra of (a) a two-paste chemically cured adhesive (Concise) and (b) a visible light-cured adhesive (Transbond XT), before (curve A) and after setting (curve B). The visible light-cured adhesive was irradiated for 40 seconds. The reduction in the peak absorbance area ratio of the aliphatic carbon double-bonds (C = C) at 1638 cm−1 to the aromatic carbon single-bonds (C-C) at 1605 cm−1 between A and B states, respectively, is greater in the visible light-cured adhesive, implying a better conversion
One-Phase Adhesive Systems
The principle of inhomogeneous polymerization was introduced in orthodontics with the development of the no-mix bonding resins, which were intended to minimize the mixing-induced defects and to reduce the steps required for placement of the material. In these systems, a catalyst gradient is established from the primed enamel surface toward the brackets by means of a diffusion process. Under these conditions, the resin strength is presumed to be decreased by the establishment of a disturbed cross-linked network, which nonetheless may facilitate bonding.
However, recent evidence suggests that the DC for these adhesives is comparable to that of the two-paste systems for surfaces in contact with the enamel. This might be attributed to the surface-to-volume ratio of the adhesive layer, which can depend upon several factors. A study found that the thickness of adhesive layers prepared under simulated clinical conditions ranged from 120 to 230 μm, depending upon the morphology and design of the bracket base. The mean layer thickness is especially increased when complex bases possessing grooves and crystal-like projections (Chapter 7) are pressed into the adhesive, presumably owing to the retention of material in the base. However, this effect depends strongly on the topography of the base and the viscosity of the adhesive. In general, sharp crystals protruding from the base should act to increase the local stress on the adhesive. In contrast, smooth bases would be expected to transfer the applied force more uniformly to the adhesive layer. It is further assumed that, when applying the bonding agent to the tooth surface and bracket base, the diffusion process required for polymerization is intensified by pressin/>
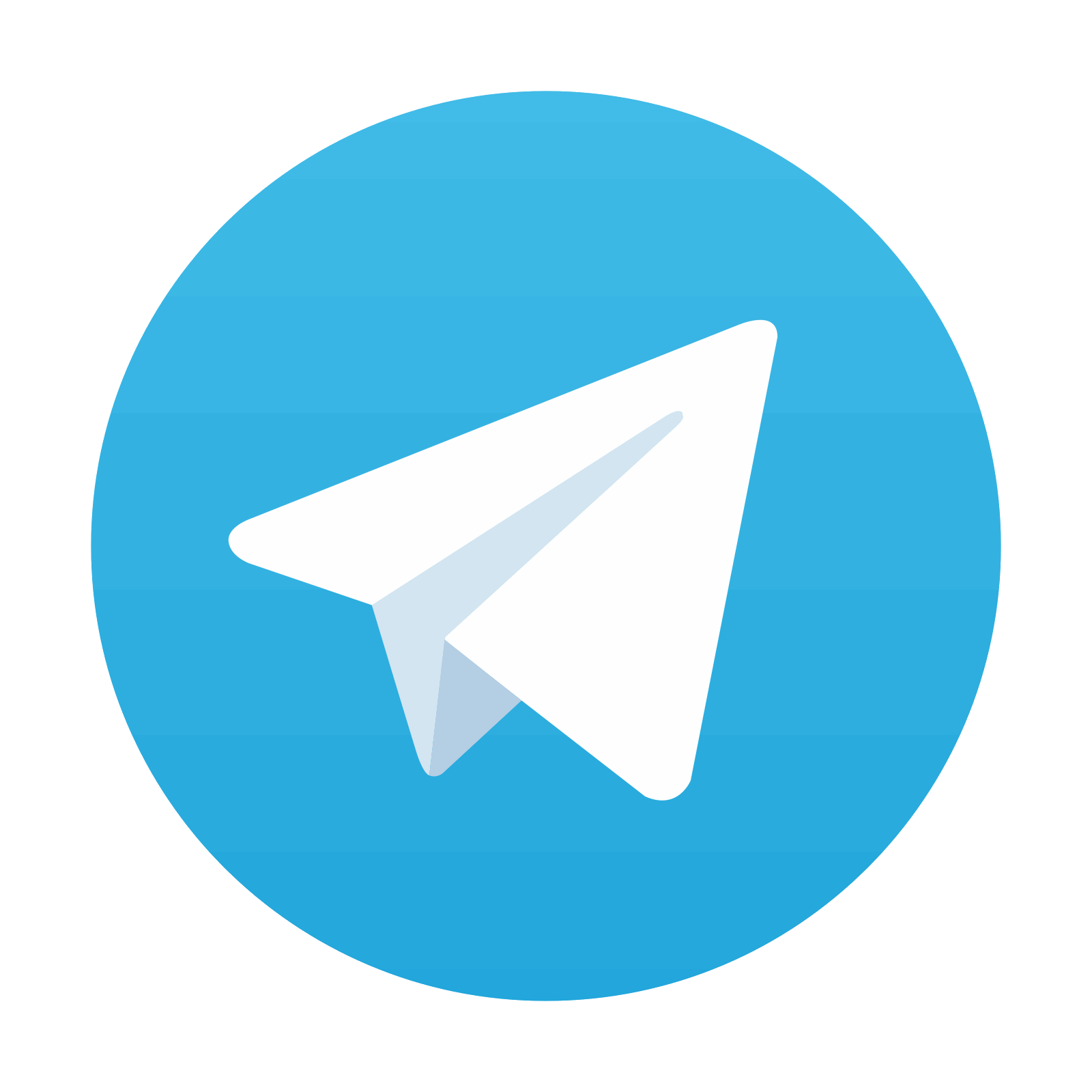
Stay updated, free dental videos. Join our Telegram channel

VIDEdental - Online dental courses
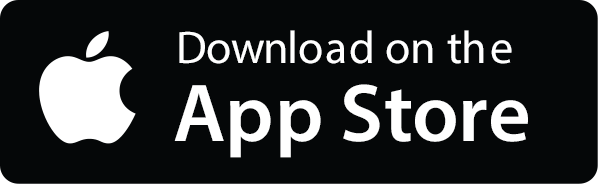
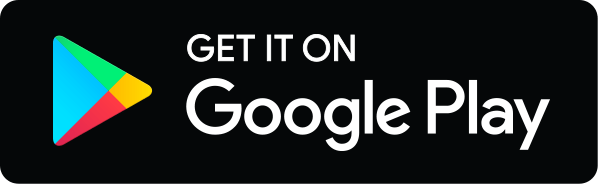