Graphical abstract

Abstract
Objective
The project aims to evaluate whether inhalation of particles released upon grinding of dental composites may pose a health hazard to dentists. The main objective of the study was to characterize the dust from polymer-based dental composites ground with different grain sized burs and investigate particle uptake and the potential cytotoxic effects in human bronchial cells.
Methods
Polymerized blocks of two dental composites, Filtek™ Z250 and Filtek™ Z500 from 3M™ ESPE, were ground with super coarse (black) and fine (red) burs inside a glass chamber.
Ultrafine airborne dust concentration and particle size distribution was measured real-time during grinding with a scanning mobility particle sizer (SMPS). Filter-collected airborne particles were characterized with dynamic light scattering (DLS) and scanning electron microscopy (SEM).
Human bronchial epithelial cells (HBEC-3KT) were exposed to the dusts in dose-effect experiments. Toxicity was measured with lactate dehydrogenase (LDH) assay and cell counting kit-8 (CCK8). Cellular uptake was observed with transmission electron microscopy (TEM).
Results
Airborne ultrafine particles showed that most particles were in the size range 15−35 nm (SMPS). SEM analysis proved that more than 80% of the particles have a minimum Feret diameter less than 1 μm. In solution (DLS), the particles have larger diameters and tend to agglomerate. Cell toxicity (LDH, CCK8) is shown after 48 h and 72 h exposure times and at the highest doses. TEM showed presence of the particles within the cell cytoplasm.
Significance
Prolonged and frequent exposure through inhalation may have negative health implications for dentists.
1
Introduction
Polymer based dental composites are now the most widely used material in restorative dentistry. They consist of inorganic filler particles, organic polymer matrix made of different methacrylate resins and a coupling agent binding the inorganic filler to the polymer matrix [ ]. In the drive to develop superior dental composite materials, the unique properties of nanomaterials is certainly attractive. Dental composite materials contain a mixture of micro- and nanosized particles [ ]. Nanoparticles are added mainly to increase filler load and thereby increase mechanical strength, diminish wear and reduce polymerization shrinkage of the composite [ ]. Nanoparticles can also provide desired aesthetic properties to the material [ ]. Hence, the use of nanoparticles in these materials have increased in the last decade. In addition to high levels of nanosized silica fillers, dental composites contain mixtures of other elements that increase radiopacity such as barium and aluminium [ , ].
As the use of dental composites in dentistry is increasing, there is a concern that dentists and other dental health workers may be exposed to nanoparticles released from materials, as well as nanoparticles generated during removal of fillings with burs and during polishing. The health risks associated with such exposure in the dental setting is not fully elucidated.
Studies have reported that particles of various sizes, including nanoparticles, are generated in high number concentrations, >10 6 cm 3 , during procedures such as high speed grinding of dental composites [ , ]. Whether nanofillers are released upon the grinding of dental composites is, however, still unclear [ ].
Van Landuyt et al. [ , ] identified the release of single nanofiller particles, while Bogdan et al. [ ] mostly observed organic nanoparticles originating from thermal decomposition of the resin matrix. In a study by Bradna Pavel et al., the bur coarseness and the bur speed was varied. No increase in particle concentration above background levels were observed when a fine diamond bur was used at 15 000 rpm compared to coarser burs used at higher speed (100 000 rpm) [ ].
Knowledge regarding indoor air quality in dental clinics from a chemical perspective are still scares. It has been shown that dental drilling procedures may cause an increase in particle concentrations in the dental office, and that the concentration was highest for particles under 0.5 μm [ ]. A study from Helmis et al. performed in several university clinics found that on most days, the daily concentration values of particulate matter <10 μm (PM10) exceeded the limit of 50 μg m −3 described by the Directive 1999/30/EC [ ]. Factors that influence the particle concentrations during different clinical procedures are the use of water spray and ventilation in the room [ ].
Dental health workers can potentially be exposed to hazardous dust or particles [ ] and methacrylate resin vapour [ , ] where inhalation and skin exposure to some of these materials might increase the risk of work-related illness and occupational diseases [ ]. An extensive literature review on occupational health problems in modern dentistry from Australia, suggests that a variety of health hazards as percutaneous exposure incidents (PEI), exposure to infectious agents, including bioareosol, respiratory hypersensivity, are found in dental practises [ ].
The characteristics of particles including size and chemical composition may affect their toxicity. It is known that the smallest particles ( i.e. nanoparticles) are accumulated in deeper parts of the respiratory system. Depending on particle size, most of the nanoparticles (<100 nm) are found in the alveolar region of the lungs [ ]. Once in the alveolar region they may cause local pulmonary inflammatory reactions or translocate into circulation [ ]. An in vitro study from Ansteinsson et al., showed that commonly used inorganic filler particles in dental composites released during dental restoration, could potentially trigger and maintain inflammatory reactions mediated by pro-inflammatory interleukin IL-6 and chemoattractant IL-8 [ ].
The main objective of this study was to perform an in-depth characterization of the dust with regards to size distribution and morphology of particles generated from two polymerized composites upon grinding with burs with different grain sizes, and while keeping the speed of the bur constant. The collected particles was used further to investigate the particle uptake and the potential cytotoxic effects on human bronchial cells.
2
Materials and methods
2.1
Dental composites
Two commercially available and commonly used dental composites were used in this study and are described by the manufacturer as follows: Filtek™ Z250 and Filtek Z500™ by 3 M™ ESPE™. The Filtek™ Z250 is a microhybrid containing zirconia/silica fillers (0.01–3.5 μm diameter) loaded to 60 vol.%. Filtek™ Z250 restorative contains BIS-GMA, UDMA, and BIS-EMA resins. Filtek™ Z500 is a nanohybrid containing a combination of aggregated 5−20 nm zirconia/silica fillers (so-called nanoclusters with average diameter 0.6–1.4 μm) and non-aggregated 20 nm silica filler ( Fig. 1 ). The inorganic filler loading is about 78.5% by wt (59.5% by volume). Filtek™ Z500 restorative contains bis-GMA, UDMA, TEGDMA, and bis-EMA resins. More information on the dental material can be found in the technical profile of 3 M™ ESPE™ Filtek™ Z250 and Z500™ Universal Restorative materials (3 M ESPE Filtek™ Z250 and Z500 Universal restorative).

2.2
Dental composites dust preparation and collection
For each composite, specimens of 2 mm × 2 mm × 25 mm were prepared in molds of stainless steel and light cured for three times 20 s on two side using Bluephase 20i (Ivoclar Vivadent) with and irradiance claimed by the manufacturer of 1200 mW/cm 2 . The weight of each block was approximately 0.2 g and the blocks were weighed before and after grinding to determine the amount of material ground. The blocks were ground inside of a chamber with hands aperture with black (super coarse, grain size 181 μm) and red (fine grain size 40 μm) diamond burs (40 000 rounds per minute) to create four different dental dust samples: Filtek™ Z250 red and black, and Z500™ red and black, respectively. The dental dust was collected using a GSP conical inhalation sampler (GSA Messgerätebau GMbH, Ratingen, GermanyCSI) with a cone diameter of 10 mm connected to a sampling pump with airflow approximately of 10 l/min. The GSP sampler contained a cassette with a filter with pore size of 5 μm (Millipore). Approximately the same amount of composite was ground for each block. The collected dental particles came from three different sampling campaigns and were, eventually, mixed to one pooled sample which was further used in the toxicity experiments.
2.3
Scanning electron microscopy (SEM)
The size distribution of all three batches as well as the pooled sample was investigated by SEM-EDX. The sample procedure for the dry collected sample was as follows: a small part of collected sample was drawn through a nozzle and impacted on a polycarbonate (PC) filter with pore size 0.2 μm in an antistatic sampler. The filter was precoated with approximately 10 nm platinum (Pt) in a Cressington 208 h sputter coater (Cressington Scientific Instruments Ltd., Watford, United Kingdom. Approximately 1 cm 2 piece of the filter was cut out, fixed on a 10 mm aluminium stub covered with double-sided carbon adhesive. Spots of carbon cement (Leit-C) were added to the sides of the stub to assure a good conductivity between filter and stub. In addition, the pooled sample was suspended in dispersion medium and sonicated (same as for DLS procedure). For the suspended particles, the dental composite particles were filtered through a PC filter with pore size 15 nm. A piece of approximately 8 × 8 mm piece of the filter was cut out, fixed on a 10 mm aluminium stub covered with double-sided carbon adhesive discs and spots of carbon cement (Leit-C, Agar Scientific Ltd.) were added to the sides of the stub to assure a good conductivity between filter and stub. The samples were coated with a 10 nm Pt layer. The specimens were analysed with a field emission scanning electron microscope SU6600 FESEM (Hitachi, Tokyo, Japan) operated with an acceleration voltage of 15 keV, analytical working distance of 10 mm, electron probe current 7−8 nA and backscatter imaging (BEI) mode using a solid-state detector. Automated analysis on the shape and size of particles was performed using the feature module of the esprit software (Bruker-AXS Microanalysis GmbH, Berlin, Germany). As a first step in the automatic particle analysis, high contrast (BE) images were acquired from the SEM at a magnification of 2000× at a resolution of 1800 × 1350 pixels (pixel size = 0.03 μm). The size of at least 2000 particles from each sample was obtained from the automatic analysis. The minimum Feret distance is equal to the smallest distance between two parallel tangents to the particle border and was chosen to represent the particle size ( Fig. 2 ).

2.4
Dust dispersion protocol and measurement by dynamic light scattering (DLS)
The pooled dental dust sample was used for the cell exposures after sonication. Filtek™ Z250 red and black and Filtek™ Z500 red and black were suspended in a dispersion medium (0.05%BSA and MilliQ water) to a final concentration of 2.56 mg/ml. Dispersion methodology and stock concentration were chosen in accordance with the Nanogenotox protocols [ ]. The solution with particles was sonicated using a 400 W Branson Sonifier S-450D (Branson Ultrasonis Corp., Danbury, CT, USA) equipped with a standard 13 mm disruptor horn (Model number: 101-147-037). Sonication was run for 15 min at 400 W and 10% amplitude. After sonication all samples were analysed with DLS and the hydrodynamic diameter measurements were conducted in dispersion medium. The measurements were done by a ZetaSizer (ZetaSizer Nano ZS, Malvern Instruments Ltd., Malvern, UK) using refractive index for a “composite” of 1.59. The analysis was conducted on three independent dispersed batches of pooled dental dust samples. Z-Average (Z-Ave) and polydispersity index (PdI) was measured. Z-Average gives the mean value of the hydrodynamic diameter of the particle and PdI measures the width of the particle size distribution.
2.5
Scanning mobility particle sizer (SMPS) measurements
Particle number concentrations were measured inside of the chamber during grinding using an SMPS (model 3938, TSI Inc., Shoreview, MN, USA). The SMPS consisted of the following TSI components: 3082 Electrostatic Classifier, 3756 Ultrafine Condensation Particle Counter (CPC, a long differential mobility analyzer (3081 DMA) and a 0.0457 cm diameter orifice aerosol inlet impactor. With the SMPS-parameters used in this study, particles in the range of 9−160 nm electrical mobility diameter were measured during grinding. A scan time of 30 s was used and was started simultaneously with the grinding. An antistatic tube of length 20 cm was connected to the impactor inlet of the Electrostatic Classifier and fixed inside the chamber. The tube creates a delay in the measurements and two scans passed before higher concentrations of nanoparticles were measured. Background levels were reached before starting a new grinding procedure. Two measurements were performed for each material and bur.
2.6
Exposure of human bronchial epithelial cells (HBEC-3KT), cell culture conditions and exposure doses
Human bronchial epithelial cell line HBEC-3KT, immortalised with CDK4 and hTERT, were used in all experiments. HBCE-3KT were maintained under standard growth conditions of 5 % of CO 2 and 37 °C [ , ]. Cell medium was a 1:1 mixture of LCH-9 (Gibco) and RPMI (Fisher) medium. Plates were pre-coated with 0.01 % collagen (Type I, PureCol® from Advanced BioMatrix). For each assay, one day prior to the experiment, HBEC-3KT cells were seeded at different densities depending on the length of exposure time and the growth area of the plate used: 2.5 × 10 4 cells/cm 2 for 24 h, 1.25 × 10 4 cells/cm 2 for 48 h and at 8.3 × 10 3 cells/cm 2 for 72 h. The exposure took place the following day. At the end of each exposure, the confluency of the cells was comparable. HBEC-3KT cells were exposed to Filtek™ Z250 (red and black) and Filtek™ Z500 (red and black) in the following concentrations; 0, 5, 10, 20, 40 and 80 μg/cm 2 . The time of exposure was 24 h, 48 h and 72 h. Doses and time points were chosen because of their relevance for occupational exposure situations. Number of biological replicates for each condition inside of the experiment is three and each experiment is repeated three times.
2.7
Cellular toxicity of filler materials
After each time of exposure, HBEC-3KT cells were tested for cell viability and cell toxicity using a cell counting kit-8 (CCK8) (Sigma-Aldrich) and lactate dehydrogenase (LDH) cytotoxicity assay (LDH–kit) (CyQUANT™, TermoFisher). CCK-8 and LDH assays are colorimetric tests based on two different metabolic reactions. They are measuring the amount of activity of living cells dehydrogenase (CCK8) and the activity of LDH dehydrogenase typical of damaged cells. The colorimetric assays are based on the conversion of the tetrazolium salt (INT) dye into a red formazan by electrons reduction, which can be quantified spectrophotometrically. The two assays combined give us more information on cell integrity and toxicity. Additionally, the convenience is that it is possible to use the same sample for both of the assays.
CCK8 absorbance was measured at 450 nm and this value is directly proportional to the number of living cells. LDH absorbance was measured at 490 nm and released values in percentage were obtained using the following formula:
%LDH releasedcytotoxicity=Compound-treated LDH activity−Spontaneous LDH activityMaximum LDH activity−Spontaneous LDH activity×100
The background signal was measured at 680 nm and subtracted from the 490 nm absorbance value. The absorbance were measured using a Spectramax-i3 and a Soft Max®Pro microplate data acquisition and analysis software (Molecular Devices).
2.8
Cellular uptake experiments with transmission electron microscopy
HBEC-3KT cells were exposed to the particles for 24 h and 72 h. At day one, the cells are exposed to Filtek™ Z500 black dental composites at the concentration of: 5 μg/cm 2 and 40 μg/cm 2 . After the exposure, the cells were fixed with a solution of 2,5% Glutaraldehyde (Fluka) in phosphate buffer saline (PBS) solution. The cells were washed three times in PBS and post-fixed for 20 min with a solution of 1%OsO 4 (Fluka). The cells were then gentle scraped and centrifuged for 5 min at the 13 000 rpm. The temperature was kept constant to not disturb the cells morphology. Porcine skin A gelatine (Electron Microscope Science™) was prepared and cool down at 37 °C and add to the cell pellet to create gelatine blocks. The gelatine blocks were embedded in durcupan mix (ACM-Fluka) and processed for the electron microscopy analysis.
Ultraphin sections were cut at 70−80 nm using a Leica Reichert Ultracut Ultratome and Diatome knife. Sections were contrasted with 2% uranyl acetate for 4 min and rinsed in MillQ water, contrast in lead citrate was avoid to not create deposition and interference during the observation. Sections were examined with a Tecnai-12 transmission electron microscope at 60 kV.
2.9
Statistical methods
SEM analysis is Kernel density non parametric distribution of minimum Feret diameter. DLS and CCK8 analysis are multiple comparisons.
To understand the changes in LDH released at different doses and to measure the amount of variability in LDH released between wells, we fitted linear mixed effects models with random effects at the well level. In particular, we considered two-way interaction terms involving time (24 h, 48 h and 72 h) and dose amounts. This helped in explaining the changes in LDH release within each dose amount from one time point to the other. Furthermore, we were able to present and explain the differences in LDH released between dose amounts at each time point using the time–dose interaction term. We also used margin plots to aid in understanding and interpreting of the interaction terms. However, the analyses showed no significant variability in LDH released between wells. All analyses were performed in StataSE 16 and the significance level was set at α = 0.05.
3
Results
3.1
Size distribution of particles generated during grinding measured with SMPS
The size distribution and number concentration of particles in the range 9−160 nm, measured inside the chamber during grinding of the different composite materials with burs with different grain size, showed air values in the range of 0.5 × 10 5 –1.5 × 10 5 cm −3 ( Fig. 3 A– D). The majority of measured particles were in the size range 15−30 nm and no significant differences between the materials were observed. As the grinding is made manually, curves are expected to fluctuate. However, the curves are still observed to have a lognormal distribution ( Fig. 3 ).
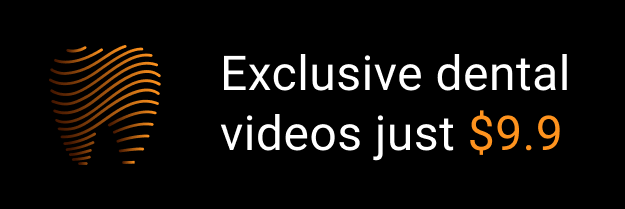