Abstract
Objective
The aim of the study was to investigate the effects of a novel polymerizable collagen cross-linker methacrylate-functionalized proanthocyanidins (MAPA) on the polymerization, microhardness and leaching of a HEMA-based experimental dental adhesive system.
Methods
Three MAPAs were synthesized using different methacrylate (MA) to proanthocyanidins (PA) feeding ratios of 1:2, 1:1, and 2:1 to obtain MAPA-1, MAPA-2, and MAPA-3, respectively. The resulting three MAPAs and PA were added to an experimental adhesive formulated with HEMA and a tri-component photoinitiator system (0.5 wt% CQ/EDMAB/DPIHP) at 1%, 5% and 10% MAPA or PA concentrations (wt%). The adhesive polymerization kinetics was measured continuously in real-time for 10 min using a Fourier-transform infrared spectroscopy (FTIR) with an attenuated total reflectance (ATR) accessory. Degree of conversion (DC) and Vickers microhardness (MH) of cured adhesives were measured at 72 h post-cure. The leaching of cured adhesives in DI water was monitored using UV–vis spectrophotometer. Statistical analysis was performed using one-way and two-way ANOVA, Tukey’s (p < 0.05).
Results
The adhesive formulations with 1%, 5% and 10% MAPAs-1, -2, -3 all generated higher rate of polymerization and 10-min DC than the formulations with PA at the same concentrations. At 72 h post-cure, the adhesive formulation with 5% MAPA-2 exhibited significantly higher DC (99.40%) and more than doubled MH (18.93) values than the formulation with 5% PA (DC = 89.47%, MH = 8.41) and the control (DC = 95.46%, MH = 9.33). Moreover, the cured adhesive with 5% MAPA-2 demonstrated significantly reduced PA leaching in comparison with cured adhesive with 5% PA.
Significance
Synthesized MAPA is a novel class of polymerizable collagen cross-linker that not only stabilizes dentin collagen via its PA component, but also improves polymerization, mechanical properties and stability of HEMA-based adhesives via its MA component. By inheriting the benefit while overcoming the drawback of PA, MAPA offers a revolutionary solution for improved bond-strength and longevity of dental restorations.
1
Introduction
Dental adhesion in modern restorative dentistry is achieved through the dentin-adhesive hybrid layer, in which adhesive resin penetrates into the demineralized dentin surface and forms micro-interlocked entanglement with exposed collagen fibrils [ ]. One of the leading causes for the failure of restorations is the lack of integrity in the dentin-adhesive hybrid layer, mainly due to incomplete resin infiltration and degradation of exposed demineralized dentin collagen, which is further aggravated by etching acid activated endogenous matrix metalloproteinases (MMPs) and cysteine cathepsins [ ].
Collagen crosslinking has been shown as an effective way to stabilize the hybrid layer [ ]. A group of naturally occurring polyphenolic compounds called proanthocyanidins (PA) is of special interest due to its dual-functionality as a cross-linker and MMP inhibitor as well as its lack of toxicity [ ]. Grape seed extract (GSE) is one of the most abundant sources of PA and has been widely studied for its applications in adhesive dentistry. Dentin collagen treated with GSE-PA has exhibited increased ultimate tensile strength, elastic modulus, bond strength and improved resistance to collagenase digestion [ ]. It was further revealed that the collagen stabilization effect of GSE-PA could be achieved in treatment time of less than 30 s, making it clinically feasible [ ]. These evidences render GSE-PA a promising agent for improving the durability of adhesive bonding in modern dental restorations. For the rest of the article, GSE-PA is denoted as PA unless otherwise stated.
Generally, there are three ways to integrate PA into an adhesive system: PA-containing primers, PA-containing etchants, and PA-containing adhesives [ ]. The use of PA as a primer prior to adhesion has been shown to protect exposed collagen fibrils in the hybrid layer from biodegradation, improve bond strength and decrease interfacial permeability [ , , ]. However, this approach adds an extra step to the procedure, which is not preferred in clinical practice. Alternatively, PA can be added to the etchant of an etch-and-rinse adhesive system. Several studies found that the incorporation of 2% PA to phosphoric acid of <20% concentration protected dentin collagen from enzymatic degradation, produced higher resin-dentin bond strength and less nanoleakage [ ]. However, this approach does not work for higher acid concentrations (20% and above) due to the breakdown of B-type interflavane links of PA in more acidic solutions [ ]. Another drawback of this approach is the poor solubility of PA in acidic solutions, which requires additional solvents such as ethanol or acetone in the formulation and thus limits the shelf life of the etchant.
Another convenient way is to add PA to dentin adhesives. Compared to the other two approaches, one advantage of this approach is the presence of PA in the hybrid layer for an extended period. Research evidence suggested that the presence of 5% PA in dental adhesives inhibited the biodegradation of unprotected collagen fibrils within the hybrid layer when bonded to the acid etched dentin [ ]. However, PA is a known radical scavenger and can interfere with adhesive monomer polymerization that relies on the generation and propagation of radical species to make the resin set [ ]. Experimentally, PA has been shown to hamper the photopolymerization and reduce the flexural strength, modulus of elasticity and microhardness of dental adhesives [ , ]. A recent clinical evaluation of PA added to a two-step etch-and-rinse adhesive showed that incorporating 2% and 5% PA to the adhesive impaired the longevity of composite restorations after 24 months [ ]. The dentin-adhesive bond strength is determined by the integrity of both the dentin collagen and the adhesive. Therefore, the use of PA together with adhesives faces an obvious dilemma and leads to unsatisfactory long-term performance.
To address the dilemma, we developed methacrylate-functionalized PAs (MAPAs), which contain polymerizable methacryloxy (MA) component and collagen crosslinking PA component, as a novel class of polymerizable collagen cross-linker (PCC) to add to dental adhesives. MAPAs are expected to help with the adhesive polymerization via the co-polymerization function of MA while inheriting the collagen stabilization function of PA. Furthermore, the chemical bonding of MAPA to adhesive monomers via co-polymerization may potentially reduce its leaching from the cured adhesive polymer. Our previous and ongoing research has already shown effective collagen stabilization function of MAPA [ ] (Part I study). The current study (Part II study) aimed to evaluate the effects of MAPA on the polymerization and microhardness of an experimental adhesive. Meanwhile, the leaching of compounds from light-cured adhesives was examined. The null hypotheses were (1) MAPA have the same effect on adhesive polymerization as PA; (2) MAPA have the same influence on the microhardness of light-cured adhesive as PA; and (3) light-cured adhesive with MAPA has the same leaching profile as that with PA.
2
Materials & methods
2.1
General
All chemicals were purchased from Sigma-Aldrich (St. Louise, MO, USA) unless otherwise stated. MegaNatural Gold grape seed extract (contains >90% proanthocyanidin) was donated by the manufacturer (Polyphenolics, Madera, CA, USA).
2.2
Synthesis and characterization of MAPAs
Three MAPAs were synthesized using different MA to PA feeding ratios with MAPA-1, MAPA-2, and MAPA-3 having MA:PA mole ratios of 1:2, 1:1, and 2:1, respectively. The chemical synthesis was accomplished via the coupling between a commercial grape seed extract (GSE, containing >90% PA) and methacryloyl chloride. The actual MA:PA ratios in the resulting MAPAs were estimated using 1 H NMR spectra to be 0.6:1, 0.9:1 and 1.5:1 for MAPA-1, MAPA-2, MAPA-3, respectively. Detailed synthetic procedures and structural characterizations of the MAPA compounds were described in Part I study [ ]. Fig. 1 a shows the schematic diagram of a representative synthesized MAPA compound. The actual chemical structures are different for the three MAPA compounds, with MA to PA ratios characterized by the 1720/1605 peak ratio in Fig. 1 b.

2.3
Formulation of experimental adhesives
The experimental adhesive was formulated by adding 0.5 wt% of camphorquinone (CQ), ethyl 4-(dimethylamino)benzoate (EDMAB) and diphenyliodonium hexafluorophosphate (DPIHP) to the 2-hydroxyethylmethacrylate (HEMA) monomer (control group). MAPA-1, MAPA-2, MAPA-3 and PA powders were then added to the adhesive solution at concentrations of 1%, 5% and 10% (wt%) to form the test groups. All adhesive solutions were prepared in the dark at room temperature and stored in a refrigerator.
The experimental adhesive formulation in the current study consisted of HEMA only as its net resin instead of a blend of bisphenol A glycidyl dimethacrylate (Bis-GMA) and HEMA as commonly used in commercial adhesives for well-thought-out reasons. HEMA monomers contain one methacrylate group and polymerize at a relatively slower pace in a linear fashion while Bis-GMA monomers contain two methacrylate groups and polymerize much faster in a crosslinking fashion. The fast rate of polymerization and high crosslinking density of Bis-GMA may mask any potential effects of MAPA on polymerization. Therefore, Bis-GMA was purposely excluded from the current adhesive formulation to allow the observation of the potential effects of MAPA and to facilitate the study of basic mechanisms.
2.4
Polymerization kinetics study
The same FTIR spectrometer was used for the kinetics study. Polymerization kinetics was monitored real-time using the Spectrum TimeBase software at a resolution of 4 cm −1 in the wavenumber range between 700 and 4000 cm −1 . A small volume of adhesive was dropped onto the top plate of the ATR diamond crystal and was immediately covered with a transparent plastic cover slip (Fisher brand, Catalog # 12–547). The adhesive was light-cured with a LED light curing unit (3M ESPE Elipar S10, 1200 mW/cm 2 , Germany) for 100 s. Three samples were tested for each group (n = 3).
To determine the degree of conversion (DC), the commonly used aliphatic C C stretching band at 1637 cm −1 couldn’t be used for our formulations due to the interference of bands associated with the phenyl groups in PA and MAPAs ( Fig. 2 a). Fortunately, the C H bending band at 815 cm −1 was well separated and therefore used for DC calculation instead ( Fig. 2 b). The peak at 1716 cm −1 (C O stretching) was chosen as the internal standard for normalization. Two-point baseline and maximum band height ratio protocol were used to measure the absorption intensity. The DC% was calculated using Eq. (1) :
DC%=1-(815cm-1/1716cm-1)Peakheightcured(815cm-1/1716cm-1)Peakheightuncured×100
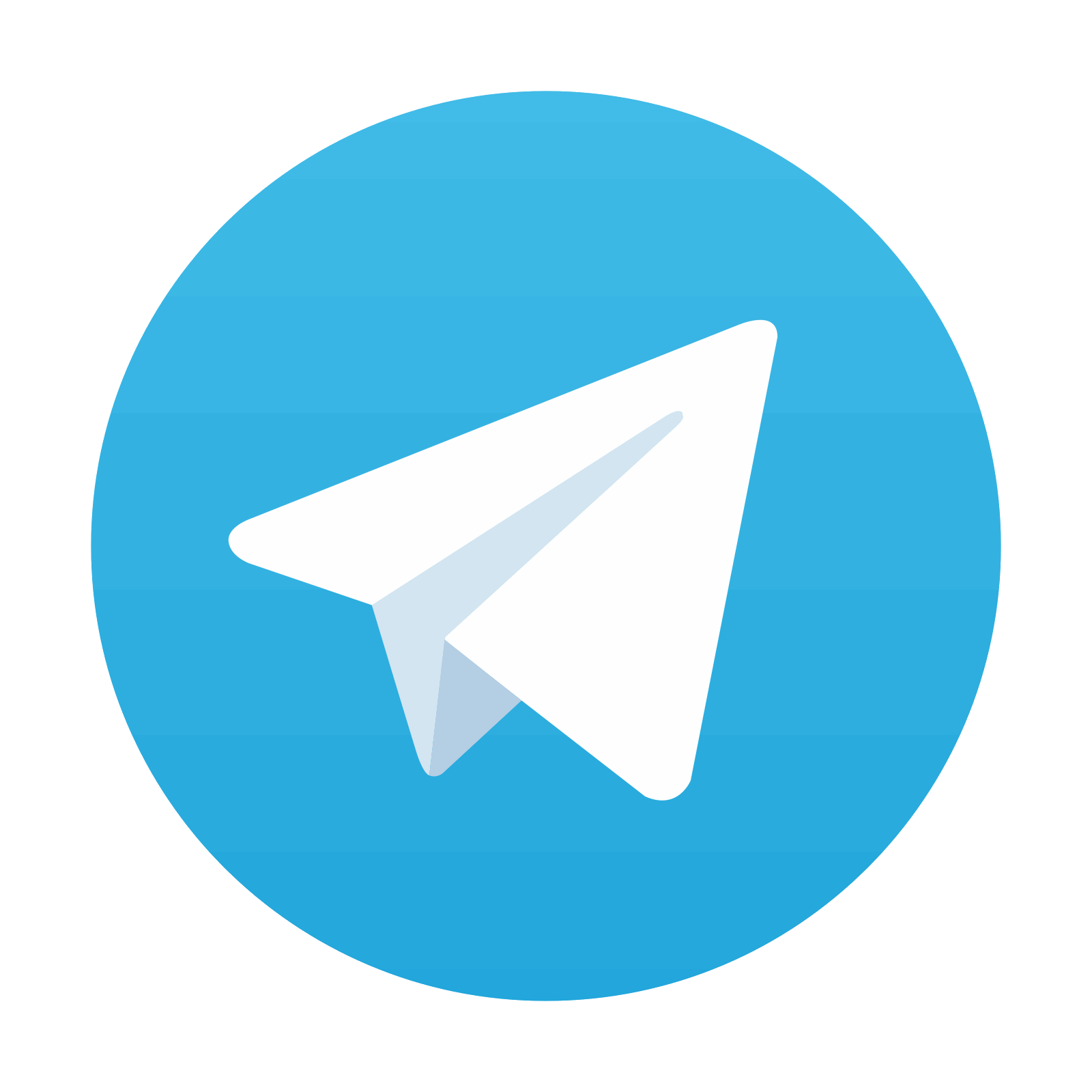
Stay updated, free dental videos. Join our Telegram channel

VIDEdental - Online dental courses
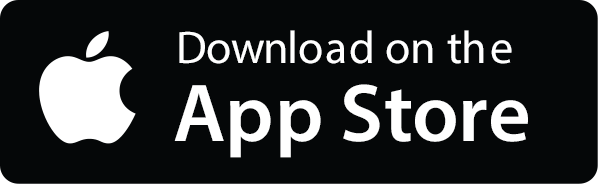
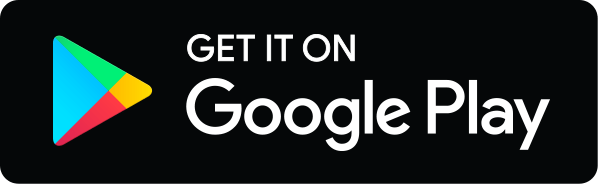
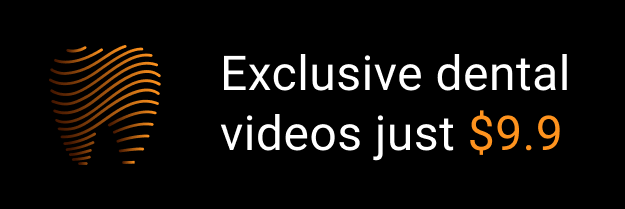