Highlights
- •
LTD develops in full zirconia prostheses after 6 months and increases with time.
- •
Glaze does not protect from LTD.
- •
LTD process is different in occlusal areas, where grain-pull out was observed.
- •
m phase quantification induces LTD underestimation in areas submitted to stress.
- •
LTD clinical impact is unknown and needs to be further studied.
Abstract
Objective
To investigate the intraoral development and kinetics of low-temperature degradation (LTD) in second-generation 3 mol.% yttria-doped tetragonal zirconia polycrystal (3Y-TZP) monolithic prostheses, as well as the influence of masticatory mechanical stress and glaze layer on it.
Methods
A total of 101 posterior tooth elements were included in a prospective clinical study, which included ex vivo LTD monitoring (at baseline, 6 months, 1 year, and 2 years) using Raman spectroscopy (n = 2640 monoclinic phase measurement points per evaluation time) and SEM. Four types of areas (1–2 mm 2 surface, 6 on molars, and 4 on premolars) were analyzed on each element surface: occlusal, axial, glazed, or unglazed. Raman depth mapping and high-resolution SEM were performed on the selected samples.
Results
LTD developed in 3Y-TZP monolithic restorations 6 months after intraoral placement and progressed with time. After two years, the tetragonal-to-monoclinic transformation was non-uniform, with the presence of localized clusters of transformed grains. In axial areas, the grain aspect was typical of the classical nucleation-growth process reported for LTD, which progresses from the surface to a depth of several tens of microns. However, in occlusal areas, tribological stress generated surface crushing and grain pull-out from the clusters, which induced an underestimation of the aging process when the evaluation was limited to monoclinic phase quantification. Glazing cannot be considered a protection against LTD.
Significance
If LTD occurs in dental prostheses in the same way as in orthopedic prostheses, its clinical impact is unknown and needs to be further studied.
1
Introduction
A low-temperature degradation (LTD) of a 3 mol.% yttria-doped tetragonal zirconia polycrystal (3Y-TZP) prosthesis was described for the first time in 1981 [ ]. This aging phenomenon is characterized by a slow surface transformation to the stable monoclinic phase in the presence of water within a certain temperature range typically from room temperature to approximately 400 °C. Transformation initially starts in the isolated grains on the surface by a corrosion stress mechanism and propagates internally in a slow nucleation and growth process [ ]. Nucleation on a particular grain at the surface in contact with water leads to a volume increase, which induces stress on neighboring grains and triggers their transformation and the formation of microcracks. This offers a path for water to penetrate by capillarity, allowing the process to also occur in the underlying layers [ ]. This phenomenon depends on severalfactors, such as the grain size, the amount and distribution of stabilizers, and the presence of manufacturing residual stresses [ ]. The LTD of 3Y-TZP ceramics used in orthopedic devices has catastrophic clinical consequences. In the early 2000s, fractures of approximately 600–800 zirconia heads within two years after implantation were reported. Seven batches of the Prozyr® material, manufactured with tunnel furnaces and expected to have increased sensitivity to LTD, were used [ ]. Several studies on LTD have been conducted at the laboratory [ ] and a few in vivo studies on aging, using retrospective explant analyses, have confirmed some of the features observed at the laboratory and showed surface degradation of retrieved zirconia hip implants [ , ] and/or related strong osteolysis [ ]. LTD accounted for some clinical failures related to the use of zirconia in orthopedics: the Prozyr® heads broke because of crack propagation from the micro-cracked transformed region loaded in tension, and some retrieved heads showed extensive grain pull-out, which led to material debris, chronic inflammation, and osteolysis in the worst cases [ ]. Those issues, especially the ‘Prozyr® failures,’ had a catastrophic impact on the use of Y-TZP in the field of orthopedics, in spite of the contrasting results for different zirconia heads, which were good and showed low wear rates [ , ].
Concomitant with its abandonment in orthopedics, the use of Y-TZP has increased in the dental field because of its better optical and biocompatibility properties when compared with metal alloys and its high mechanical resistance when compared with glass-ceramics. Surprisingly, 3Y-TZP is extremely popular and widely used in the field of dental prostheses [ ]; however, LTD has been sparsely investigated in vivo during clinical studies. The mechanisms of LTD have been relatively well-described, but there are still debates on the in vivo performance of Y-TZP in dental applications, particularly the 3Y-TZP second generation called the “translucent” zirconia [ ]. The increased translucency of these materials allows for the fabrication of high-strength monolithic crowns and fixed dental prostheses (FDPs), which are not needed in the posterior zone, to be covered by a feldspathic ceramic layer to obtain satisfactory esthetics. However, translucency is generally improved through a decrease in the content of alumina (a t-phase stabilizer) and/or an increase in the sintering temperature to reduce porosity and increase the grain size (2 h sintering at 1350 °C gives a 0.3 μm grain size, while it is higher than 2 μm at 1650 °C), which, in turn, increases the material metastability and LTD sensitivity [ , ] ( Table 1 ). Owing to the high variability of the materials tested, protocols employed, and the risks of bias of several studies [ ], the results for the influence of LTD on material strength are controversial; some studies have shown that LTD significantly decreases [ , ] or increases [ , ] the flexural strength of various Y-TZP dental ceramics, while others have reported that it does not have any influence [ ]. However, according to a meta-analysis of in vitro studies published in 2015, LTD induced by autoclave aging (aging duration longer than 20 h, pressure greater than or equal to 2 bars, and temperature of 134 °C) significantly increases the m-phase content and decreases the flexural strength of first- and second-generation 3Y-TZP dental ceramics [ ]. Some studies reported that LTD was responsible for a significant decrease in flexural strength when 50% of the sample surface was transformed [ ] and recent international standard rules for zirconia [ ] (ISO 13356- 2015) state that the monoclinic phase content should not exceed a maximum of 25% for Y-TZP implants to be considered suitable for biomedical purposes after aging in an autoclave at 134 °C and 2 bars for 5 h. However, in vitro autoclave aging conditions are different from the body environment [ ]. Moreover, the exposure to saliva (an ionic solution), acidic beverages, and constant changes in pH have not been taken into account [ , ], and mechanical stress due to masticatory forces (notably tribological effects) has not been considered, which can accelerate Y-TZP degradation, as shown in vitro [ ] and in vivo for zirconia hip prostheses (explant analysis) [ , ]. Recently, Miragaya et al. [ ] and Schepke et al. [ ] reported in vivo data on the LTD of Y-TZP dental ceramics, but they were limited. In the first study, flat Y-TZP specimens were attached to personalized intraoral resin appliances and exposed to the oral cavity (but not to mechanical stress) for two months, whereas in the second study, the transgingival part of zirconia implant abutments was observed after one year of clinical function.
Zirconia | Composition | Properties | Indications |
---|---|---|---|
First-generation | 3Y-TZP (<15% cubic phase) | High opacity/refractive index | Framework for veneered restorations |
High strength & toughness | |||
1000−1500 MPa flexural strength (manufacturers’ data) | |||
Second-generation : Translucent | 3Y-TZP (<15% cubic phase) | ↗Translucency | Monolithic (or veneered) posterior restorations |
↘ Alumina | ↗LTD | ||
Sintering at higher temperature to reduce porosities | 900−1300 MPa flexural strength (manufacturers’ data) | ||
↗ Metastability | |||
Third-generation: High-translucent | 4Y-PSZ (>25% cubic phase) | ↗ Translucency | Monolithic (or veneered) single-unit restorations |
5Y-PSZ (>50% cubic phase) | ↘ LTD | Max 3 elements-bridges | |
↗ Grain size: 1.5 μm | ↘ Strength & toughness | ||
↘ Metastability | 400−1000 MPa flexural strength (manufacturers’ data) |
In conclusion, the rationale for the long-term follow-up on dental prostheses is represented by the following questions: Is there aging in current dental zirconia? Are masticatory forces/contact stresses important in the process? If there is an operant, is this a clinical issue? Therefore, an original clinical research protocol, which includes ex vivo monitoring, was designed to investigate the in vivo development and kinetics of LTD in second-generation zirconia dental prostheses and the influence of masticatory mechanical stress on this process over 5 years [ ]. The secondary objective includes the influence of glaze protection on this process. The present work reports the 2-year results of this 5-year prospective clinical study on intraoral LTD. Additionally, this project also analyzed the general clinical performance (notably evaluation using criteria of the World Dental Federation) and material wear of prostheses using 3D-profilometry: the 2-year results of these specific investigations were reported in a previous paper [ ].
2
Materials and methods
2.1
Study design
The protocol of this prospective clinical study was approved by the Ethics Committee of the University Hospital Center (CHU) of Liege and was registered in the ClinicalTrials.gov database (Identifier NCT02150226). The study design is illustrated in Fig. 1 . Written patient consent was obtained before inclusion. The patients were treated by four experienced operators in the Department of Fixed Prosthodontics, Institute of Dentistry, CHU of Liege, Belgium. The eligibility criteria included the need for a molar or premolar crown (maximum of 6 elements per patient). The restorations were realized either on the teeth or the implants, and FDPs were also included, provided they were on the implants and limited to three elements (maximum 2 FDPs per patient). The exclusion criteria were severe or acute periodontal or carious diseases and poor oral hygiene. Patients without antagonistic teeth or those with removable prostheses as antagonists were excluded. A total of 47 patients, with a mean age of 54.3 ± 15.3 years, were recruited (14 males, 33 females). A total of 75 restorations (101 tooth elements) were included. Sample descriptions of patients, restorations, and tooth elements are presented in Table 2 . The participants received no financial compensation, although the treatment and prostheses were free of charge. All of the patient-related data were recorded in a specifically designed online database, to facilitate easy access to clinical data, pictures, and data obtained from ex vivo analyses for consultation. The database is hosted on a university hospital-secured server.

Patients (n tot = 47) | % (n) |
---|---|
Sex | |
Female | 70.2 (33) |
Male | 29.8 (14) |
Restorations (n tot = 75) | % (n) |
---|---|
Crowns | |
Implant screw-retained crowns | 58.7 (44) |
Implant cemented crowns | 5.3 (4) |
Tooth cemented crowns | 17,3 (13) |
Bridges | |
Screw-retained bridges on two implants | 17.3 (13) |
3-element | 14.7 (11) |
2-element | 2.7 (2) |
3-element cemented bridge on two implants | 1.4 (1) |
Tooth elements (n tot = 101) | % (n) |
---|---|
Support | |
Tooth | 12.9 (13) |
Implant | 87.1 (88) |
Tooth type | |
Premolar | 38.6 (39) |
Molar | 61.4 (62) |
Analyzed areas (n tot = 528) | % (n) |
---|---|
Occlusal (n = 326) | |
GCC | 26.4 (86) |
UCC | 23.9 (78) |
GNCC | 29.1 (95) |
UNCC | 20.6 (67) |
Axial (n = 202) | |
Buccal (Glazed) | 50 (101) |
Lingual/Palatal (Unglazed) | 50 (101) |
Raman measurement points (MPs) (n tot = 2640) | % (n) |
---|---|
Occlusal (n = 1630) | |
GCC | 26.4 (430) |
UCC | 23.9 (390) |
GNCC | 29.1 (475) |
UNCC | 20.6 (335) |
Axial (n = 1010) | |
Buccal (Glazed) | 50 (505) |
Lingual/Palatal (Unglazed) | 50 (505) |
2.2
Clinical procedure and fabrication of the restorations
2.2.1
Tooth preparation and impression for tooth or implant-supported prostheses
All clinical and technical procedures were performed strictly according to the manufacturer’s recommendations. The teeth were prepared following standardized criteria (1.0–1.5 mm occlusal depth cut to achieve the appropriate occlusal anatomy, 1.0–1.5 mm functional cusp tip reduction, 0.5 mm gingival chamfer reduction, and a 6–8 degree taper to the axial walls). A double-mix impression was performed with high- and low-viscosity A-silicone impression materials (Aquasil Heavy/XLV, Dentsply De Trey, Konstanz, Germany). An open tray impression technique was performed using the same impression material used for implant restorations. Two implant systems (NobelReplace, Nobel Biocare, Gothenburg, Sweden; and Standard Implants, Straumann, Basel, Switzerland) were used in this clinical study. The shade was registered using the Vita Classic System (Vita Zahnfabrik, Bad Säckingen, Germany). Restorations of antagonistic teeth were replaced in the presence of a deficient marginal joint, decay, or inadequate morphology.
2.2.2
Provisional restorations
Before manufacturing the zirconia restorations, CAD-CAM composite provisional crowns (Lava Ultimate, 3M ESPE, Seefeld, Germany) or PMMA-provisional FDPs were made. After die scanning the restoration was designed using CAD/CAM software: Exocad (Darmstadt, Germany) or Dental Wings (Montreal, Canada) (DPI Lava milling center, Anderlecht, Belgium). Specific buccal and palatal undercuts were added to the crown design to facilitate cemented crown removal ( Fig. 2 a). The file was transferred to a milling machine for manufacturing (Lava CNC 500, Serial Number: 07019 (2009), 3M ESPE). The provisional restorations were adjusted in the mouth and used as a mock-up for the design of zirconia restorations. Particular attention was paid to the occlusal contact point adjustment to obtain at least one flat contact surface of a minimum of 1−2 mm 2 per cusp by grinding or adding a composite ( Fig. 2 a and b).

2.2.3
Zirconia prostheses
Provisional restorations were scanned for zirconia restoration fabrication (Lava Plus, 3M ESPE, Seefeld, Germany) with the same milling system. Lava Plus is a second-generation 3Y-TZP, with a higher translucency than Lava Frame Zirconia (first-generation) due to a lower alumina content of 0.1%, optimally distributed within the material (Lava Plus, technical product profile, 3M). Sintering was performed according to the manufacturer’s instructions at 1450 °C for 2 h. The zirconia restorations were polished with a sequence of three diamond-impregnated silicone discs (Edenta AG, Hauptstrasse, Switzerland) and a diamond polishing paste (Bredent, Senden, Germany). Implant-supported restorations were bonded onto a specific titanium abutment (1000er-Serie, Medentika, Hugelsheim, Germany) with a resin composite cement (RelyX Ultimate [3M, Seefeld, Germany] in the first part of the study or Multilink Hybrid Abutment [Ivoclar Vivadent, Schaan, Liechtenstein] in the second part) after sandblasting the abutment and the zirconia restoration with 50 μm alumina particles at 2 bar according to the manufacturers’ recommendations.
Zirconia restorations were tried-in, and, if needed, occlusal contact points were adjusted with a diamond bur (ISO 524 grit size) and polished using a sequence of three zirconia-dedicated silicon gums (Diasynt Plus/Diacera Zirconium, Eve Ernst Vetter, Pforzheim, Germany), following the manufacturer’s recommendations. The need for occlusal adjustments was encoded in an online database for each occlusal area.
For restorations on implants that had no undercuts on buccal and lingual/palatal surfaces, a small groove was designed with a diamond bur ( Fig. 3 a) as a landmark for ex vivo analyses of axial surfaces, which were the control areas (free of mechanical stress). For molars, two cusps were randomly selected to remain unglazed: one centric cusp (UCC) and one non-centric cusp (UNCC). The two other cusps were called “glazed centric cusp” (GCC) and “glazed non-centric cusp” (GNCC) ( Fig. 2 c and d). For premolars, one cusp was randomly selected to remain unglazed ( Fig. 3 a). The buccal surface was systematically glazed, and the lingual/palatal surface was left unglazed on a part above the undercut or groove ( Figs. 2 c and 3 a). The glaze (IPS empress stains and eMax Ceram glaze, Ivoclar Vivadent, Schaan, Liechtenstein) was sintered at 780 °C for 1 min. Definitive bonding (bond was eliminated during glaze firing) on the specific titanium abutment was performed following a previously described procedure. The glazed restorations were tried-in, and occlusal contact point areas (one per cusp, i.e. , four on molars and two on premolars), as well as lingual/palatal and buccal areas, were marked for ex vivo analyses and registered with a picture ( Figs. 2 f and 3 b).

2.2.4
Zirconia prostheses placement and removal for ex vivo analyses
Baseline ex vivo analyses of the zirconia restorations were performed before placement. Screw-retained restorations were torqued at 35 Ncm −1 ( Fig. 3 c and d). To remove the gold sputter coating, the restorations were cleaned first with an alcohol-soaked cotton ball, brushed with toothbrush and toothpaste, and finally immersed in an ultrasonic bath containing alcohol for 3 min. Cemented restorations were sealed with eugenol-free cement (RelyX Temp NE, 3 M ESPE) ( Fig. 2 g and h), and the teeth were disinfected with 2% chlorhexidine. After 6 months, the restorations were removed for ex vivo analyses. Provisional restorations replaced the zirconia restorations during the analyses. The zirconia restorations were placed again in the mouth of the patient, following the same procedure as for the first time. Evaluations were repeated after a one-year intraoral stay and after two years. The occlusal contact points were landmarked with bite registration paper before removing the prostheses and encircled with permanent ink ( Figs. 2 f and 3 b).
2.3
Ex vivo analyses
Ex vivo analyses included (1) phase transformation with Raman spectroscopy and (2) glaze wear using scanning electron microscopy (SEM). Sample descriptions of the ex vivo analyzed areas (occlusal or axial, glazed, or not glazed) (n tot = 528) are presented in Table 2 .
2.3.1
Raman spectroscopy
Five measurement points (MPs) were randomly chosen for each occlusal contact area and each axial area ( i.e. , 30 measurements for a molar and 20 measurements for a premolar). Consequently, the analyses were performed for 2640 MPs at each evaluation time (baseline, 6 months, 1 year, and 2 years). Sample descriptions of the MPs are presented in Table 2 . Raman spectra were recorded using a Raman spectrometer (Horiba Jobin Yvon, Kyoto, Japan). The excitation laser was provided by a HeNe laser (632 nm), with 1 mW power focused at the surface of the specimen, and the Raman spectra were acquired using a charge-coupled device detector (Horiba-Jobin Yvon, Kyoto, Japan) with a cm −1 spectral resolution of 1 (1800 grooves/mm grating). The Raman spectrometer was combined with an optical microscope (Olympus LX71; Olympus Corporation, Tokyo, Japan). A confocal pinhole with adjustable diameter was used for confocal detection, and an 80× objective (numerical aperture 0.75) was used to achieve a 1 μm 3 resolution (lateral × axial). A pinhole aperture of 1.99 μm was used to reach a collection depth of 1.44 μm. The depth was determined using the calibration-wedge method [ ].
The collected spectra were analyzed, and the transformed monolithic volume fraction (V fm ), which is a measurement of the transformation volume ratio in the confocally probed volume, was estimated using Eq. (1) , which was initially proposed by Clarke and Adar [ ]
Vfm=Im178+Im1890.33It145+It256+Im178+Im189
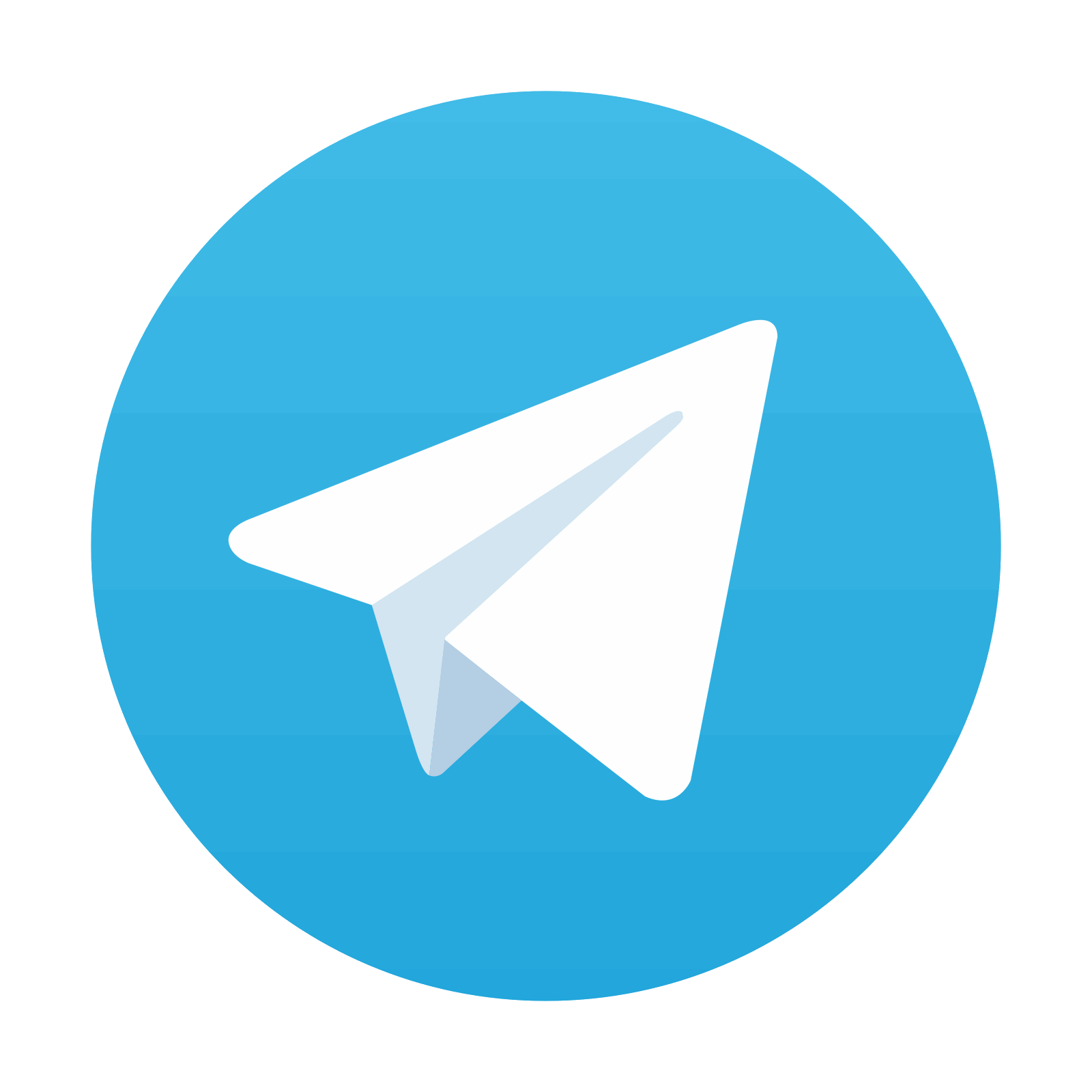
Stay updated, free dental videos. Join our Telegram channel

VIDEdental - Online dental courses
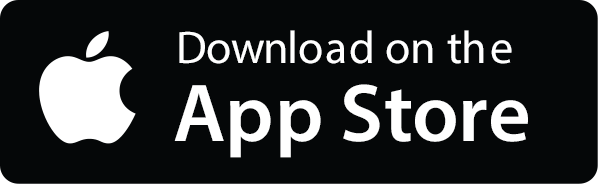
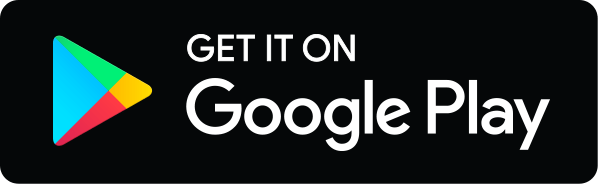
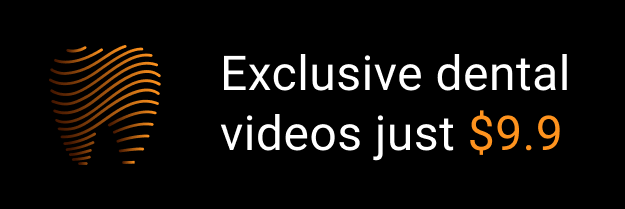