Direct Activation Pathways
-
Explain the difference between the direct and indirect pathways of motor control.
-
Discuss the differences and similarities between and UMN and LMN.
-
Discuss the motor somatotopy of the cortex and the spinal cord.
-
Identify the three major motor tracts of the direct pathway.
-
Describe the pathway of the corticospinal and corticobulbar tracts.
-
Discuss a lesion of the corticobulbar tract involving CN VII and CN XII.
-
List the clinical signs of upper motor neuron syndrome and lower motor neuron syndrome.
Overview of Direct Motor Pathways
Motor control is a complex system composed of several individual components that together produce movement. These include motor neurons, descending motor pathways and associated cortical areas, basal ganglia, and cerebellum. Pathways that are directly responsible for the voluntary activity of the muscles of the head, neck, and limbs are referred to as the direct activation pathway. The direct motor pathway is monosynaptic. Cortical upper motor neuron (UMN) project to and synapses on a lower motor neuron (LMN) located in the brainstem and spinal cord. Fibers from cortical neurons connect the UMN, LMN, and the skeletal muscle they innervate forming the descending pathways. The corticobulbar tract involves cortical UMNs that project to cranial nerve motor nuclei (LMN) in the brainstem. The corticospinal tract consists of UMN in the cortex projecting to the ventral gray matter of the spinal cord where the LMN cell bodies are located. The axons of LMNs synapse on skeletal muscle to initiate movement. Although not part of the direct motor pathways, the basal ganglia and cerebellum have profound regulatory influence on movement (see Chapter 17). Other descending pathways involved in movement but not originating in the cortex and involving multiple synapses are the rubrospinal tract, the tectospinal tract, reticulospinal tract, and the vestibulospinal tract (see Chapter 17). These four indirect pathways originate in brainstem nuclei. In addition to movement, motor pathways are important components of reflex pathways. Reflexes are involuntary (efferent) responses to sensory (afferent) input.
Motor Neurons
-
UMN (pyramidal cells) reside within the cerebral cortex. There are four specific cortical areas where the corticospinal and corticobulbar tracts originate ().
-
The precentral gyrus is somatotopically organized.
-
Stimulation of motor neurons in a specific area of the primary motor cortex results in the contraction of specific muscles. In other words, there is a direct relationship between areas of the motor cortex and regions of the body creating a cortical map, known as a homunculus ().
-
The proportions of the motor homunculus represent the relative number of motor units involved in control of that particular region. Thus, the homunculus indicates the amount of fine motor control in a given area. For example, the hands, face, and tongue are depicted as being quite large compared to the trunk.
-
-
UMN cells in the cortex project to LMN in the brainstem and spinal cord ().
-
The axons of the UMNs that innervate cranial nerve nuclei (LMN) exit the pathway at the appropriate level forming the corticobulbar tract.
-
The remainder of the axons continue through the pyramids. Most will cross or decussate forming the decussation of the pyramids. All will enter the spinal cord as the corticospinal tract.
-
-
Skeletal muscles receive innervation from a motor pool.
-
A motor pool consists of all a-motor neurons that innervate a single muscle.
-
Each a-motor neuron within a motor pool sends an efferent (motor) axon toward the periphery, which branches to form synapses called neuromuscular junctions (NMJs) on specific groups of individual muscle fibers.
-
Innervation is achieved by release of a neurotransmitter (acetylcholine) that is released from the axon of the LMN. Receptors present in the membrane of the plasma membrane of the muscle fiber bind the acetylcholine, which will facilitate the transmission of the impulse resulting in contraction of the muscle fiber.
-
-
The group of individual muscle fibers innervated by a single a-motor neuron and its efferent axon constitutes a motor unit. An action potential generated by a motor neuron results in the simultaneous contraction of all muscle fibers in the motor unit.
-
Any given muscle contains multiple types of motor units that are interspersed throughout different regions of the muscle and provides a selective level of motor unit activation and control. Difference in motor unit fiber distribution reflects difference in muscle function.
-
The relative proportion of a specific type of motor unit along with the innervation ratio (motor unit size), the motor unit distribution within a muscle, and the overall number of motor units found in a muscle varies between different muscles and reflects muscle function.
Fig. 16.1 Sensory and motor systems. The sensory system and motor system are so functionally interrelated they may be described as one (sensorimotor system). Cortical areas of the sensorimotor system. Lateral view of the left hemisphere. (Reproduced with permission from Gilroy AM, MacPherson BR, Ross LM. Atlas of Anatomy. Second Edition. © Thieme 2012. Illustrations by Markus Voll and Karl Wesker.)
Fig. 16.2 (a) Motor homunculus. (b) Sensory homunculus. The relationship between the motor cortex and the rest of the body can be illustrated by the homunculus. This cortical map represents the area of the brain that is responsible for motor function in the rest of the body, as well as the relative number of motor units as seen by the proportions of the structures.
slow oxidative, fatigue resistant |
Remain tonically active during movements requiring sustained activation |
||||||
Type II fibers; fast oxidative/glycolytic, fatigue resistant |
|||||||
Corticospinal Tract
-
Multiple cortical areas contribute to motor control of the head and the rest of the body.
-
The axons of the UMN in the cortex project to both the spinal cord and brainstem.
-
There are three major descending motor tracts that innervate skeletal muscles of the head and body ().
-
The axons of cortical UMNs exit the subcortical white matter and form the corona radiata ().
-
Thus, the internal capsule contains the same axons of the corona radiata ().
-
The motor fibers continue to descend through the brainstem (a). As they approach the crus cerebri (the anterior portion of the cerebral peduncle) (b), they reorganize such that the fibers associated with the head are more medial (corticobulbar) and those associated with the legs are more lateral (corticospinal). The fibers that will innervate the upper limbs are in between (corticospinal).
-
As the fibers approach the lower brainstem (medulla), the corticospinal and corticobulbar fibers form the medullary pyramids (a, b).
-
When the corticospinal fibers approach the spinal cord, most will cross in the midline to form the pyramidal decussation.
-
From the decussation, the corticospinal tract continues into the spinal cord (a, b).
-
Approximately 85% of the corticospinal fibers will cross as the lateral corticospinal tract. They descend in the lateral funiculus of the spinal cord and will ultimately innervate the more distal musculature.
-
The remaining 15% of corticospinal fibers that descend uncrossed are called the anterior (ventral) corticospinal tract. These fibers run in the anterior funiculus of the spinal cord and will innervate the more proximal musculature.
-
-
The majority of the corticospinal fibers will terminate on interneurons rather than directly on motor neurons in the ventral horn of the spinal cord ().
-
There are functional differences between the different components of corticospinal tract.
-
The lateral corticospinal tract innervates the cervical and lumbar part of the spinal cord and controls fine movements of the extremities.
-
The anterior corticospinal tract mediates postural mechanisms.
-
Some fibers from the corticospinal tract project to the dorsal horn in order to modulate sensory information that travels to the cortex.
-
Fig. 16.8 (a) Division of the brainstem into levels: Midsagittal section: The brainstem is divided macroscopically into three levels, with the bulge of the pons marking the boundary lines between the parts: (A) Mesencephalon (midbrain). (B) Pons. (C) Medulla oblongata. The three levels are easily distinguished from one another by gross visual inspection, although they are not differentiated in a functional sense. The functional organization of the brainstem is determined chiefly by the arrangement of the cranial nerve nuclei. Given the close proximity of nuclei and large fiber tracts in this region, even a small lesion of the brainstem (e.g., hemorrhage, tumor) may lead to extensive and complex alterations of sensorimotor function. (b) After exiting the internal capsule, the motor fibers descend through the brainstem. (Fig. 16.8a: Reproduced with permission from Baker EW. Anatomy for Dental Medicine. Second Edition. © Thieme 2015. Illustrations by Markus Voll and Karl Wesker. Fig. 16.8b: Reproduced with permission from Schuenke M, Schulte E, Schumacher U. THIEME Atlas of Anatomy Second Edition, Vol 3. ©Thieme 2016. Illustrations by Markus Voll and Karl Wesker.)
Fig. 16.9 (a) The descending motor fibers form the medullary pyramids at the lower end of the brainstem. (b) Most of the corticospinal fibers cross as they near the spinal cord forming the pyramidal decussation. Corticobulbar fibers exit the pyramids along the length of the medulla. (Fig. 16.9a: Reproduced with permission from Schuenke M, Schulte E, Schumacher U. THIEME Atlas of Anatomy Second Edition, Vol 3. ©Thieme 2016. Illustrations by Markus Voll and Karl Wesker. Fig. 16.9b: Modified with permission from Silbernagl S, Despopoulos A. Color Atlas of Physiology. 6th edition. © Thieme 2009.)
Fig. 16.10 (a) The corticospinal tract continues into the spinal cord. (b) The majority of the fibers will cross as the lateral corticospinal tract. They descend in the lateral funiculus and will innervate the more distal muscles. (a: Reproduced with permission from Schuenke M, Schulte E, Schumacher U. THIEME Atlas of Anatomy Second Edition, Vol 3. ©Thieme 2016. Illustrations by Markus Voll and Karl Wesker. b: Reproduced with permission from Gilroy AM, MacPherson BR, Ross LM. Atlas of Anatomy. Second Edition. © Thieme 2012. Illustrations by Markus Voll and Karl Wesker.)
Corticobulbar Tract
-
The corticobulbar tract originates from the lateral aspect of the primary motor cortex ().
-
Cortical neurons from the parietal area represent the UMNs that target cranial nerve motor nuclei, which are the LMNs.
-
The axons of the UMN travel through the corona radiata, internal capsule, crus cerebri of the midbrain, and the medullary pyramids in a similar trajectory to corticospinal fibers.
-
The UMN of the corticobulbar tract will synapse directly on motor neurons or indirectly on interneurons of the reticular formation.
-
This system is responsible for motor control of the muscles of facial expression, eye movements, movements of the tongue, and elevation and depression of the mandible.
-
One of the unique aspects of the corticobulbar system is how it innervates some of its targets.
Lesions of the Corticobulbar Tract
As previously indicated, most of the innervation of cranial nerve motor nuclei from the cortex is bilateral. Therefore, a lesion that occurs between the cortex and the cranial nerve nuclei will not result in paralysis of the muscles of the face and head.
-
In the case of the face (CN VII), the area above the eyes would only experience mild weakness, if anything, due to the bilateral innervation. However, the area below the eyes receives unilateral innervation, so marked weakness or paralysis would be evident. Furthermore, the input is contralateral so that muscles of facial expression below the eyes and protrusion of the tongue would be affected on the side contralateral to the lesion ().
-
Clinical symptoms of a CN XII lesion are also dependent on whether or not the lesion involves UMNs or LMNs (a, b). A supranuclear lesion (UMN) lesion of CN XII would result in deviation of the tongue on protrusion to the side opposite to the lesion.
-
CN XII innervates the genioglossus, which is responsible for protrusion of the tongue.
-
Knocking out the fibers (lesion) projecting to the motor nucleus of CN XII (UMN) will produce contralateral deficits. The tongue protrudes to the side opposite to the lesion because it is unopposed.
-
Knocking out CN XII at the level of the nucleus or distally (LMN) would result in ipsilateral deficits. In an LMN lesion, the tongue will deviate to the same side as the lesion because it is unopposed.
-
Fig. 16.13 (a) LMN lesion. (b) UMN lesion. Most of the cranial nerve nuclei are bilaterally innervated (right and left cortices) so a lesion occurring between the cortex and the nuclei (UMN) will not result in paralysis. One exception to that rule is CN VII. In the face, the area above the eyes is bilaterally innervated, so a lesion would result in mild weakness, if anything. However, the area below the eyes in unilaterally innervated, so the same lesion would cause marked weakness or paralysis. Additionally, the unilateral innervation is contralateral, so the effect would be seen on the side of the face opposite to the lesion (lesion right side = paralysis left side) LMN, lower motor neuron; UMN, upper motor neuron.
(b) A UMN lesion would result in contralateral deviation of the tongue (opposite the lesion) while an LMN lesion would result in ipsilateral deficits (same side as the lesion). LMN, lower motor neuron; UMN, upper motor neuron. (a: Reproduced with permission from Alberstone CD, Benzel EC, Najm IM, et al. Anatomic Basis of Neurologic Diagnosis. © Thieme 2009.)
Disorders of the Motor System
Damage or disease of the motor system can cause unique symptoms and challenges for patients depending on the type of motor neuron that is involved. Damage can involve the motor cell bodies or their axons. Both UMN and LMN damage produces characteristic symptoms that allow clinicians to determine the type of disorder the patient is experiencing (Clinical Correlation Box 16.1 and 16.2) ().
Brown Sequard syndrome is a spinal cord lesion that results from a hemisection injury to the spinal cord (). This results in interruption of the lateral corticospinal tract, lateral spinothalamic tract, and posterior column. Clinical presentation includes a spastic weak leg with intact reflexes and a strong leg with loss of pain and temperature. The motor fibers of the corticospinal tract cross at the decussation of the pyramids. Thus, damage to the corticospinal tract on one side produces ipsilateral weakness below the lesion with UMN and LMN signs. Common causes of spinal cord hemisection include trauma, herniated disks, and degenerative disease of the vertebral column.
Fig. 16.16 Brown Sequard syndrome is a condition that is caused by injury to the spinal cord resulting in a hemisection. The result is damage to the corticospinal tract, spinothalamic tract, and posterior column. Clinical presentation includes: affected (weak) leg with intact reflexes and unaffected (strong) leg with loss of pain and temperature. (Reproduced with permission from Alberstone CD, Benzel EC, Najm IM, et al. Anatomic Basis of Neurologic Diagnosis. © Thieme 2009.)
Myasthenia gravis (MG) is a chronic autoimmune disease that results in weakness of skeletal muscles. The hallmark characteristic of this disorder is muscle weakness that becomes more severe with increased activity and shows improvement with rest. MG pathology is due to antibody-mediated blocking of acetylcholine receptors at the neuromuscular junction. In these individuals, autoantibodies form against the postsynaptic receptors involved in neuromuscular transmission. The result is inefficient transmission of the impulse from the motor neuron to the muscle fiber. When this failure occurs in enough muscle fibers, it can manifest clinically. The ocular and bulbar muscles are most frequently involved and are often the most severe. One of the greatest concerns with this disease is if respiratory muscles become involved it can lead to respiratory failure. MG is a disorder that has been studied extensively and is clearly defined. It is also very well managed. Therapy includes anticholinesterase drugs as well as immunotherapy. Thymectomy is often performed if acetylcholine receptor antibodies are present.
Upper Motor Neuron Syndrome
Damage to UMNs is common because of the large surface area they occupy in the cortex and because the pathways run for significant distances, that is, cortex to lower end of the spinal cord. Lesions occurring anywhere along the pathway result in a disorder known as upper motor neuron syndrome.
-
Damage to the motor cortex or internal capsule results in immediate muscle flaccidity on the contralateral face and body.
-
The initial hypotonia is also known as spinal shock (Clinical correlation Box 16.3).
-
Trunk muscles may be functional due to bilateral innervation from the corticospinal pathway.
-
Classic patterns of UMN syndrome are as follows:
-
-
The normal response to stroking the sole of the foot is flexion of the great toe as well as the other toes. UMN damage results in the extension of the great toe and fanning of the other toes upon stroking the sole of the foot. A similar response is present in infants prior to the development of the corticospinal pathway. Children from birth to 2 years of age will display this plantar response due to the underdeveloped motor pathways.
-
-
Lower Motor Neuron Syndrome
Damage to the motor neurons of the spinal cord and brainstem are called lower motor neuron syndrome. Damage may occur to the cell bodies or their axons.
Spinal Reflexes
A spinal reflex involves efferent output in response to afferent input (). Reflexes range from simple such as the stretch reflex to more complicated activities like walking movements. Therefore, all reflexes involve a motor neuron and a sensory neuron and except for a few circumstances, interneurons as well.
-
The stretch reflex is a myotactic reflex. It is one of the simplest of reflexes involving two neurons and one synapse. The afferent limb of the reflex is an afferent fiber from a sensory neuron in the dorsal root ganglia. Its peripheral process is associated with a muscle spindle. The central process of the sensory neuron synapses on a motor neuron within the spinal cord. Activation of the motor neuron will cause the muscle to contract.
-
One of the major roles of the myotactic reflex is to maintain posture. If an individual is standing upright and loses balance, the muscles in the limbs are stretched, activating the reflex arc to counteract the swaying.
-
The myotactic reflex is a clinically important reflex as it is the basis for the knee-jerk reflex. By tapping the patellar tendon, the reflex movement of the extensor muscles of the thigh occurs, thereby extending the lower leg. This is also known as a deep tendon reflex and, if absent, can indicate an interruption in the corticospinal pathway.
-
Hyporeflexia is an absent or diminished reflex response and can indicate an issue with one of the components in the two-neuron reflex.
-
Hyperreflexia is an overreaction and repeated (clonic) response. It usually indicates a problem with the corticospinal tract or other descending pathways that influences the reflex arc.
-
-
-
The flexor reflex is initiated by a cutaneous receptor and involves the entire limb. This reflex is also called the withdrawal reflex, which is illustrated by a painful stimulus and a subsequent withdrawal from that stimulus by flexion.
-
Crossed extension reflex is a compensatory reflex that results in support of the body during a flexion reflex. In other words, activation of the flexion reflex on one side of the body which results in flexion of a group of muscles in one limb is always accompanied by contraction of the extensors of the limb on the other side of the body.
-
This activity provides support for the body and prevents a loss of balance.
-
Afferents activated from the flexion reflex innervate an interneuron that sends its axon across the midline to the contralateral spinal cord. Here, it excites a motor neuron that innervates extensor muscles of the opposite leg. The extensors act as contralateral antagonists.
-
This crossed effect allows balance and body posture to be maintained.
-
-
Autogenic reflex/Golgi tendon reflex is thought to have a protective function by regulating motor unit activity. It most likely also plays an important role in normal function by spreading work more evenly across the entire muscle, thus making motor units more efficient.
-
The Golgi tendon organ (GTO) is located at the junction of the tendon and the muscle.
-
When tension is applied to a muscle, afferents innervating the GTO are activated.
-
The central process of the afferent neuron synapses on an interneuron that then makes an inhibitory synapse on the motor neuron that innervates the same muscle that is sending the afferent signal.
-
This reflex results in a decrease or cessation of the muscle contraction.
-
Spinal shock refers to the pathophysiologic condition that occurs following a transection of the spinal cord resulting in temporary loss or diminished spinal reflex activity below the level of injury. Spinal shock is commonly associated with trauma and is typically immediate. In some instances, loss of reflex activity may be seen above the lesion. The period of spinal shock varies from days to weeks. The end of the phase is marked by abnormal corticospinal reflexes such as Babinski’s sign or exaggerated muscle spindle reflex arcs. Reflexes may never return in areas that are severely damaged by the trauma.
Questions and Answers
-
Which of the following structures contributes proprioceptive information to the corticospinal tract?
Answer B: The postcentral gyrus is also called the primary somatosensory cortex. It provided proprioceptive information via the dorsal column-medial lemniscus pathway; (A) precentral gyrus is the primary motor cortex; (C) the precentral gyrus, (D) the supplemental motor area and the premotor cortex are all found in the frontal lobe. None of these areas provide sensory information.
-
In terms of the motor homunculus, which of the following areas represent the origin of the corticobulbar tract?
Answer B: Frontal lobe; (A) Parietal, (C) Temporal and (D) Ooccipital lobes are not involved in the motor activity.
Answer C: Lateral corticospinal tract; (A) Fibers from Corticobulbar tract exit the pyramids to innervate the head; (B) Ventral corticospinal tract descends into the spinal cord uncrossed; (D) Anterior funiculus is made up of descending motor fibers from the ventral corticospinal tract within the spinal cord.
-
Patient A presents with a lesion of the right lateral corticospinal tract at L2. Which of the following signs would be present?
Answer D: Ipsilateral hypertonia. Lesions of the corticospinal tract results in ipsilateral UMN symptoms, which includes hypertonia; (A) Clonus would be positive ipsilaterally; (B) Ipsilateral positive Babinski sign would be present; (C) Spastic paralysis would be present but it would be ipsilateral.
-
Patient B suffered a stroke. Among other issues, when asked to protrude her tongue, it deviates to the right side. There is no muscle atrophy. Fasciculations are absent. What type of lesion does Patient B suffer from?
Answer B: UMN left side. Lack of muscle atrophy and fasciculations with deviation of the tongue indicates UMN damage. Symptoms in an UMN syndrome are contralateral to the lesion. Patients’ tongue deviates to the right so lesion occurred on the left side; (A) would be ipsilateral; (C) and (D) answers are incorrect because symptoms indicate and UMN lesion.
Indirect Activation Pathways
-
Describe the indirect motor pathway. How does it differ from the direct pathway?
-
What is the rubrospinal tract? Describe its function and pathway.
-
What is the reticulospinal tract? Describe its function and pathway.
-
What is the vestibulospinal tract? Describe its function and pathway.
-
What is the tectospinal tract? Describe its function and pathway.
-
What is the function of the basal ganglia? What are the inputs and outputs?
-
List the symptoms commonly seen in a basal ganglia disorder.
-
What are the typical symptoms seen in individuals with a cerebellar lesion?
Overview of Indirect Influences on Movement
Historically, the corticospinal and corticobulbar tracts were considered to be part of the pyramidal system because their fibers run in the medullary pyramids. More recently, the pyramidal/extrapyramidal nomenclature has been dropped in favor of more descriptive terms such as direct (corticospinal and corticobulbar) and indirect activation pathways in the motor system. Physiologically, the corticospinal and corticobulbar are monosynaptic; an upper motor neuron (UMN) projects and synapses on a lower motor neuron (LMN) which in turn innervates skeletal muscle. Thus, they directly control voluntary motor activity. However, there are numerous other polysynaptic pathways that also influence movement, which were referred to as the extrapyramidal system. For the purposes of this discussion, theses tracts will be identified as indirect pathways. In the indirect system, neuronal activity also begins in the cortex and then project to structures such as the basal ganglia, brainstem nuclei, and cerebellum before synapsing on LMN (). The role of the indirect pathways is to modify or influence neural impulses originating in the cerebral cortex. These pathways include the rubrospinal, reticulospinal, vestibulospinal, and tectospinal tracts as well as the basal ganglia and cerebellum ().
Fig. 17.1 Connections of the cortex with the basal ganglia and cerebellum: programming of complex movements. The pyramidal motor system (the primary motor cortex and the pyramidal tract arising from it) is assisted by the basal ganglia and cerebellum in the planning and programming of complex movements. While afferent fibers of the motor nuclei (green) project directly to the basal ganglia (left) without synapsing, the cerebellum is indirectly controlled via pontine nuclei. The motor thalamus provides a feedback loop for both structures. The efferent fibers of the basal nuclei and cerebellum are distributed to lower structures including the spinal cord. The importance of the basal ganglia and cerebellum in voluntary movements can be appreciated by noting the effects of lesions in these structures. Although diseases of the basal ganglia impair the initiation and execution of movements (e.g., in Parkinson’s disease), cerebellar lesions are characterized by uncoordinated movements (e.g., the reeling movements of inebriation caused by a temporary toxic insult to the cerebellum). (Reproduced with permission from Schuenke M, Schulte E, Schumacher U. THIEME Atlas of Anatomy Second Edition, Vol 3. ©Thieme 2016. Illustrations by Markus Voll and Karl Wesker.)
Brainstem Nuclei and Tracts
The brainstem houses several nuclei that are important in movement. UMN in the cortex directly influences spinal cord circuits by synapsing on LMN in the ventral horn of the spinal cord or by synapsing on cranial nerve motor nuclei in the brainstem. Indirectly, some cortical neurons influence movement via polysynaptic pathways involving the red nucleus, vestibular nuclei, reticular nuclei, and from neurons located in the superior colliculus of the midbrain. These nuclei are associated with descending motor tracts that influence movement ().
Fig. 17.2 Descending tracts of the extrapyramidal motor system. The neurons of origin of the descending tracts of the extrapyramidal motor system* arise from a heterogeneous group of nuclei that includes the basal ganglia (putamen, globus pallidus, and caudate nucleus), the red nucleus, the substantia nigra, and even the motor cortical areas. The following descending tracts are part of the extrapyramidal motor system: (A) Rubrospinal tract, (B) Olivospinal tract, (C) Vestibulospinal tract, (D) Reticulospinal tract, (E) Tectospinal tract. These long descending tracts terminate on interneurons which then form synapses onto alpha and gamma motor neurons, which they control. Besides these long descending motor tracts, the motor neurons additionally receive sensory input. All impulses in these pathways are integrated by the alpha motor neuron and modulate its activity, thereby affecting muscular contractions. The functional integrity of the alpha motor neuron is tested clinically by reflex testing. (Reproduced with permission from Schuenke M, Schulte E, Schumacher U. THIEME Atlas of Anatomy Second Edition, Vol 3. ©Thieme 2016. Illustrations by Markus Voll and Karl Wesker.)
Rubrospinal Tract
-
The rubrospinal tract (RST) originates from the red nucleus (RN) of the midbrain ().
-
The red nucleus receives input from the motor cortex and the cerebellum.
-
The rubrospinal tract represents an alternative to the corticospinal tract by which motor commands can be sent to the spinal cord.
-
The rubrospinal tract is more prominent in species that use limbs for locomotion. In humans, the tract is primarily directed to cervical levels in the spinal cord and its size is somewhat diminished. In lower species, it continues into the lumbar area.
Fig. 17.3 The rubrospinal tract originates from the red nucleus of the midbrain. It receives input from the motor cortex and the cerebellum. It facilitates control of motor neurons supplying flexors of the upper extremity (among other functions). (Reproduced with permission from Alberstone CD, Benzel EC, Najm IM, et al. Anatomic Basis of Neurologic Diagnosis. © Thieme 2009.)
Reticulospinal Tract
The reticular formation forms the core of the brainstem. It extends from the caudal medulla and continues rostrally to include the midbrain. There are two reticulospinal tracts originating from the reticular formation: the lateral, which arises from the medulla, and the medial, which originates from the pons ().
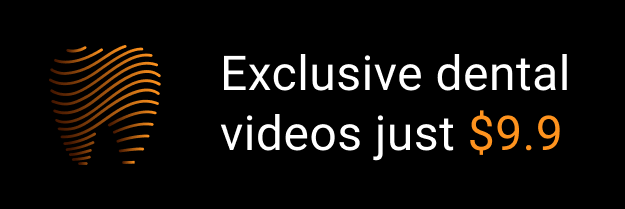