Highlights
- •
PICN has high wear resistance against steatite antagonist.
- •
Low impact on surface roughness for PICN by wear.
- •
Zirconia reinforced lithium disilicate is less abrasive against steatite.
Abstract
Objectives
The aim of this laboratory study was to evaluate the wear resistance of crowns made from current computer-aided design and computer-aided manufacture (CAD/CAM) materials. In addition, the abrasion of the steatite antagonist against these materials was compared.
Methods
Identically shaped crowns of lithium disilicate, zirconia-reinforced lithium disilicate and a polymer-infiltrated ceramic network (PICN) were fabricated with an occlusal thickness of 1.5 mm and a lateral wall thickness of 1.2 mm (n = 8). The crowns were cemented with a dual-polymerizing luting resin on composite resin dies. Using spherical steatite antagonists, all specimens were loaded with 49 N for 1,200,000 cycles in a mastication simulator with additional thermocycling. After 120,000, 240,000, 480,000, 960,000, and 1,200,000 cycles, precision impressions were made and investigated with a laser scanning microscope. The vertical and volume substance loss was measured. Additionally, the substance loss of the antagonists was evaluated after 1,200,000 loading cycles.
Results
No significant difference (p > 0.05) was found in the median volume loss of the test materials after 1,200,000 cycles (lithium disilicate: 0.405 mm 3 , PICN: 0.362 mm 3 , zirconia-reinforced lithium disilicate: 0.340 mm 3 ). The vertical substance loss of PICN (157 μm) was significantly lower (p ≤ 0.05) than that of lithium disilicate (201 μm) and zirconia reinforced lithium disilicate (191 μm). However, the substance loss of steatite against zirconia-reinforced lithium disilicate (0.191 mm 3 ) was significantly lower (p ≤ 0.05) than against lithium disilicate (0.296 mm 3 ) and PICN (0.531 mm 3 ).
Significance
All three CAD/CAM materials showed wear resistance that seems appropriate for clinical application. Also, the abrasion of the antagonist looks promising.
1
Introduction
All-ceramic restorations are popular in contemporary dentistry [ , ]. In addition to their good mechanical and chemical properties, dental ceramics provide better biocompatibility and esthetics than dental alloys [ ]. As computer-aided design and computer-aided manufacturing (CAD/CAM) provides the possibility of efficient chairside production, the demand for monolithic restorative materials is high [ ]. Although highly esthetic monolithic silicate ceramic has been successfully used for small single tooth restorations, there are reports about complete fractures of large restorations, including crowns [ , ]. The reason for this is the brittleness and fragility of silicate ceramics; therefore, dental restorations made of silicate ceramics are only reliable if an adequate thickness is maintained [ ].
Compared with conventional silicate ceramics [ ], lithium disilicate ceramic has a higher flexural strength and fracture strength [ ] and is clinically well proven for large restorations [ ]. However, to minimize tooth reduction as much as possible, CAD/CAM materials that allow thinner crown designs are required.
Polymer-infiltrated ceramic networks (PICN) are formulated to combine the benefits of the polymers and ceramics. Unlike conventional dental composite resins, which have ceramic filler particles in an organic matrix, PICNs are composed of a ceramic network that is infiltrated with a polymer in order to imitate the mechanical properties of a natural tooth. The mechanical properties of PICN, including the modulus of elasticity, flexural strength, and fracture toughness, have been investigated, and promising results have been reported for the material’s flexibility and stability [ ]. In addition the fatigue resistance of PICN crowns during mastication simulations and/or crown fracture tests showed a high stability [ , , ]. While the wear behavior of PICN has been investigated, many of these studies investigated only the short-term behavior, performing 120,000 or fewer cycles [ ], and few studies performed tests that corresponded to a life time of 5 years [ , ]. The results of these studies seem to be contradictory, as one study reported major wear after mastication simulations [ ], while two studies indicated that the occlusion of PICN was quite stable [ ] and showed only minor traces of wear [ ]. A fourth study showed lower vertical substance loss in PICN than in ceramic materials [ ].
Another approach to enhancing the mechanical properties of silicate ceramics is their reinforcement with zirconia. The flexural strength and fracture strength of a lithium silicate ceramic including 10% zirconia are higher than the corresponding values of lithium disilicate [ ]. In addition, crowns fabricated of zirconia-reinforced lithium silicate showed promising results in a crown fracture test before and after fatigue by mastication simulation [ ]. Nevertheless, few studies have measured the two-body wear behavior of this material [ , , ], and only two studies have investigated the long-term behavior (1,200,000 loading cycles simulating 5 years of function). Both studies indicated that the zirconia-reinforced lithium silicate ceramic causes minimal damage to the antagonist natural tooth but is sensitive to vertical and volume material loss [ , ].
As natural teeth also show abrasion, the phenomenon of wear is a physiological process. Any restorative material might affect the wear of the antagonist teeth [ ]. Ideally, the restorative material should not increase the wear of antagonist enamel [ ]. However, reduced wear of the occlusal surface may lead to periodontal or functional problems by initiating occlusal interferences in the long term [ ]. Only few and partly contradictory studies concerning the long-term wear behavior of PICN and zirconia- reinforced lithium silicate are available, and, to the best of the authors’ knowledge, none study that investigate the progress of wear during mastication simulation that corresponds to five years of function.
Therefore, the study aimed to investigate the progress of wear of a PICN material and a zirconia-reinforced lithium silicate compared with that of the clinically well-documented lithium disilicate. In addition, the total wear of the antagonist material was investigated. The first null hypothesis of the study was that no difference would be found in the progress of wear of the three tested CAD/CAM materials. The second null hypothesis was that the total wear of the antagonist material would be independent of the test material.
2
Materials and methods
2.1
Specimen preparation
A typodont model of a first molar (KaVo Dental, Bieberach/ Riß, Germany) was prepared with an axial reduction of 1.2 mm and an occlusal reduction of 1.5 mm according to the usual dimensions of a ceramic crowns. A preparation angle of 4° was prepared by using special drills fixed in a parallelometer. Silicone impressions were then made, and replicas were produced by filling these impressions with resin-based composite (Z100, 3M ESPE, Seefeld, Germany) and polymerizing the composite according to the manufacturer’s instructions. To stabilize the water uptake of the resin-based composite die after crown cementation [ , ], the replicas were stored in distilled water (37 °C) for a minimum of 30 days before crown fabrication.
A complete crown wax pattern was created with the dimensions 1.2 mm/1.5 mm on the die. To facilitate the abrasion measurements, the cusps were designed as planes with an opening angle of 150° as shown in Fig. 1 . The anatomic contour waxing was scanned, and the design finalized by using the 3D scanner and a software program (Sirona 3shape scanner, Sirona Dental GmbH, Salzburg, Austria). Eight crowns of each of the three test materials were milled ( Table 1 ). No glaze was used. After polishing the crowns with the aid of magnification loupes (4.5 magnification Zeiss, Jena, Germany) and specific rotary instruments (Polishing Set clinical, VITA Zahnfabrik, Bad Säckingen Germany), the crowns were cemented, and the composite resin dies conditioned according to the manufacturer’s instructions by using a dual-polymerizing luting composite resin (Multilink Automix, Ivoclar Vivadent, Schaan, Liechtenstein). The crowns were conditioned by using an adhesion promoter (Monobond Plus, Ivoclar Vivadent, Schaan, Liechtenstein) according to the manufacturer’s instructions. After 24 h storage in distilled water (37 °C), a 0.9-mm steel wire was added to the root part of the specimens to prevent any movement during mastication simulation. Afterwards the specimens were embedded in a polyester resin (Technovit 4000, Kulzer, Wehrheim, Germany).
Material | Manufacturer | Composition | LOT |
---|---|---|---|
IPS e.max CAD | Ivoclar Vivadent, Schaan Liechtenstein | Lithium disilicate ceramic (SiO 2 57−80%, Li 2 O 11−19%, ZrO 2 1−8%) |
T08295 |
VITA Enamic | VITA Zahnfabrik H. Rauter, Bad Säckingen, Germany | Polymer-infiltrated-ceramic-network (Inorganic ceramic part 86% (58−63% SiO 2 , 20−23% Al 2 O 3 ), organic polymer part 14% (UDMA, TEGDMA) |
44640 100013 |
VITA Suprinity | VITA Zahnfabrik H. Rauter, Bad Säckingen, Germany | Zirconia reinforced lithium silicate (SiO 2 56−64%, Li 2 O 15−21%, ZrO 2 8−12%) |
36852 35540 |
2.2
Wear evaluation
All 24 specimens, eight of each of the three test materials, underwent 1,200,000 loading cycles in a mastication simulator (Chewing Simulator CS-4, SD Mechatronic, Feldkirchen-Westerham, Germany) with a load of 49 N, a descending speed of 30 mm/s, and a lateral movement of 0.3 mm down the plane of the cusp. The first contact point was chosen so that the complete gliding lateral movement took place at the plane of the cusp. However, the loading steatite ball had to be machined as indicated in Fig. 1 to prevent destructive contact with the opposing cusp. The loading frequency was 1.2 Hz. After 120,000, 240,000, 480,000, 960,000, and 1,200,000 cycles elastomeric impressions (Express 2 Ultra-Light Body Quick, 3M ESPE, Neuss, Germany) were made from each specimen and investigated with a laser scanning microscope (vk-x 100, Keyence, Osaka, Japan) by using a laser with a wavelength of 408 nm and ×10 magnification. The area of wear was marked, and the vertical and volume substance loss evaluated by using a software program (VK-H1XAD, Keyence) for each impression. After 120,000 and 1,200,000 loading cycles, SEM images of a typical impression for each material were made (XL30, Philips, Kassel, Germany) after sputtering a 10-nm gold layer on the impression (Leica EM QSG 100, Wetzlar, Germany). The images were made by using an acceleration voltage of 10 kV.
After mastication simulation, all steatite antagonists were removed from the mastication simulator, and the height of abrasion was determined by using a calibrated optical camera system and an imaging software program (Photoshop CS, Version 11.0, Adobe, San Jose, USA).
The clinical wear of materials is highly dependent on factors other than the material, e.g., the contact area [ ]. For that reason, no power analysis was done, as it is difficult to determine an adequate effect size that correctly takes the clinical relevance into account. For this reason, the number of specimens was chosen based on previous studies that investigated wear behavior with a number of specimens ranging from 5 to 10 per group [ , , , , , ]. The data were statistically evaluated using SPSS statistical program (Version 20, IBM, Armonk, NY, USA). The Shapiro–Wilk test showed that the wear data of the tested crowns and of the antagonist were not normally distributed. Therefore, the data were evaluated by using the Kruskal–Wallis test followed by a pairwise Mann–Whitney test and Bonferroni–Holm correction.
3
Results
Median, means, and standard deviations of the vertical substance loss of the crowns and the volume loss of the crowns and the steatite antagonists are given in Tables 2–4 , respectively. An overview of the progress of the substance loss is given in Fig. 2 (vertical substance loss) and 3 (volume substance loss). The volume loss of the PICN crowns occurred early in the mastication simulation (after 120,000 cycles) and was significantly smaller (p ≤ 0.05) than the volume loss of the lithium disilicate and the zirconia-reinforced lithium silicate crowns. However, The PICN crowns showed statistically significantly less vertical substance loss for all numbers of loading cycles than lithium disilicate and zirconia-reinforced lithium silicate crowns. After 1,200,000 cycles, the steatite antagonists showed significantly less volume loss (p ≤ 0.05) against the zirconia-reinforced lithium silicate crowns compared with the other tested materials.
Cycles | LDS | PICN | ZLS | |
---|---|---|---|---|
120,000 | Median | 0.119 C, α | 0.071 A, α | 0.107 B, α |
Mean | 0.123 | 0.072 | 0.107 | |
SD | 0.013 | 0.013 | 0.009 | |
240,000 | Median | 0.163 A, αβ | 0.121 A, αβ | 0.194 A, αβ |
Mean | 0.156 | 0.126 | 0.198 | |
SD | 0.022 | 0.045 | 0.032 | |
480,000 | Median | 0.256 A, αβγ | 0.201 A, αβγ | 0.233 A, αβγ |
Mean | 0.246 | 0.214 | 0.218 | |
SD | 0.045 | 0.072 | 0.052 | |
960,000 | Median | 0.318 A, βγ | 0.273 A, βγ | 0.307 A, βγ |
Mean | 0.375 | 0.291 | 0.352 | |
SD | 0.131 | 0.070 | 0.121 | |
1,200,000 | Median | 0.405 A, γ | 0.362 A, γ | 0.340 A, γ |
Mean | 0.396 | 0.400 | 0.357 | |
SD | 0.065 | 0.092 | 0.070 |
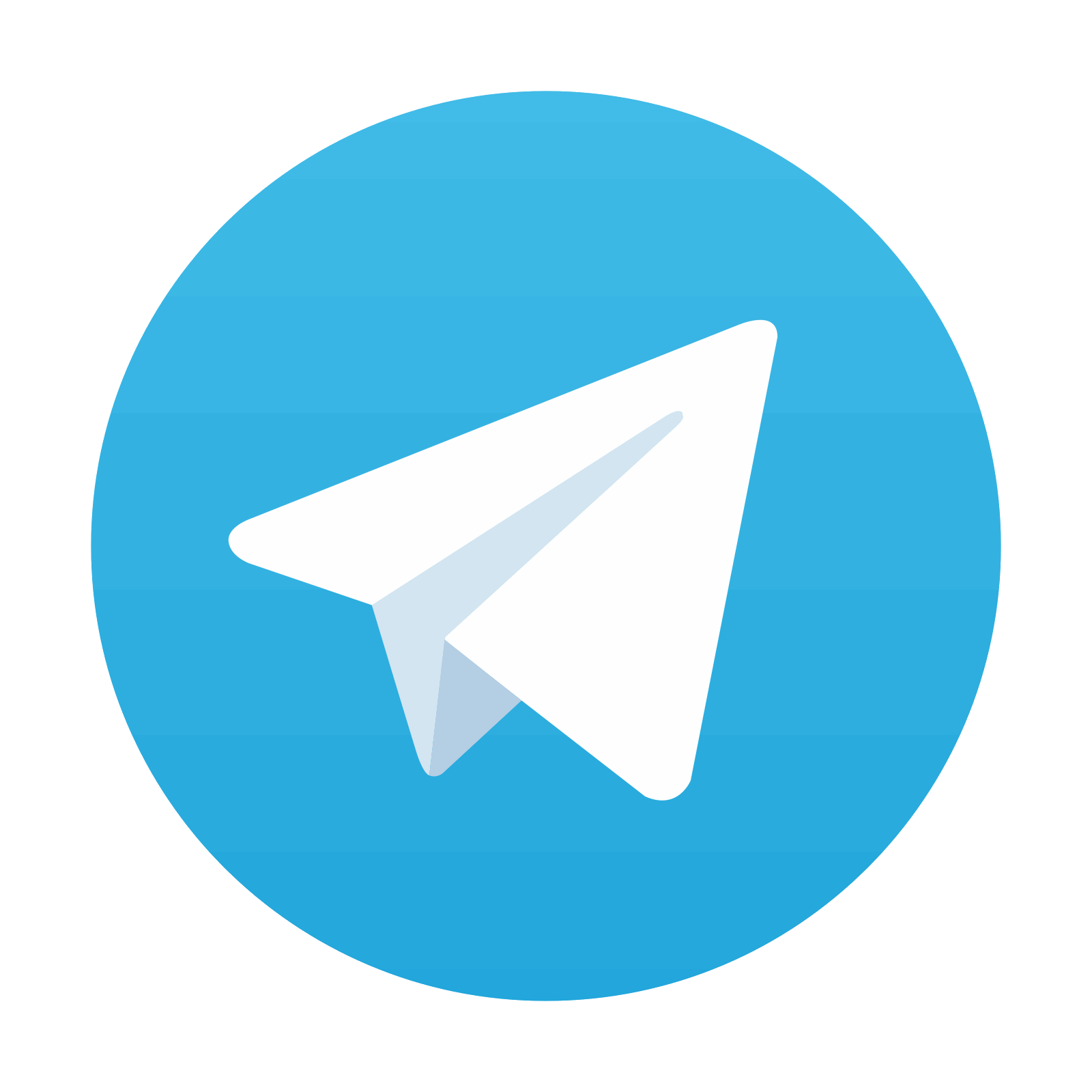
Stay updated, free dental videos. Join our Telegram channel

VIDEdental - Online dental courses
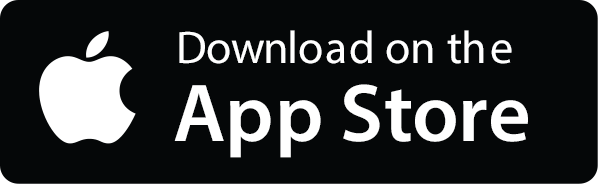
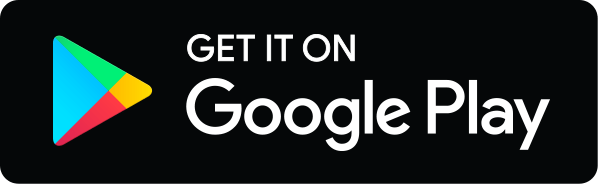
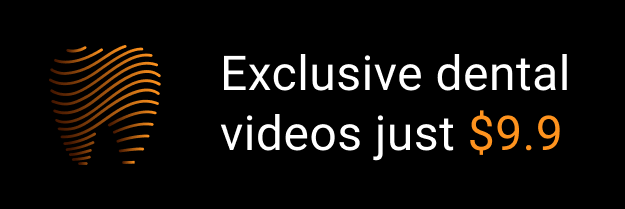