Fig. 2.1
(a) Diagram of sensitive dentin showing exposed tubules in an area of dentin erosion or gingival recession. Stimuli, for example, air blasts directed toward the dentin surface, induce outward fluid shifts in the tubules which activate the nerve ending located in the deep dentin and pulp. The odontoblasts respond to various forms of pain-producing stimuli, but it is not clear if these cells can activate the intradental nerve fibers. Treatments for sensitive teeth can act by reducing the dentin permeability or by reducing the excitability of the intradental nerves (Reprinted with permission from Markowitz (2010, 2013)). (b) Schematic diagram showing the physiological processes leading to pain-associated DH. Fluid flow in the dentin tubules triggers nerve responses which lead to pain perception in the central nervous system (CNS). The odontoblasts may also respond to certain pain-provoking stimuli. Some stimuli, for example, cold, may also cause pain by directly activating the nerve endings. The relationship between the magnitude of a stimulus applied to the dentin and pain perception is by no means fixed. Dentin permeability, the biological environment of the dental pulp, and central psychological and physiological factors are variables that can alter pain processing (Reprinted with permission from Markowitz (2009))
Odontoblasts, the principal cellular element at the dentin-pulp boarder, have a process extending approximately one-third the distance to the dentinoenamel junction (Byers and Sugaya 1995). Recent physiological and gene expression studies have demonstrated that these dentin-forming cells respond to pain-provoking stimuli in a way that was similar to sensory receptors (Fig. 2.1b) (Magloire et al. 2010; El Karim et al. 2011; Chung et al. 2013). It is not clear, however, whether odontoblasts can activate the intradental nerves, since conventional synaptic structures have not been identified linking these cells. Trauma to the dentin that destroys the odontoblast layer fails to abolish dentin hypersensitivity (DH). Odontoblasts then may play a role but may not be critical to tooth sensitivity (Brännström 1963, 1968; Lundy and Stanley 1969; Lilja et al. 1982).
Gysi (1900) was the first investigator to speculate that the sensitivity of dentin was due to stimulus-evoked fluid shifts in the dentin tubules. Brännström and his colleagues systematically studied fluid movement in dentin in vitro that occurred in response to thermal, evaporative, tactile, and osmotic stimuli (Brännström 1962, 1965, 1966, 1986, 1992; Brännström et al. 1967). In general, stimuli that elicit pain when applied to exposed dentin also induce fluid shifts that can be studied in laboratory experiments. Brännström’s theory was referred to as the hydrodynamic hypothesis. Later animal experiments that directly linked transdentin fluid movements with excitation of the intradental nerves (Matthews and Vongsavan 1994) gave credence to the view that intradental nerves act as mechanoreceptors that are activated by dentin fluid shifts. The hydrodynamic mechanism in teeth represents a novel adaptation of fluid flow-sensing mechanoreceptors to the unique anatomical environment of the dentin pulp complex.
Fluid shifts, however, are not the only way that pain can be evoked. As with the other parts of the body, intradental nerves can respond directly to pain-producing stimuli regardless of the dentin fluid shifts evoked by those stimuli (Chung et al. 2013). Cold foods and beverages may, in part, stimulate nerves directly by activating thermal receptors located on intradental nerve endings (Park et al. 2006). Even where the intensity of the pain response does not correlate with the magnitude of the dentin fluid shifts, the dentin tubules act as conduits whereby the external stimuli reach the pain transduction mechanism (Chidchuangchai et al. 2007).
Although considered “pathological,” DH may serve important physiologic functions in humans and other species. The intradental nerves participate in the sensation of biting force and, in this way, may protect the teeth from fracture (Olgart et al. 1988). Dentin sensation arising in the elongated tusk of the narwhal whale appears to contribute to the animal’s ability to sense the tonicity in its immediate environment. This tusk has cementum channels connecting dentin tubules to the tooth’s surface. This sensory input is important in the Arctic since it helps the animal avoid being trapped by ice (Nweeia et al. 2014).
Understanding pain plasticity is critical to appreciating the impact of disease states, therapy, and host factors in modulating this experience (Pashley 2013). Dentin permeability and intradental nerve excitability are peripheral loci where disease processes can upregulate pain and where therapeutic strategies seek to interrupt the events leading to pain transmission. Analysis of these peripheral factors highlights the relationship of DH to patient behavior and other dental health issues, for example, tooth wear, erosion, caries, and periodontal disease (see Chaps. 4 and 10). Less well understood, but equally important, however is the role of central nervous system plasticity in explaining the large degree of variability observed in the intensity of DH symptoms and the impact of this type of pain on quality of life (Sessle 2000; Bekes and Hirsch 2013; Sixou 2013) (see Chap. 9).
The Anatomical Substrates of Dentin Hypersensitivity (DH)
Intense cold can elicit pain in healthy intact teeth. This form of stimulation is generally used to determine pulp vitality in clinical examinations. Hot foods and beverages can also elicit pain in healthy teeth. Cold, however, has been observed to be a more reliable modality for pulp vitality testing (Fuss et al. 1986). In intact teeth, dentin is covered coronally by enamel and by cementum on the root surfaces. These external coverings seal the tubules so well that there are little intratubular fluid shifts in response to the application of hydrodynamic stimuli (thermal, evaporative, tactile, or osmotic). Once the peripheral coverings of dentin are removed by abrasion, erosion, caries processes, or scaling procedures, dentin becomes hyperconductive, compared to its previous unexposed condition. In this state, hydrodynamic stimuli elicit large fluid shifts that evoke pain (Pashley 2013).
Dentin
Compared to nonsensitive dentin, sensitive areas have exposed patent tubules (Absi et al. 1987, 1989; Yoshiyama et al. 1989). In human subjects, the severity of sensitivity symptoms correlated with the number of open tubules/dentin surface area and the diameters of those tubules (Kontturi-Nahri and Närhi 1993). Root scaling and restorative procedures leave the dentin surface covered by a layer of burnished cutting debris called the smear layer (Pashley 1984) (Fig. 2.2). This cutting debris is actually forced into the tubule orifices for approximately 1 μm and is then covered by a further 1 μm of smear layer. The smear layer reduces the hydraulic conductance (the capacity of dentin for pressure-driven, convective flow), about 10–30 times compared to what it would be in the absence of these surface materials (Pashley et al. 1981; Carrilho et al. 2007). When the smear layer and smear plugs are dissolved by the organic acids present in the diet or produced by plaque microorganisms, the exposed dentin can become sensitive (Pashley 1986).
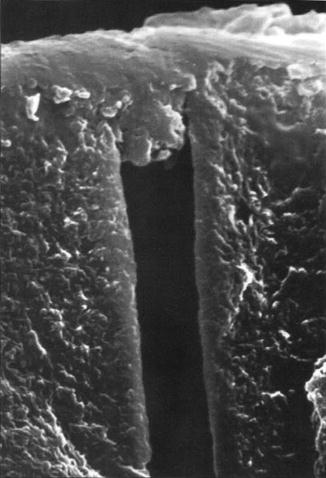
Fig. 2.2
Smear layer covering dentin cut with a high-speed carbide bur. Note that cutting debris extends into the dentin tubule about 1–2 μm. The combination of smear layer/smear plugs reduces fluid flow across dentin by 90 %. Loss of smear layers and smear plugs increases dentin fluid flow and hence DH (Used with permission from Pashley (1992))
The dental pulp is surrounded by mineralized dentin. Measurements of the tissue pressure of pulpal soft tissues have shown that its hydrostatic pressure is positive, rather than being negative as is common in the skin (Guyton and Hall 2000). Intraocular, intramedullary, intracranial, and intrapulpal tissue pressures are all positive 14–16 mmHg (Matthews and Vongsavan 1994; Ciucchi et al. 1995; Heyeraas and Berggreen 1999; Guyton and Hall 2000). Positive pulpal tissue pressure is probably the result of the average capillary hydrostatic pressure exceeding the oncotic pressure of plasma proteins and the relative meager lymph drainage of the pulp (Pashley 1992; Heyeraas and Berggreen 1999). This positive tissue pressure in the pulpal soft tissues is responsible for the slow outward seepage of dentin fluid whenever the outer coverings (enamel or cementum) are lost (Ciucchi et al. 1995). The rate of this outward fluid flow that is present in the absence of hydrodynamic stimulation is not sufficient to activate pulpal sensory nerves in the pulp. This was shown definitively in cat teeth by Vongsavan and Matthews (1992) and by Matthews and Vongsavan (1994).
Stimulus-induced fluid shifts occur when the baseline outward convective flow accelerates or reverses in response to probing, air blasts, or thermal changes (Anderson et al. 1967; Matthews et al. 1993; Pashley et al. 1996; Andrew and Matthews 2000; Vongsavan et al. 2000). Experiments using hydrostatic pressure stimuli on human and animal teeth (Fig. 2.3a) demonstrate that the intradental nerves are more sensitive to outward flow than to inward fluid displacements (Fig. 2.3b, c) (Matthews and Vongsavan 1994; Andrew and Matthews 2000; Charoenlarp et al. 2007; Vongsavan and Matthews 2007).
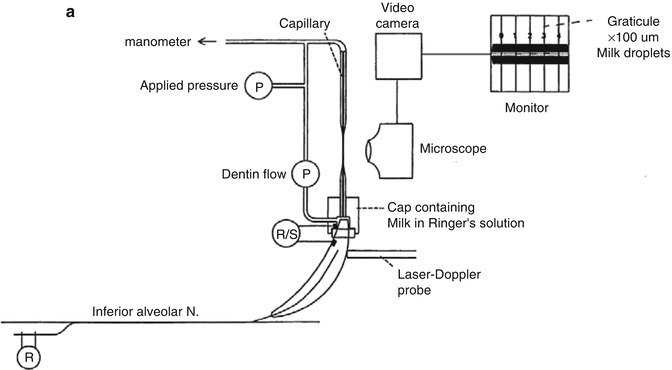
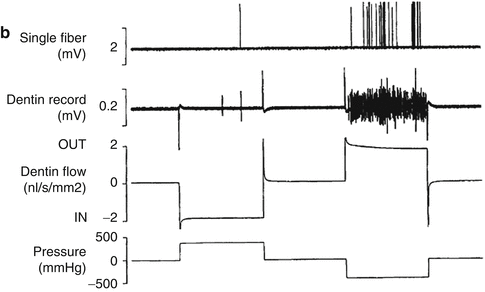
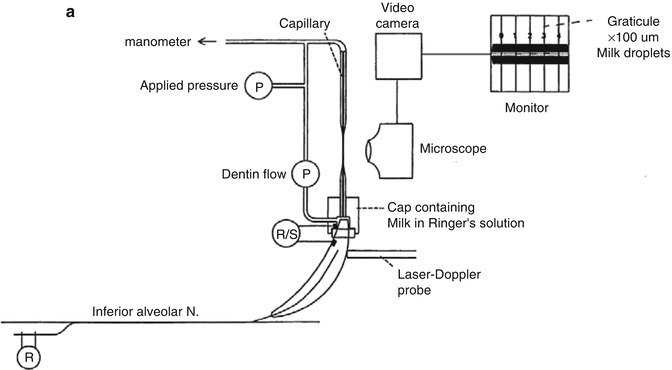
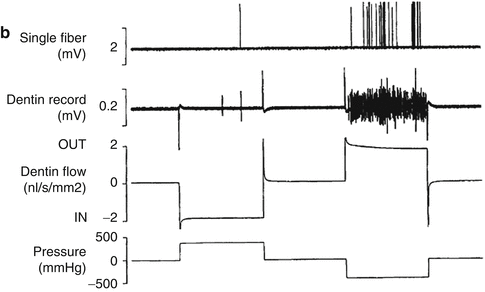
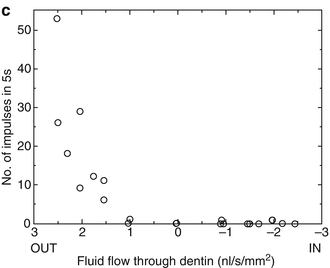
Fig. 2.3
(a) Experimental arrangement used to examine the effect of hydrostatic pressure application to an etched dentin surface on transdental fluid flow and intradental nerve activity in animal experiments. Intradental nerve activity was recorded from single fibers isolated from the inferior alveolar nerve and from multiple units in the dentin. (b) Following the application of positive pressure to the dentin surface, there is inward dentin fluid flow. This results in little activation of the intradental nerves. In contrast, negative pressure induces outward flow; this is associated with a vigorous nerve response recorded from both the dentin and the single-unit recordings. (c) Relationship between transdentin flow rates produced in response to pressure applications, and number of nerve impulse recorded from the dentin. Inward flow evoked little in the way of nerve responses. Outward transdentin flow above approximately 0.75 nl/s/mm2 evoked nerve responses. The magnitude of the nerve response appeared to be positively associated with the outward flow rate (Vongsavan and Matthews (2007) reproduced with permission)
Both tactile stimulation and air blasts are commonly used stimuli in DH clinical studies (Gillam et al. 2000) (see Chaps. 5 and 7). Intradental nerve responses to these stimuli may also be evoked when the smear layer is removed from dentin, and not in its presence (Hirvonen et al. 1984). Abrading the dentin to form a smear layer or applying agents to the dentin that occlude the dentin tubules abolished these responses.
Pressing the dentin with a sharp explorer induces sufficient inward fluid shift to exceed the pain threshold (Fig. 2.4a). When the pressure is removed, an outward fluid shift occurs due to the elastic recoil of the dentin surface that also activates the intradental nerves (Fig. 2.4b) (Camps et al. 2003).
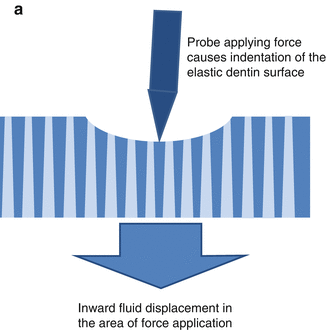
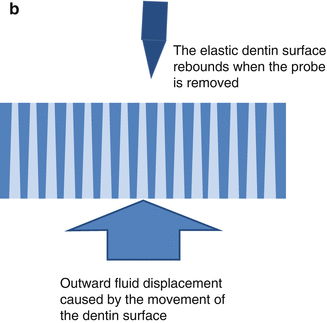
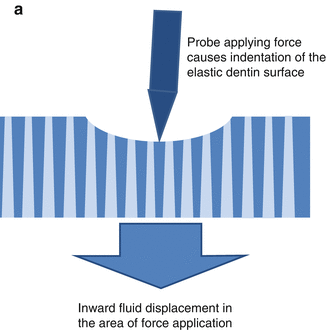
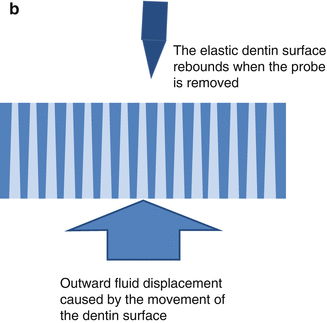
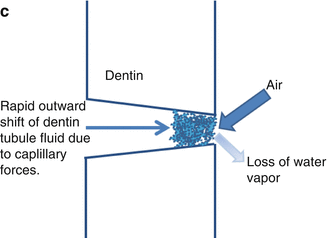
Fig. 2.4
Mechanism by which tactile and air blast stimulations cause pain in sensitive teeth. (a) Tactile stimulation with a sharp probe may cause both plastic (scratching) and elastic (reversible) deformation of the exposed, possibly demineralized, dentin surface. When tactile forces are applied with a sharp probe, the dentin surface indents. Since the dentin tubules widen toward the pulp, this causes an inward fluid shift that may be of sufficient magnitude to stimulate the intradental nerves in the deep dentin and pulp (not shown). (b) Removal of the probe from the dentin surface causes the surface to recoil. This recoil triggers outward dentin fluid flow that is highly excitatory to the intradental nerves (Camps and Pashley 2003; Camps et al. 2003). (c) Air blasts convert fluid in the exposed open ends of the dentin tubules into water vapor that escapes under the influence of the air current. This causes an immediate outward movement of dentin fluid due to capillary forces. The evaporative action of air blasts can also cause cooling of the dentin surface that can induce pain as well. Occlusion of the dentin tubules by a porous substance, such as the smear layer, may result in a near-complete blockage of convective fluid movements but has considerably less impact on fluid shifts induced by air blasts since evaporation of fluid from the tubule orifice will still occur (Matthews et al. 1993)
Air blasting is a very effective provocation of tooth sensitivity pain that acts by inducing rapid outward fluid shifts (Fig. 2.4c) (Matthews et al. 1993; Pashley et al. 1996; Andrew and Matthews 2000). Air blasts evaporate fluid from dentin tubules. This, in turn, produces cooling of the dentin and an outward fluid shift caused by capillary action. Evaporative fluid movement may occur through small or incompletely occluded tubules. It is commonly experienced in clinical practice that superficial dentin as, for instance, in a cavity preparation extending to the dentinoenamel junction (DEJ) is quite sensitive. Superficial dentin has been reported to possess narrow tubules that have a lower hydraulic conductance than deep dentin (Fogel et al. 1988). The dentin tubules do, however, have extensive branching near the DEJ that makes this tissue porous and can sustain evaporative fluid movements (Mjör and Nordahl 1996).
Physiological Factors Altering Dentin Permeability and Sensitivity

(2.1)
-
where Q = volumetric fluid flow (μL)
-
N = number of pores (i.e., tubules per unit area)
-
ΔP = hydrostatic pressure difference across dentin (cm H2O−1)
-
r = radius of dentin tubules
-
η = viscosity of fluid
-
L = length of dentin tubules (cm)
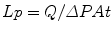
(2.2)
-
where Lp = hydraulic conductance (μL cm−2 min−1 cm H2O−1)
-
Q = volumetric fluid flow (μL)
-
ΔP = hydrostatic pressure difference across dentin (cm H2O−1)
-
A = area of dentin (cm2)
-
t = time in min.
Being a system of tubules arrange approximately parallel (in particular in the mid-coronal dentin), flow and diffusion through dentin follows certain physical laws. Fluid flow is generally expressed as the ease with which fluid can flow across dentin or its hydraulic conductance (Lp), (Eqs. 2.1 and 2.2). That is, hydraulic conductance is simply the measure fluid flow rate (Q) divided by the difference in hydrostatic pressure across dentin forcing the fluid through 1 cm2 of dentin surface area per min (Eq. 2.2). Both convective fluid flow and diffusion are proportional to the number of exposed tubules and inversely proportional to their length. Diffusion across dentin varies with the square of the tubule radius, while convective fluid flow through the dentin tubules varies with the fourth power of the radius (Eq. 2.1). The steep relationship between radius and convective flow is because intratubular fluid flow is laminar (Merchant et al. 1977). That is, concentrically smaller cylinders of fluid slip beneath larger more stationary cylinders adjacent to the walls of the tubules. This causes frictional resistance to fluid flow. If a tubule-occluding agent can reduce tubule radii anywhere throughout the length of the tubule by one-half, the resistance to fluid flow increases not by one-half squared, as in diffusion, but by one-half to the fourth power. Thus, reductions in tubule radius by one-half reduce diffusion to one-fourth of its original value but restrict convective fluid flow to one-sixteenth of its original value. This relationship predicts that dentin tubules need not necessarily be completely sealed in order to reduce DH (Reeder et al. 1978). The dependence of fluid flow on tubule radius, density, and length also explains the anatomic variations that are observed in the permeability of dentin from different sites within a tooth. In general, the deep dentin above a pulp horn of a posterior tooth or above the pulp tip in an anterior tooth has the highest permeability of any area of exposed dentin in that tooth (Pashley et al. 1978, 1987). These areas of dentin also have the highest innervation density (Byers 1984) and tend to be most sensitive.
If one compared the convective flow measured through a dentin specimen of known tubule diameter, thickness, and density with the expected flow calculated for that specimen, the calculated flow is usually an order of magnitude greater than the actual flow (Michelich et al. 1978). Dentin tubules have irregular walls and contain collagen fibers and other structures that partially obstruct flow.
The hydraulic conductance (Lp) of a porous solid is a measure of the ease with which a pressure gradient generates flow through the material (Eq. 2.2). In experiments where dentin flow is measured, hydraulic conductance is a reflection of the dentin’s permeability to convective flow (Reeder et al. 1978). As described above, removal of the smear layer and smear plug increases the dentin’s hydraulic conductance. Hydraulic conductance measurements are used to screen the ability of agents to treat DH by reducing dentin permeability.
When solutes are applied to permeable dentin surfaces, they diffuse through the tubules in proportion to their concentration gradient. The physiological outward flow of dentin fluid reduces the inward flux of solutes by its rinsing action (Pashley and Matthews 1993). Due to the different dependencies of diffusion and flow, partial tubule occlusion can reduce this outward rinsing action of flow and enhance inward diffusion (Pashley et al. 2002). The opposing action of outward flow on inward diffusion is an important factor in limiting transdentin drug delivery, the interaction of restorative materials with dentin, and the effect of bacterial toxins on the dental pulp. The outward flow may also transport host-derived proteins, for example, clotting factors (Pashley et al. 1984) and immunoglobulins into the dentin tubules (Hahn and Overton 1997). These macromolecules may interact with one another or the tubules themselves forming obstructions that subsequently reduce fluid flow.
Solutes applied to the dentin surface also initiate outward osmotic water movements. For example, pellets soaked in saturated calcium chloride can draw sufficient fluid through dentin to elicit pain. This may be used as a clinical test to detect defective restoration margins (Pashley et al. 1996). In general, osmotic water movements through dentin are limited since the tubules are permeable to most low molecular weight solutes (Pashley and Whitford 1980). Large molecular weight solutes, for example, proteins, have a limited ability to diffuse into dentin, particularly in the presence of the smear layer. Under conditions where the penetration of the solutes into dentin is restricted, outward osmosis is favored (Pashley et al. 1979).
The dentin tubules provide the pathway that links dentin stimulation with nerve responses. The dentin tubules are also the routes by which pain can be facilitated by bacterial factors or relived by therapeutic agents that either reduce the dentin permeability or inhibit the activation of the intradental nerves (Markowitz and Pashley 2008; Pashley 2013). Although dentin does not remodel in the same way as bone, the formation of smear layers, tertiary dentin, peritubular dentin, and intratubular mineral can reduce dentin permeability and DH (Mjör 2001). The susceptibility of this hard tissue to acid dissolution and abrasion is an important factor in understanding DH etiological factors and designing preventive strategies that are critical to patient management (Addy 1992) (see Chap. 4).
Nerve Activation
The densely innervated teeth give rise to a spectrum of pain experiences ranging from the agony of acute pulpitis to the sensations encountered by DH sufferers. Clinical research, together with evidence from in vivo studies where the nerve responses to dentin stimulation are recorded, has been useful in determining which of the various sensory nerve fiber types present in the pulp respond to dentin stimulation and in determining the role of dentin permeability in altering the capacity of stimuli to evoke nerve responses (Markowitz and Pashley 2008).
Stimulation of exposed dentin in healthy human teeth results in a sharp, well-localized pain that does not last beyond the duration of the stimulus (Anderson et al. 1967; Anderson and Matthews 1976; Ahlquist and Franzén 1994). This corresponds to pain associated with A-fiber type nociceptor activation evident in other parts of the body. The identity of the nerve fiber type responding to dentin stimulation was confirmed in physiological experiments. In these experiments, the nerve units responding to air blasts, probing, and other stimuli applied to etched dentin were observed to have low electrical thresholds and rapid conduction velocities consistent with A-fibers (Närhi et al. 1982, 1992; Närhi 1985). These A-fibers are myelinated throughout most of their course but lose myelination as they reach the superficial pulp and dentin. This loss of myelination has, however, made characterization of the distal portions of the afferents difficult when viewing histological sections. Recently, it has been demonstrated using immunohistochemical markers that most of the unmyelinated nerve fibers present in the superficial pulp tissue are the extensions of myelinated axons (Henry et al. 2012). In contrast to the intradental A-fibers, slowly conducting pulpal C-fibers are unmyelinated throughout their entire course. These units do not respond to the types of innocuous dentin stimuli that evoke DH pain, but they do respond to direct injurious stimuli, for example, noxious heat, directed to the pulp tissue. Capsaicin, the active ingredient in hot peppers, stimulates C-fibers via the same receptor as is activated by heat but has little effect on the rapidly conducting intradental A-fibers (Närhi et al. 1992; Ikeda et al. 1997).
The relationship between the activation of intradental nerve fiber types and the individual’s pain experience was studied in experiments conducted on human volunteers (Ahlquist and Franzén 1994). In these experiments, the electrophysiological responses of the intradental nerves to tooth stimulation were recorded from dentin cavities at the same time as the experimenters recorded the subject’s pain rating and description. Cold stimulation evoked pain described as sharp and evoked A-fiber impulses recorded from the dentin (Ahlquist et al. 1984). For a series of cold stimulus applications, the intensity of the pain was proportional to the frequency of the nerve response (Fors et al. 1984). In contrast to cold, the application of several algesic (pain-producing) chemicals to deep dentin or pulp evoked dull aching pain and did not produce nerve responses that could be recorded through dentin cavities (Ahlquist et al. 1994). This type of pain was probably due to small-diameter C-fiber activation. These human and animal studies have established a functional classification of the intradental nerves and relate people’s pain experiences to activation of the afferent type and relate tooth sensitivity to other forms of sharp well-localized pain.
Nociceptive afferents may also be classified by histochemical markers, as well as according to types of receptors, ion channels, and transmitters present in the neuron (Chung et al. 2013). Mammalian trigeminal afferents are a heterogeneous population of sensory neurons, most of which convey non-painful mechanosensory information arising in the exquisitely sensitive skin and hairs of the face (Catania 2011), as well as sensation from the mucosa (Sessle and Greenwood 1976). In order to examine the properties of pulpal afferents, histological examination of pulp tissue is performed, or the cell bodies of pulpal afferents are examined in the trigeminal ganglion (Byers et al. 1982, 1987; Pan et al. 2000). Pulpal afferents can be traced to their cell bodies by placing certain rapidly diffusing tracers into dentin cavities, and following a period of time to allow for the transport of these tracers, the cell bodies in the trigeminal ganglion can be visualized (Eckert et al. 1997; Pan et al. 2003). Using the appropriate histochemical technique, these labeled cell bodies can be examined for the presence of various receptors and other markers of neuronal phenotype (Henry and Hargreaves 2007). The labeled pulpal afferent cell bodies can also be isolated, maintained in tissue culture, and harvested for physiological or biochemical analysis (Kim et al. 2011; Chung et al. 2013). Using this approach to isolate pulpal afferent cell bodies, the gene expression of various receptor types and ion channels can be characterized (Chung et al. 2013). Although the electrophysiological responses of isolated cell bodies to various chemicals and thermal stimulation can be recorded, this approach does not allow for the responses of afferents to natural dentin stimuli to be examined (Kim et al. 2011). Because of these limitations, we cannot with certainty assign the various ion channels, transmitters, and receptors observed in pulpal afferents belonging to A- or C-fibers.
The majority of pulpal afferent cell bodies that were isolated in culture were observed to respond to heat and the heat sensation-inducing chemical agent capsaicin. This population of cells also binds the plant isolectin IB4. Electrophysiological recordings revealed that these IB4+ and capsaicin-responsive cells had action potentials having inflections (humps) on their repolarizing phase (Kim et al. 2011). A majority of pulpal afferents also expressed mRNA for TRPV1 (Transient receptor potential cation channel subfamily V member 1) – the heat/capsaicin receptor. This population of neurons probably corresponds to C-fiber nociceptors. In contrast, approximately 20 % of the pulpal afferent cell bodies examined lacked responses to capsaicin, lacked IB4 binding, and had action potentials that did not have a hump on the repolarizing phase. A similar proportion of afferents were TRPV1 mRNA negative (Kim et al. 2011). Some pulpal afferent cell bodies also respond to cold and cold sensation-inducing chemicals, for example, menthol (Park et al. 2006).
These and other results clearly demonstrate that pulpal afferents possess the ability to respond directly to thermal stimulation. Noxious heat stimulates responses in intradental C-fibers (Närhi et al. 1982; Jyväsjärvi and Kniffki 1992). The sharp abrupt pain responses observed when cold stimuli are applied to teeth are possibly a combined effect of cold-induced dentin fluid flow and direct action of cold on receptors on intradental nerve endings (Jyväsjärvi and Kniffki 1987; Park et al. 2006; Chidchuangchai et al. 2007).
Pulpal afferents also possess purinergic receptors (Cook and McCleskey 2002). When pulpal cells are damaged, cytoplasmic ATP is released into the extracellular fluid. ATP is a potent excitant of nociceptors. The phenomenon of ATP release may contribute to nerve activation by hydrodynamic forces since the odontoblast cell bodies are often aspirated into the tubules and destroyed during air blasting or traumatic restorative procedures (Brännström 1963).
Nitric oxide (NO), an oxidative free radical generated by the enzyme nitric oxide synthetase (NOS), has been implicated in inflammatory processes in the dental pulp. Because NO is rapidly destroyed, investigators examined increases in NOS enzyme activity in normal vs. inflamed rat pulps (Law et al. 1999; Fujita et al. 2004). The exact role of NO in DH remains to be discovered.
When viewed within the intact trigeminal ganglion, a slightly different neuronal pattern emerges. Most pulpal afferents were IB4- and contained the vasoactive neuropeptide – calcitonin gene-related peptide (CGRP) (Fried et al. 2011). Capsaicin stimulation of isolated pulp tissue induces the release of CGRP, indicating that this neuronal population possesses the thermally activated TRPV1 receptor and plays a role in controlling the pulpal vasculature (Fehrenbacher et al. 2009). One population of non-neuropeptide-containing afferents was observed to account for few trigeminal ganglion cell bodies, but formed a dense network of nerve fibers in the superficial pulp, indicating a role in dentin sensation (Chung et al. 2012). This population may include the cell bodies that give rise to the intradental A-fibers since this population is known to be relatively unresponsive to capsaicin (Närhi et al. 1992).
Several characteristics of DH are unique. Pain is the only sensory modality experienced when teeth are stimulated. In conducting experiments using electrical stimulation, human subjects report a tingling (rather than painful) sensation when threshold is reached. This observation suggested that not all tooth stimulation resulted in pain (McGrath et al. 1983). Several lines of evidence also indicate the relationship of this “pre-pain” sensation to pain. Temporal (increasing the frequency) and spatial (simultaneous administration of the stimulus to adjacent teeth) summation results in this liminal stimulus being perceived as painful, indicating that the tingling sensation was in fact brief, low-amplitude pain (Närhi et al. 1984; Virtanen et al. 1987).
In many respects, the intradental nociceptors resemble low-threshold mechanoreceptors (LTM) known to mediate non-painful mechanosensation in other parts of the body (Fried et al. 2011). The conduction velocities of some of the pulpal afferents classify these units as Aß-fibers (Närhi et al. 1983). This type of afferent is not normally associated with pain but with non-painful mechanosensation. Examination of pulpal afferent cell bodies in the trigeminal ganglion indicated that many express markers and have cytological feature, for example, cell size that resembles LTM rather than typical nociceptors. The stimuli that trigger pain in sensitive teeth are innocuous in that they do not damage tissue without forceful or repeated application. These features of DH have led investigators to consider the intradental nerves responding to hydrodynamic forces – “low-threshold algoneuron” – as opposed to true nociceptors. The reason that activation of these algoneurons results in pain has to do more with their connections in the central nervous system than to the intrinsic response properties of these cells (Fried et al. 2011).
The transduction of hydrodynamic forces into nerve responses implies that these intradental algoneurons possess receptors that are activated by mechanical forces. The preferential sensitivity of intradental nerves to outward fluid displacements indicates that these mechanoreceptors respond best to a tugging type of force application (Vongsavan and Matthews 2007) (Fig. 2.3).Mechanosensitive ion channels are found widely in nature and underlie nerve responses to several modalities in addition to touch, including osmotic stimulation and sound. In mechanoreceptors, a transmembrane transduction channel is anchored by intracellular and extracellular elements to the cytoskeleton of the receptor or nerve and to an extracellular structure (Fig. 2.5). The transduction channel responds to tension or shear opening or closing, and it is this action that gates ion conductance (Gillespie and Walker 2001; Tsunozaki and Bautista 2009).
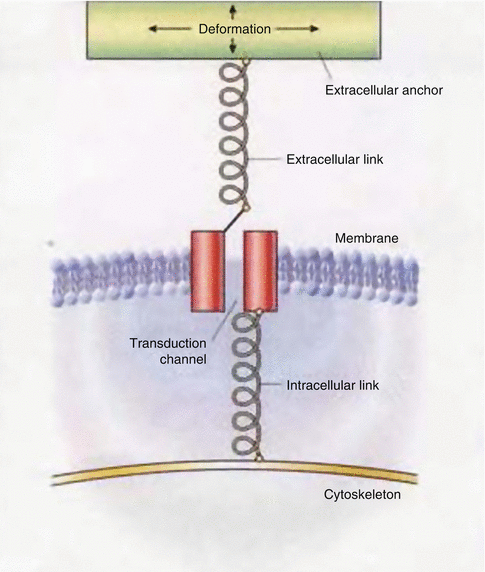
Fig. 2.5
Schematic of a hypothetical mechanosensitive receptor anchored at one end in a nerve membrane and, at the other end, in an extracellular surface. Deformation would open an ion-sensitive channel (Gillespie and Walker (2001) used with permission)
Many ion channels have been proposed as putative mechanoreceptors (Delmas and Coste 2013). Most of these channels also mediate responses to other stimuli such as cold and low pH. It has been difficult to prove a mechanosensory function for several candidate ion channels since genetic knockout animals lacking these channels occasionally appear to retain some mechanically mediated sensations (Kang et al. 2012).
Gene expression of a number of proposed mechanosensory ion channels has been examined in rat trigeminal ganglion cells including labeled pulpal afferents (Hermanstyne et al. 2008). Trigeminal neurons express mRNA for a large number of putative mechanosensory channels, most of which were not expressed in pulpal afferents. Of the mechanosensory channels examined, only one, the acid-sensing ion channel 3 (ASIC3), was expressed in 67 % of the pulpal afferents sampled. In this study, a majority of pulpal afferents also expressed TRPA1, a chemoreceptive channel that may also be activated by cold (McKemy 2005). Based on the observation that TRPA1 is also activated by oxidizing compounds, it has been hypothesized that this receptor is responsible for the pain that accompanies tooth bleaching (Markowitz 2010).
The percentage of neurons expressing ASIC3 was not altered by the induction of pulp inflammation, indicating that ASIC3 is constitutively expressed in the nerve fibers of intact healthy teeth (Hermanstyne et al. 2008). This observation that intradental mechanoreceptors are present in healthy intact teeth was expected since freshly exposed dentin, as would occur in fractured or chipped teeth, is sensitive to both cold air and other stimuli. Physiological experiments examining the nerve responses induced by hydrodynamic stimulation in animals (knockouts) lacking ASIC3 are needed to definitively establish the role of these, and other, ion channels in mediating nerve responses to hydrodynamic stimulation.
In order to activate pain or other forms of conscious sensation, nerves responding to stimuli transmit all-or-none impulses to the central nervous system. In pain fibers, activation of one of the sensory receptors described above depolarizes the nerve cell membrane; if a threshold is reached, voltage-gated ion channels open (Armstrong and Hille 1998), resulting in an action potential that is transmitted to the central nervous system. The pattern and frequency of action potentials encodes information concerning the perceived intensity of the stimulation. Several types of cation-selective ion channels mediate the initiation and termination of the action potential, with voltage-gated sodium channels (VGNa) being responsible for the action potential’s rising phase (Amir et al. 2006). In myelinated pulpal afferents, the distribution of VGNa channels is restricted to the nodes of Ranvier in the myelinated segment of the fiber and spreads out in the peripheral unmyelinated portion (Henry et al. 2012).
Dentin overlying a disrupted odontoblast layer may however be still sensitive. This observation challenges the notion that the odontoblasts are a critical cellular participant in DH (Lilja et al. 1982) (Fig. 2.1). However, odontoblasts do possess an assortment of nerve-like receptors that enable these cells to respond to various chemical, thermal, and mechanical stimuli (Magloire et al. 2010; El Karim et al. 2011; Chung et al. 2013; Tsumura et al. 2013). They may also express various VGNa channels depending on the location within the tooth and the tooth’s developmental stage (Davidson 1994; Allard et al. 2006; Byers and Westenbroek 2011; Ichikawa et al. 2012).
Although specific intercellular communication mechanisms, for example, synaptic structures linking odontoblasts with the intradental nerves, have not been identified (Byers 1984), sensory transduction by odontoblasts may influence the intradental nerves. The odontoblasts control the environment of the intradental nerve endings, determining the dentin fluid’s ionic composition, and they also provide the structural support needed for the mechanosensitive nerve endings to detect dentin fluid flow (Magloire et al. 2010). In response to dentin stimulation, damage to the odontoblasts may also result in prostaglandin formation and, as noted above, release of cytoplasmic ATP or potassium ions into the extracellular fluid (Cook and McCleskey 2002). In experiments where odontoblasts and trigeminal neurons were maintained in culture together, mechanical stimulation of one of the odontoblasts evoked an excitatory calcium conductance in other nearby odontoblasts and neurons. The mechanically stimulated odontoblast released ATP into the extracellular medium through specialized membrane pores. The responses of the neighboring odontoblasts and neurons to this mechanically induced ATP release are mediated by various purinergic receptors (Shibukawa et al. 2014). ATP and other chemical factors released by odontoblasts excite and sensitize the intradental nerves (Utreras et al. 2013). It has been proposed that odontoblasts participate in mediating the response to hydrodynamic dentin stimulation not by conventional synapses but rather by autocrine/paracrine types of signaling (Shibukawa et al. 2014).
It is also possible that the physiological responses of odontoblasts, like those of osteocytes in bone, have little to do with pain. Osteocytes respond to the mechanical stimulation of bone by boosting their metabolic activity and maintaining the mineralization of the bone matrix (Cowin 2007). In the case of odontoblasts, responses to dentin damage and stimulation may be involved in tertiary dentin formation (Byers and Westenbroek 2011). Further research, however, is needed to determine the sensory function of these versatile cells.
The Physiological Basis of Hypersensitive Dentin
Symptoms including a decrease in the pain threshold and an increase in the intensity of pain accompany inflammation (Park et al. 2001). Pulpal inflammation may be responsible for exposed dentin becoming hypersensitive (Pashley 2013). Inflammation-related plasticity has a major impact on the excitability and pharmacological sensitivity of nociceptors during pathological states (Gold and Flake 2005; Henry and Hargreaves 2007; Chung et al. 2013). Pulp inflammation may also be triggered by trauma to the tooth or bacterial invasion of the pulp or dentin.
Oral microorganisms invade and colonize the dentin tubules when caries spreads into dentin (Love et al. 2000). Bacterial products, enzymes, and antigens diffuse through relatively intact dentin layers into the pulp where they can produce an inflammatory response (Brännström 1962; Lundy and Stanley 1969; Goldberg et al. 2008). In acute pulpitis, inflammatory mediators accumulate in the pulp resulting in sensitization and eventual activation of the nociceptors leading to spontaneous pain (Bowles et al. 2003). This process may also be triggered by bacterial products invading the dentin (Warfvinge et al. 1985; Jontell et al. 1998; Fouad 2012), by chronic dentin exposure, or by the trauma of restorative procedures (Olgart et al. 1991; Mjör 2001).
In caries, the type and intensity of the pulp’s inflammatory response are dependent on the depth of bacterial invasion into the dentin (Izumi et al. 1995; Cooper et al. 2011). Considerable variability exists in the symptoms experienced by people with carious teeth. Teeth with deep carious lesions, heavily infected with Lactobacillus species, were observed to be relatively asymptomatic. In contrast, teeth with lesions infected with predominantly anaerobic organisms were more likely to present with thermal sensitivity and other symptoms (Hahn et al. 1993).
Various by-products of bacterial metabolism, for example, amines, are highly excitatory to intradental nerves (Panopoulos 1992). Organic acids, the principal metabolic by-product of sugar fermentation by Lactobacillus and other caries-associated bacteria, have complex effects on pain. Organic acids have an inhibitory effect on intradental nerve activity induced by chemical stimulation (Panopoulos 1992). Acids also enhance dental pain by activating certain receptors and sensitizing other receptors, e.g., TRPV1, to heat stimulation (Goodis et al. 2006). Variations in the bacterial population of a carious lesion may also influence the type of lesion metabolism and the inflammatory response that develops in the pulp, which in turn may determine the degree to which pain sensitization occurs (Hahn and Liewehr 2007).
Even without directly invading the pulp, bacteria can influence the function of the intradental nerves. Lipopolysaccharide (LPS) is an important toxin released by gram-negative anaerobic bacteria that initiate both local pulpal and systemic inflammatory responses (Okiji et al. 1992; Chattipakorn et al. 2002; Bletsa et al. 2006). This toxin can trigger shock and systemic organ damage (Quan et al. 2001). Direct exposure of the pulp to LPS induces severe inflammation and pain facilitation-associated changes in the central nervous system areas that receive input from pulpal nociceptors (Chattipakorn et al. 2005). LPS may diffuse through dentin and facilitate the actions of thermally activated ion channels (Nissan et al. 1995; Chung et al. 2011; Diogenes et al. 2011). These changes would be expected to make teeth very sensitive to hot and cold.
There are many links that exist between the activation of the immune system, inflammation, and pain facilitation (Bletsa et al. 2009; Kress 2010). For example, trigeminal neurons possess toll-like receptors and other components of the innate immune system (Byers et al. 1992; Byers and Närhi 1999; Veerayutthwilai et al. 2007; Horst et al. 2009). These receptors are part of the body’s early warning system that responds to LPS and other bacterial products. This mechanism may help explain the algesic effect of anaerobic bacteria (Wadachi and Hargreaves 2006). Inflammatory mediators appear to induce phosphorylation of TRPV channels, causing them to become more sensitive than normal to thermal stimulation (Jeske et al. 2006).
Inflammation and pain are intimately linked with nociceptor activation being an initiating factor in triggering inflammation and inflammation sensitizing nociceptors (Kim 1990). Painful stimuli, for example, tooth preparation, result in an increase in pulpal blood flow, as well as increases in intrapulpal pressure and outward dentin fluid flow. These effects can also be evoked by activation of the intradental sensory nerves by electrical, chemical, and hydrodynamic stimuli (Pertl et al. 1993; Heyeraas et al. 1994; Olgart 1996; Andrew and Matthews 2002). Stimulus-evoked release of vasoactive peptides, for example, substance P and CGRP from the pulpal terminals of intradental nerve endings, initiates vasodilatation and increases vascular permeability, the early vascular manifestations of inflammation (Kerezoudis et al. 1993; Fehrenbacher et al. 2009). This phenomenon is referred to as “neurogenic inflammation.”
Compared to acute pulpitis, the role of bacteria and pulp inflammation in DH is less clear. Although healthy, freshly exposed dentin can be sensitive and sensitive cervical abrasions are frequently plaque-free (Addy et al. 1987), sensitization of intradental nerve responses can occur due to interactions between the pulp and plaque covering the tooth’s root surface (Tammaro et al. 2000). However, bacteria do not pass through the intact dentin boundary separating the pulp from the tooth surface in sensitive teeth (Michelich et al. 1980). Although dentin tubules observed in SEMs appear to have a diameter of about 1 μm, their functional diameter is only about one-twentieth of that value due to the presence of intratubular material such as collagen fibers, mineral concretions, etc. (Michelich et al. 1978). The functional consequence of this restricted tubule diameter is that dentin does not permit bacteria to reach the pulp. That is, the dentin fluid that reaches the pulp chamber is sterile (Michelich et al. 1980). Dentin acts in a similar manner to a 0.2 μm Millipore filter in keeping oral microorganisms from infecting pulpal soft tissue.
Inflammation can exacerbate DH symptoms by making the intradental nerve endings more responsive to dentin fluid flow. Serotonin (5-HT) is an inflammatory mediator that is released by platelets and mast cells into damaged tissue. It is involved in a number of pain conditions including migraine headache (Sommer 2004). 5-HT application to exposed dentin also lowers the threshold of A-fiber units to hydrodynamic dentin stimulation (Ngassapa et al. 1992). Prior to 5-HT application, these units failed to respond to a weak osmotic stimulant – glucose solution application – to exposed etched dentin. Following application of 5-HT to the dentin, glucose application evoked an excitatory response indicting a lowering of the hydrodynamic threshold. This observation may explain the sensitivity to sweets often experienced during pulpitis. Serotonin sensitizes trigeminal nociceptors to both thermal and chemical stimulation and enhances stimulation-evoked CGRP release (Loyd et al. 2013).
Inflammatory mediators frequently act synergistically in potentiating the response to excitation (Hirafuji and Ogura 1987; Bletsa et al. 2009). For example, bradykinin enhances the capsaicin-evoked CGRP release from intradental nerve endings (Goodis et al. 2000). In those experiments, prostaglandin-E application enhanced this action of bradykinin.
There are also structural changes in the intradental nerves that are a consequence of injury and inflammation (Byers and Närhi 1999). Following traumatic cavity preparation, sprouting of CGRP-containing nerve fiber terminals was observed in the superficial pulp tissue (Taylor et al. 1988). Because of this response, there may be a large increase in the innervation density of the superficial pulp tissue following injury, especially in the normally sparsely innervated cervical and radicular pulp. The timing of sprouting follows the general time course of increases in postoperative DH symptoms encountered following restorative or periodontal procedures. Nerve sprouting is at its maximum 1 week following injury, and then anatomic pattern returns to normal by 3 weeks after injury (Byers 1994; Byers and Närhi 1999; Rodd and Boissonade 2001). Postoperative symptoms tend to be at their worst 1 week after any restorative procedure and generally abate during the following weeks. The nerve sprouting reaction has also been observed in human teeth with deep caries (Rodd and Boissonade 2001).
Injury and inflammation also may initiate the partial demyelination of afferents and changes in the type and spatial pattern of the VGNa channels (Henry et al. 2009). Demyelination can result in abnormal electrical interactions between the axons that allow impulses in one afferent to excite adjacent neurons. These alterations make the afferents more excitable.
The specific type of VGNa channels expressed in nerve fibers may be altered by inflammation and nerve injury (Renton et al. 2005; Henry et al. 2009; Luo et al. 2010). The VGNa channels expressed in neurons exposed to inflammation have altered pharmacological sensitivities compared to neurons innervating healthy tissue (Lai et al. 2004). It is hypothesized that this altered pharmacological susceptibility is responsible for the difficulties encountered obtaining local anesthesia in inflamed teeth (Hargreaves and Keiser 2002).
A mild inflammatory response can be induced in human teeth by preparing dentin cavities and restoring the teeth with defective temporary restorations that allow bacterial leakage. Following 1 week, the inflamed teeth exhibited enhanced pain responses to cold stimulation compared to intact teeth. These changes in thermal sensitivity are not initiated by an increase in dentin permeability (Ajcharanukul et al. 2011). These experiments demonstrate that the pain response in sensitive teeth may be enhanced by inflammation, making the teeth hypersensitive. When sensitive teeth are evaluated clinically, for example, in dental caries, defective restorations and tooth fractures need to be considered in the differential diagnosis and corrected, since in the presence of these conditions, DH is unlikely to resolve with standard topical desensitizing treatments (Fig. 2.6) (Orchardson and Gillam 2006) (see Chaps. 4 and 5).
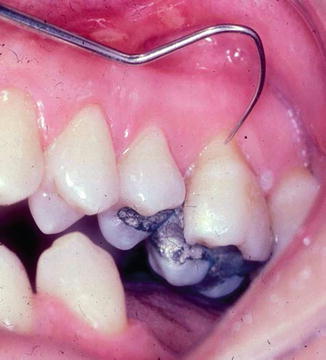
Fig. 2.6
Clinical photograph of a supererupted, sensitive first molar showing the relationship between DH and defective restorations. The tooth was sensitive to cold liquids, air blasts, and probing of the exposed mesial buccal root with a dental explorer as shown. The patient’s sensitivity symptoms were long standing and failed to improve following the application of an oxalate-based topical desensitizer to the exposed root surface. Following the replacement of the existing amalgam restoration, the sensitivity symptoms resolved and the tooth remained asymptomatic and vital. Pulpal inflammation caused by bacterial leakage around the restoration is presumed to have sensitized the intradental nerves to stimuli. In this sensitized state, usually effective topical desensitizing agents fail to provide pain relief. Replacement of the defective restoration allows the inflammatory process and the pain sensitization to resolve (From Markowitz K, unpublished photograph (2000))
Using advanced imaging and electrophysiological methods, it is possible to localize the areas of the brain activated by tooth stimulation (Kubo et al. 2008; Meier et al. 2012). Repeated or intense activation of nociceptors may facilitate pain transmission in the central nervous system. Activation of pulpal afferents by noxious heat stimulation has been reported to induce an enhancement of the responses to those same thermal stimuli by second-order neurons in the trigeminal nuclear complex (Ahn et al. 2012). Intense activation of the intradental nerves or the induction of pulpal inflammation by the administration of noxious chemicals may also induce alterations in gene expression in the trigeminal nuclear complex and enhanced nociception in other facial areas, e.g., the lip (Park et al. 2001; Chattipakorn et al. 2005). The role of central plasticity in DH is however unclear at present. It is possible that nociceptor activation accompanying periodontal procedures that precede episodes of DH sensitizes the central pathways, resulting in more pain being triggered by dentin stimulation.
Oral microorganisms also have profound effects on the permeability of exposed dentin. Despite the capacity for excessive toothbrushing to cause tooth surface loss, plaque control has an important role in preventing DH following periodontal therapy (Wallace and Bissada 1990; Tammaro et al. 2000). Plaque-covered exposed dentin surfaces were observed to have widened tubules compared to surfaces that were kept plaque-free (Suge et al. 2006). The acid liability of smear layers is responsible for the frequently observed lack of DH in patients immediately after root scaling and the onset of DH that appeared 7–14 days following scaling procedures (see Chap. 3). This time delay presumably enables the plaque to form on the root surface and the microorganisms to dissolve the smear layer/smear plug combination (Kerns et al. 1991).
Dentin is a biological composite consisting of mineral and an organic phase that is mostly collagen. In addition to collagen, the organic matrix of dentin also contains several non-collagenous proteins that play an important role in dentin mineralization (Tay and Pashley 2008, 2009; Kim et al. 2010). These proteins are highly acidic and contain calcium-binding phosphate groups that pattern mineral formation (Boskey et al. 1990). The odontoblasts trigger mineralization by secreting these proteins into the predentin at the mineralization front (Veis 1993). As with dentin from which it is derived, dentin smear layers also contain mineral and organic components (Pashley 1992).
The composition of dentin influences its susceptibility to damage and its capacity for repair. In order to degrade dentin, both the organic and inorganic phases of the tissue must be attacked (Love 2002). Plaque-derived acids dissolve the mineral component of the smear layer and underlying dentin; bacterial and host-derived enzymes can attack the organic phase of the dentin (Chaussain-Miller et al. 2006). Host-derived collagen-degrading enzymes, for example, the matrix metalloproteinases, (MMPs) originate in the crevicular fluid, the saliva, and the dentin itself. In dentin, these enzymes can be activated by acid exposure, and these enzymes are believed to contribute to erosive tooth structure loss (Buzalaf et al. 2012). In restored teeth, the long-term action of MMPs and other enzymes may weaken the dentin-restoration bond by degrading resin-infiltrated collagen fibrils (Tjäderhane et al. 2013a). Dentin treatment with chlorhexidine or other cationic compounds preserves bond strength by inhibiting these enzymes (Tjäderhane et al. 2013b).
Reducing DH: Natural Processes and Treatment Effects
Desensitization Treatments Targeting the Dentin Permeability
The reduction of dentin permeability via tubule occlusion is an important strategy for treating sensitive teeth (Pashley 1986; Cummins 2009). Reducing dentin permeability acts not only to attenuate stimulus-evoked dentin fluid flow but also to prevent the activation of the intradental nerves by directly acting physical stimuli and by reducing the inward diffusion of irritating chemical agents (Hirvonen et al. 1984; Rifai et al. 2004; Chidchuangchai et al. 2007). In vitro studies measuring the impact of treatments on the dentin’s permeability and microscopic appearance are used to screen the ability of materials and products to reduce dentin fluid flow (see Chap. 6). Treatments that are effective in vitro studies may be considered to be acceptable candidates for more focused research activities including clinical trials. Due to the steep relationship between tubule radius and flow (flow ∝ r 4
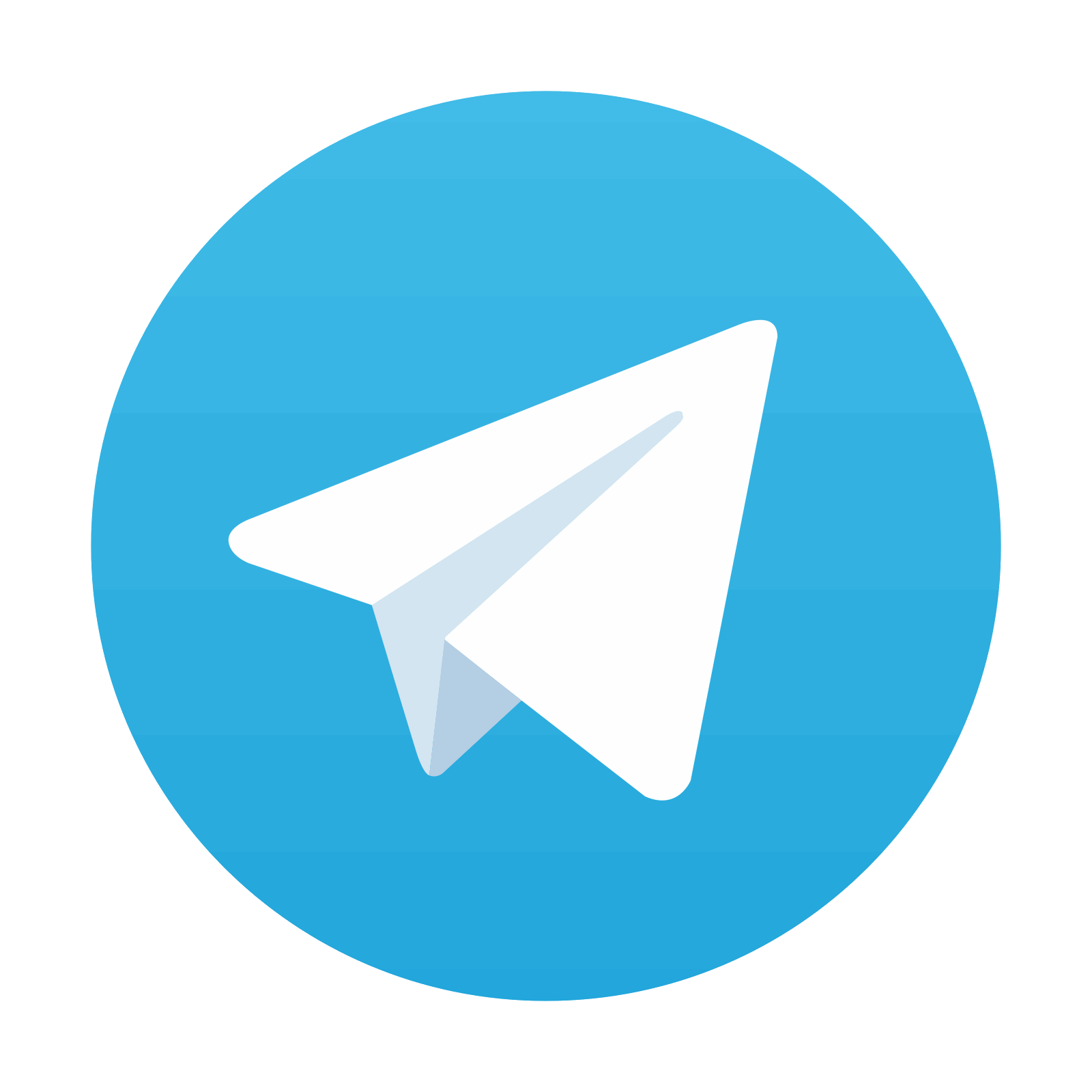
Stay updated, free dental videos. Join our Telegram channel

VIDEdental - Online dental courses
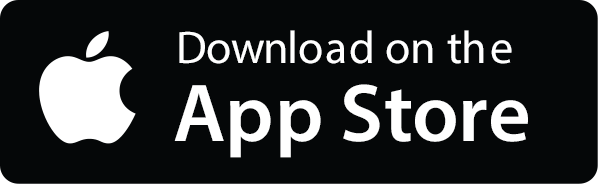
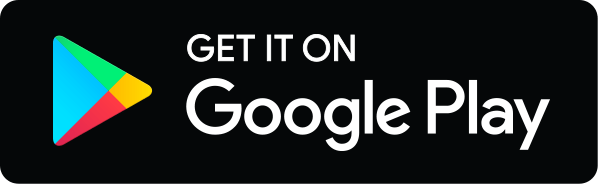