Abstract
Objective
Imperfect polymer formation as well as collagen’s susceptibility to enzymatic degradation increase the vulnerability of hybrid layers over time. This study investigated the effect of new dimethyl sulfoxide (DMSO)-containing pretreatments on long-term bond strength, hybrid layer quality, monomer conversion and collagen structure.
Methods
H 3 PO 4 -etched mid-coronal dentin surfaces from extracted human molars (n = 8) were randomly treated with aqueous and ethanolic DMSO solutions or following the ethanol-wet bonding technique. Dentin bonding was performed with a three-step etch-and-rinse adhesive. Resin-dentin beams (0.8 mm 2 ) were stored in artificial saliva at 37 °C for 24 h and 2.5 years, submitted to microtensile bond strength testing at 0.5 mm/min and semi-quantitative SEM nanoleakage analysis (n = 8). Micro-Raman spectroscopy was used to determine the degree of conversion at different depths in the hybrid layer (n = 6). Changes in the apparent modulus of elasticity of demineralized collagen beams measuring 0.5 × 1.7 × 7 mm (n = 10) and loss of dry mass (n = 10) after 30 days were calculated via three-point bending and precision weighing, respectively.
Results
DMSO-containing pretreatments produced higher bond strengths, which did not change significantly over time presenting lower incidence of water-filled zones. Higher uniformity in monomer conversion across the hybrid layer occurred for all pretreatments. DMSO-induced collagen stiffening was reversible in water, but with lower peptide solubilization.
Significance
Improved polymer formation and higher stability of the collagen-structure can be attributed to DMSO’s unique ability to simultaneously modify both biological and resin components within the hybrid layer. Pretreatments composed of DMSO/ethanol may be a viable-effective alternative to extend the longevity of resin-dentin bonds.
1
Introduction
Despite all significant advances in dental adhesion during the past decades, resin-dentin bonding remains a challenge [ ]. Dentin is a biologically active histologic tissue characterized by an intrinsically hydrated and complex structure containing several endogenous proteases. Bonding to this highly mineralized dynamic structure constitutes a unique form of tissue engineering in which demineralized collagen is used as the scaffold for resin infiltration to couple restorative composites to the underlying mineralized dentin. Unfortunately, hybrid layers formed during collagen hybridization with methacrylate resin monomers are often referred to as the weakest link in resin-dentin interfaces [ , ]. Poorly infiltrated demineralized collagen between hybrid layers and mineralized dentin is inherent to the traditional etch-and-rinse mechanism [ , ]. It is present in even less aggressive self-etch dentin bonding approaches, albeit to a lesser extent [ , ]. Irrefutably, the presence of such vulnerable collagen network further challenges dentin-bond durability [ , ]. No resin-dentin bonding protocol created hitherto is able to produce perfectly hybridized dentin interfaces within clinically reasonable time [ , , ]. Furthermore, activation of endogenous enzymes during bonding contributes to rapid collagen hydrolysis over time [ ] damaging the link between resin components and dentin substrate. Since collagen fibrils serve as reinforcement against cyclic crack growth within the resin-dentin interfaces, collagen degradation invariably creates detrimental weak links [ ]. In this context, maintenance of collagen integrity over time is crucial to the anchorage and durability on resin-dentin interfaces [ , ].
The quality of the polymer-network within the hybrid layer can also be problematic [ , ]. Even though exceedingly high degree of conversion values in the hybrid layer have been commonly reported for different adhesive systems [ ], some well above 80% [ , ], the reality may be unfulfilling [ , ]. Herein, preservation of the demineralized collagen network over time protected by a well-formed polymer-network is of utter importance for the durability of hybrid layers [ ].
Considering such drawbacks, new resin-based adhesives and countless bonding techniques have been proposed to overcome such limitations, but only few protocols have been truly considered for clinical use. The ethanol wet-bonding technique was proposed in 2007 [ ] following etch-rinse bonding and it is considered one of the most effective strategies [ , ] to improve hybrid layer formation in vitro, especially when combined with hydrophobic-bonding monomers [ ]. However, it is technique sensitive and clinically impractical due to the time needed for successive ethanol applications for adequate dentin dehydration [ , ] and detrimental effects on composite durability in vivo have been reported [ ]. Reduction of the water-contamination effect as well as technique simplification could aid the implementation of such bonding approach to truly benefit resin-dentin bonding [ , , ].
Recently, dimethyl sulfoxide (DMSO) has emerged as a new potential solvent in adhesive dentistry [ ] displaying innumerous properties that may benefit resin-dentin bonding [ , ]. DMSO [(CH 3 ) 2 SO] is a polar aprotic colorless solvent classified as an organosulfur complex, which is capable of dissolving both polar and nonpolar compounds [ ]. It is miscible in several organic solvents, in resin monomers commonly used in dentistry [ ] and in water [ ]. DMSO can: ( i ) hydrogen-bond to proteins; ( ii ) increase collagen fibril interspacing [ ]; ( iii ) improve dentin wettability [ ]; ( iv ) facilitate monomer penetration within demineralized dentin [ ]; ( v ) lower the activity of endogenous hydrolytic enzymes [ , ] and ( vi ) reduce the technique sensitivity in etch-and-rinse dentin bonding, even under drastic air-drying conditions [ ]. DMSO also facilitates radical polymerization in solvent‐monomer mixtures [ ]. However, the mechanisms involving hybrid layer improvement by DMSO are not yet fully understood. For instance, monomer polymerization in loco across the hybrid layer and its impact on the mechanical properties of collagen remain unknown. Therefore, the aim of this study was to examine the central hypothesis that both resin and collagen components of the hybrid layer may be simultaneously optimized by binary solutions of DMSO, containing water or ethanol, at different concentrations. The tested null hypotheses were that the etch-and-rinse bonding approach associated to DMSO-pretreatments: ( i ) would have no effect on the long-term resin-dentin bond strength; ( ii ) the mechanical properties/collagen integrity and ( iii ) the polymer network formed within the hybrid layer would not be affected.
2
Materials and methods
Extracted sound human third molars were obtained with informed consent from patients (18–32 years) under a protocol approved by the University of Oulu, Finland (#23-2003) in accordance with local regulations. The primary indications for tooth extractions were not related to the present study. After the extractions, teeth were stored at 4 °C in 0.9% NaCl containing 0.02% NaN 3 to prevent microbial growth and were used within 1 month.
2.1
Experimental design and bonding procedures
Specimen preparation followed the Academy of Dental Materials guidance of in vitro testing for non-trimmed microtensile bond strength testing [ ]. Teeth were coronally sectioned under water cooling to expose flat mid-coronal dentin surfaces using a slow speed diamond saw (Isomet, Buehler Ltd, Lake Bluff, IL, USA). Absence of remaining enamel on the dentin surfaces was verified with a stereomicroscope (Leica M60, Leica Microsystems, Wetzlar, Germany) at 40× magnifications. Roots were removed 1 mm below the cervical line and discarded. Exposed dentin surfaces were standardized by wet-polishing with 320-grit SiC paper for 60 s. Crown segments (n = 8/group) were randomly allocated into 4 groups following a study design with two factors: (i) “dentin treatment” in four levels consisting of no treatment, ethanol wet-bonding and use of 50 vol% DMSO (Dimethyl Sulfoxide, Sigma-Aldrich, St Louis, MO, USA) dissolved in either water (DMSO/H 2 O) or ethanol (Sigma-Aldrich) (DMSO/EtOH); and “storage time” in two levels 24 h and 2.5 years in artificial saliva. Dentin surfaces were initially etched for 15 s with H 3 PO 4 32 wt% (Scotchbond Universal Etchant, 3M ESPE, St. Paul, MN, USA) and rinsed for 15 s. The control group was conventionally wet bonded following manufacturer’s instructions. After rinsing, dentin surfaces were blot dried with lint-free absorbent paper until water uptake by capillarity was no longer detected. Dentin surfaces remained partially moist but not overwet. In experimental groups, dentin was treated with DMSO-containing solutions or followed the ethanol-wet bonding technique. For the ethanol-wet bonding [ , ], etched dentin surfaces were dehydrated with a series of increasing ethanol concentrations (50%, 70%, 80%, 95% and 3 × 100%, 30 s each; i.e. 3 min 30 s in total). During this procedure, dentin surfaces were kept fully immersed in the ethanol solutions to avoid collapse of demineralized collagen. The 50% DMSO (v/v) solutions were prepared by mixing equal volumes of DMSO in distilled water or ethanol. 50 μL of DMSO/H 2 O or DMSO/EtOH solutions [ , , ] were applied on the etched-dentin surfaces, left undisturbed for 60 s and blot dried until paper filters presented no moisture absorption from the bonding surface by capillarity. Dentin surfaces presented no visible superficial moisture before bonding. For dentin hybridization, one gold-standard three-step etch-and-rinse adhesive (Scotchbond Multi-Purpose: SBMP, 3 M ESPE) was used following manufacturer’s instructions. SBMP’s Primer and Bond were applied sequentially for 10 s and air dried for 10 s each. Both were actively applied with manual pressure of approximately 4.0 g ± 1.6 [ ]. Adhesive procedures were carried out in a controlled environment with a temperature of 24 °C and a relative humidity of 45–55%. Composite blocks were built with a nanofilled composite resin (Filtek Z350 XT, 3M ESPE, Shade A2) in 3 increments of 1.5 mm. Each increment was light-cured for 20 s using a LED light-curing unit (Elipar Deepcure, 3 M ESPE) at 1200 mW/cm 2 . All bonding procedures were carried out by a single operator. The restored crown segments were stored in distilled water for 24 h at 37 °C to allow water sorption and postoperative polymerization. Resin–dentin beams were produced with a cross sectional area of approximately 0.9 mm 2 by sectioning the restored crowns longitudinally in mesio-distal and buccal-lingual directions perpendicular to the bonded interface with a slow-speed diamond saw (Isomet, Buehler Ltd).
2.2
Resin-dentin beam storage
Resin-dentin beams were randomly selected for the microtensile test under two conditions: after 24 h of storage in distilled water at 37 °C and long-term aging for 2.5 years in artificial saliva (pH 7.4) at 37 °C containing 5 mM HEPES, 2.5 mM CaCl 2 ·H 2 O, 0.05 mM ZnCl 2 , and 0.3 mM NaN 3 [ ] and changed biweekly in accordance with protocols previously described. In order to obtain a research design balanced by tooth dependency [ ], resin-dentin beams from the same tooth were submitted to both testing periods ( i.e. 24 h and 2.5 years). The nanoleakage evaluation included beams aged for 2.5 years to focus on the effect of aging on hybrid layer integrity.
2.3
Microtensile bond strength (μTBS)
Resin-dentin bond strength evaluation followed the Academy of Dental Materials guidelines for non-trimmed μTBS testing [ ]. A minimum of 8 beams per tooth were tested on each storage period. Specimens were tested by a blinded operator. Beams were individually attached to a custom made micro-tensile testing jig using a cyanoacrylate adhesive (Loctite 416, Henkel Corp., Dublin, Ireland) and tested under tensile forces (Bisco, Schaumburg, IL, USA) at a crosshead speed of 0.5 mm/min until failure. Pretesting failures were recorded and considered as 0 MPa for the statistical analyses. The cross-sectional area of each beam was measured with a digital caliper for μTBS calculation in MPa. Tooth was considered the statistical unit, the bond strength average of beams tested at each time period representing the μTBS for each tooth (n = 8). Both surfaces of fractured resin-dentin beams were analyzed by scanning electron microscopy (SEM) (Phenom ProX, Phenom-World, Eindhoven, Netherlands) to determine fracture patterns. The fracture modes were classified as: cohesive (failure exclusive within dentin or resin composite); adhesive failure (failure at resin/dentin interface); and mixed failure (failure at resin/dentin interface with cohesive failure of the neighboring substrates).
2.4
Nanoleakage evaluation
Three resin-dentin beams per tooth (n = 8) aged for 2.5 years were randomly selected to evaluate the integrity of hybrid layers through the measurement of silver nitrate uptake at the bonded interface. Nanoleakage evaluation was performed according to a protocol previously described by Tay et al., [ ]. Briefly, resin-dentin beams were initially wet-polished with 2000-grit SiC paper and coated with two layers of nail varnish applied up to 1 mm of the bonded interfaces. After rehydration in distilled water for 1 h, beams were immersed for 24 h in 50% (w/v) ammoniacal silver nitrate (pH 9.5), and thoroughly rinsed in distilled water for 120 s. Samples were then immersed in photo-developing solution (Kodak Professional D-76 developer, Kodak Rochester, NY) for 8 h under a fluorescent light to reduce silver ions into metallic silver grains within the water-filled voids along the bonded interface. Beams were embedded in epoxy resin, wet-polished with 600-, 1000-, and 2000-grit SiC paper (Carbimet, Buehler Ltd.,) and 1, 0.25 (MetaDi, Buehler Ltd) and 0.05 μm (MasterPrep, Buehler Ltd) polishing pastes. Resin-dentin beams were ultrasonically cleaned in distilled water after each polishing step, air dried for 2 h, mounted on stubs, dried in silica overnight and carbon-sputtered. Nanoleakage extension was semi-quantified using SEM imaging on backscattering mode at 10 kV (Phenom ProX, Phenom-World). A series of sequential micrographs (2000× magnification) were obtained from each beam to detect silver deposition within the adhesive interface. Total silver nitrate uptake was measured on the 2D images using open-source image software (ImageJ, National Institute of Health, Bethesda, MD, USA) by a single-blinded examiner. The overall extension of silver uptake (μm) along the bonded interface was calculated and converted into percentage values. Silver uptake patterns were evaluated at higher magnifications (4000−10000×).
2.5
Collagen apparent modulus of elasticity (E)
One disc measuring 0.51 (±0.06) mm from the mid-coronal dentin region of each tooth (n = 10/group) were sectioned perpendicularly to the its long axis using a slow speed diamond saw (Isomet, Buehler Ltd,) under water cooling, wet-polished with 600-grit SiC paper to remove superficial imperfections from the low speed diamond blade and to fine adjust their thickness. The discs were then sectioned mesial-distally to produce rectangular dentin beams measuring approximately 0.5 mm thickness × 1.7 mm width × 7 mm length [ , ]. A dimple was made on the corner of each beam on the occlusal surface allowing repeated measures to be performed on the same surface. Beams were then demineralized in 10 wt% phosphoric acid [ , ] for 7 h at 24 °C under constant stirring and thoroughly rinsed with distilled water for 10 min. Digital radiography was used to document the absence of residual minerals. Digital images were obtained on a stereo microscope (Leica M60, Leica Microsystems) and thickness and width dimension of the demineralized beams were precisely measured using ImageJ. Beams were placed on a 3-point flexure jig with 5 mm span length between supports fully immersed in distilled water and tested under flexure to 3% strain [ ] with a displacement rate of 0.5 mm min −1 using a 5 N load cell (SMT1-5N, Interface, Scottsdale, AZ, USA) mounted on a universal testing machine (Autograph AGS-X, Shimadzu, Japan). After maximum displacement, the load was immediately returned to 0% stress without further holding to prevent creep of the demineralized collagen matrix. Specimens were tested by a blinded operator. Load-displacement curves were converted to stress-strain curves and the apparent modulus of elastic ( E ) in MPa was calculated using the following formula: <SPAN role=presentation tabIndex=0 id=MathJax-Element-1-Frame class=MathJax style="POSITION: relative" data-mathml='E=mL3/4bh3′>?=??3/4?ℎ3E=mL3/4bh3
E = m L 3 / 4 b h 3
where m is the steepest slope of the linear portion of the load- displacement curve (N/mm), L is the span length (5 mm), b is the width of the test specimen and h is the beam thickness. The 3-point bending test was selected for evaluation of the apparent elastic modulus because it is nondestructive and allows repeated measurements to be performed. After the baseline measurements, dentin beams (n = 10/group) were distributed into 5 balanced groups, so that their mean values were not statistically different. To allow proper solvent diffusion throughout the entire sample extension [ , ], demineralized beams were then immersed for 2 h in the DMSO solutions used for the bonding protocols ( i.e. 50% DMSO/H 2 O and 50% DMSO/EtOH), in EtOH, following the sequential EtOH wet-bonding dehydration protocol used except that the total time in the final EtOH step was 2 h. Undiluted DMSO was also included as a treatment. Beams were retested fully immersed in their respective solutions. After 15 min of rehydration, beams were retested in distilled water. Following this immediate testing in their respective treatment solutions, samples were stored for 7 and 30 days in artificial saliva at 37 °C and retested. Demineralized dentin beams without any treatment served as a negative control group.
2.6
Loss of dry mass (L dm )
Fifty demineralized dentin beams were dehydrated in silica under vacuum during 72 h at 24 °C and then desiccated to a constant weight (variations lower than 0.1 mg over 6 h; approximately 48 h in total). The initial dry mass ( M1 ) was followed gravimetrically to the nearest 0.01 mg using an analytical balance (XS 105, Mettler Toledo, Hightstown, NJ, USA). After the initial dry mass measurement, each dried-shrunken dentin beam was hydrated in water for 2 h before being immersed in the treatment solutions for 2 h: EtOH following the ethanol-wet bonding protocol, pure DMSO, DMSO/H 2 O and DMSO/EtOH (n = 10). Untreated collagen beams served as control. Subsequently, treated beams were rehydrated in artificial saliva for 2 h and placed in separate polypropylene tubes containing 3 ml of artificial saliva. Samples were placed in a shaking-bath to facilitate artificial saliva diffusion within collagen fibrils. After 30 days of incubation at 37 °C, the beams were rinsed with water for 10 min and sonicated for 5 min in distilled water to remove media salts. Determination of the dry mass was repeated under the same conditions after dehydration in silica ( M2 ). Specimens were tested by a blinded operator. Loss of dry mass ( L dm ) was calculated according to the formula: <SPAN role=presentation tabIndex=0 id=MathJax-Element-2-Frame class=MathJax style="POSITION: relative" data-mathml='Ldm%=M2-M1M1.’>???(%)=?2−?1?1.Ldm%=M2-M1M1.
L d m % = M 2 – M 1 M 1 .
Loss of dry mass over time provides an indirect measurement of collagen solubilization by endogenous enzymes.
2.7
Adhesive conversion in the hybrid layer (DC)
Additional resin-dentin beams were created for each group (n = 6) following the previously described bonding protocols with the exception that samples were kept in 100% humidity for 24 h at 37 °C before testing instead of water-immersion. The rationale for this change was to limit HEMA elution from the bonded interface to reduce the discrepancy between “apparent” and “real” adhesive conversion values [ ]. In order to more precisely locate the hybrid layer, samples were embedded in epoxy-resin and wet-polished with 600, 1000 and 2000-grit SiC paper (Buehler Ltd., Lake Bluff, IL, USA), ultrasonically cleaned for 2 min after the last step. Since water is a weak Raman scatterer, samples were not dehydrated. Raman spectra of resin-dentin interfaces were collected using Raman microscope (Thermo DXR2xi, Thermo Fisher Scientific, Madison, USA) equipped with a 785 nm laser and 400 lines/mm grating resulting in approximately 5 cm −1 spectral resolution and 3300–50 cm −1 spectral range. Spectra were obtained with a 100× objective at the top half and bottom half of the hybrid layer, in arbitrary areas of intertubular dentin. Care was taken to select areas between dentin tubules. Instrumental calibration was performed according to manufacturer’s specifications before each experiment. Specimens were tested by a single blinded operator. The reactive peak at 1639 cm −1 is attributed to the methacrylate CC in both HEMA and Bis-GMA monomers [ , ]. Upon polymerization, the area of this peak diminishes as C C are converted into CC to form the polymer chain. An unchanging reference peak at 1609 cm −1 is correlated with the aromatic C C [ , ]. The ratio of double-bond content of monomer to polymer in the hybrid layer was calculated according to the following formula:
DC%=1-RCuredRUncured×100
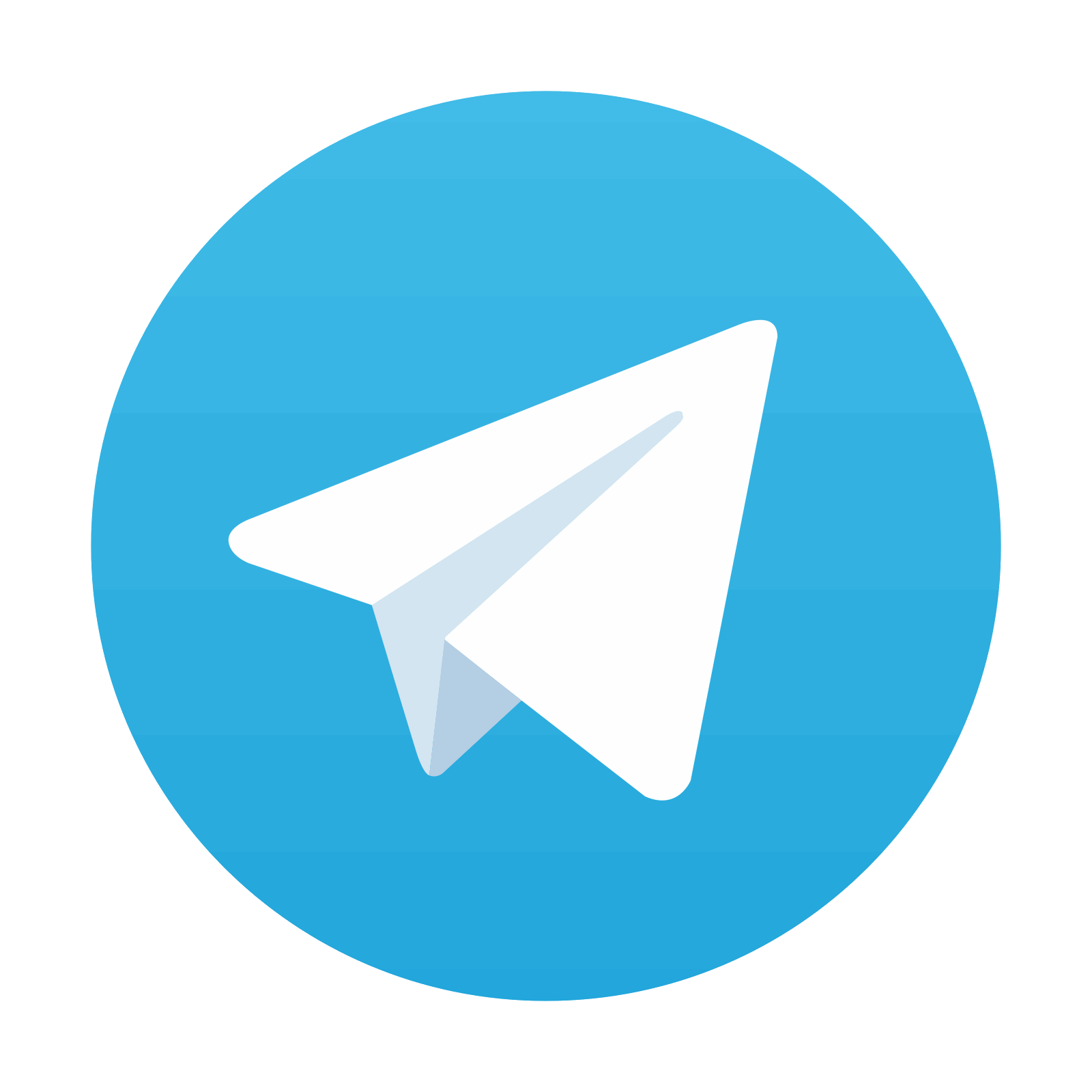
Stay updated, free dental videos. Join our Telegram channel

VIDEdental - Online dental courses
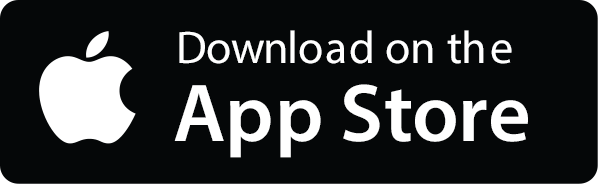
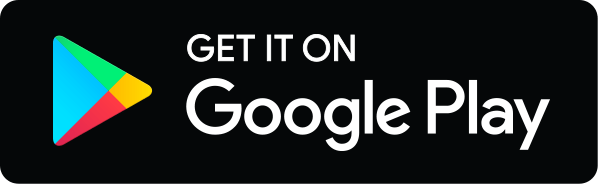
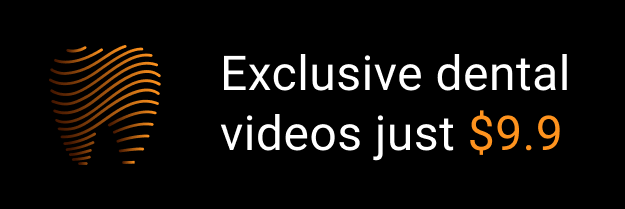