Fig. 11.1
SEM of mid-coronal dentine section before (1) brushing and (2) after brushing with Colgate ProRelief toothpaste
Strontium Salts
Strontium salts have been used in desensitising toothpaste formulations for over 50 years (Markowitz 2009) (see also Chap. 8). Originally strontium chloride was used but more recently strontium acetate at a loading of 8 % w/w has been used. The acetate form has been used to reduce the risk of strontium precipitating as strontium fluoride when a fluoride source is included in the toothpaste formulation. The main mode of action of strontium formulations (acetate/chloride) as discussed in Chap. 8 has been suggested to be by tubular occlusion (Fig. 11.2), although the actual effect attributed to strontium in clinical studies has yet to defined (Rösing et al. 2009). When applied to tooth surfaces, it is believed that an adsorption of strontium ions occurs on the odontoblastic processes or in the dentine tubules, thus blocking the transmission or neural impulses from the dentine surface to the dental pulp. The strontium ion is a normal constituent of tooth structure at trace element concentrations and has a low systemic toxicity. In fact in recent years, strontium salts have been approved for the treatment of osteoporosis where 1–2 g/day is administered. It is thought that this mechanism of strontium binding provides a mechanism to control and manage DH. It is also thought that detergents or surface-wetting agents in the toothpaste enhance the effectiveness of soluble strontium ions by facilitating the penetration of strontium ions into the tooth structure.
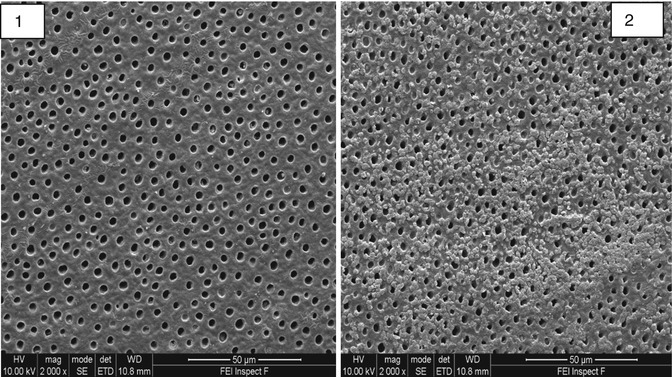
Fig. 11.2
SEM of mid-coronal dentine section (1) before brushing and (2) after brushing with Sensodyne rapid relief toothpaste
Another possibility is that soluble strontium ions may aid apatite formation. The strontium ion is only slightly larger than the calcium ion and strontium can completely replace calcium in the apatite crystal structure. It is thought that the solubility product of a mixed calcium strontium apatite may be lower than that of calcium hydroxyapatite or strontium hydroxyapatite (Pan et al. 2009; Ni et al. 2012).
Consequently soluble forms of strontium in toothpaste may cause apatite to precipitate from saliva. This may be aided by the presence of fluoride. The original strontium chloride toothpaste formulation did not contain fluoride since the fluoride was thought to initiate the precipitation of strontium fluoride (SrF2); however this problem can be reduced by using strontium acetate as the source of strontium. However much of the initial tubule occlusion and reduction in dentine fluid flow may be due not to the strontium ion per se but rather to the presence of small silica particles added as an abrasive that are capable of entering the dentine tubules.
Hydroxyapatite
Hydroxyapatite Ca10(PO4)6(OH)2 is the basis of the mineral phase of enamel and dentine. Enamel contains approximately 98 % by weight apatite whilst dentine contains approximately 60 % by weight. Hydroxyapatite (Ha) has been used as a remineralising additive in toothpaste formulations for many years, particularly in Asia, but only recently been used for the treatment of DH (Park et al. 2005; Kang et al. 2009; Kim et al. 2009; Yuan et al. 2012). Most Ha toothpaste formulations, however, do not contain fluoride, since Ha is promoted as an alternative remineralising additive to fluoride. The apatite crystal structure is shown in Fig. 11.3.
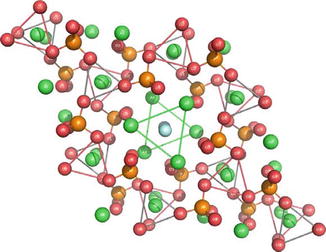
Fig. 11.3
The crystal structure of apatite. The green spheres represent calcium, the orange phosphorus and the red oxygen, whilst the light blue are hydroxyl (Acknowledgement: Figure from Powerpoint presentation by Wolfram Hoeland, Ivoclar Lichtenstein)
The open nature of the crystal lattice makes it relatively easy to substitute other ions in the crystal lattice. Manufacturers in recent years have taken advantage of this feature to produce substituted hydroxyapatites. These include strontium-substituted hydroxyapatite, Ca(10-X) Srx(PO4)6(OH)2; zinc-substituted hydroxyapatite, Ca(10-X)Znx(PO4)6(OH)2; and hydroxycarbonated apatite (HCA) where carbonate ions are substituted for orthophosphate ions.
Some of these toothpaste formulations presented in Table 11.1 are currently promoted as nanohydroxyapatites (nanoHA). In these products the crystallite size is typically 20–100 nm. However the hydroxyapatite particle size is always much larger and consists of many hundreds of aggregated nanocrystals. This is shown schematically in Fig. 11.4 and in the broader diffraction lines (XRD patterns) for Enamel and a Ultradex hydroxyapatite toothpaste (Fig. 11.5). These toothpaste formulations generally contain polymers with carboxylic acid functional groups that are capable of chelating calcium ions in the apatite particles and the apatite in enamel and serve to stick the particles to the tooth surface and prevent them from being washed away by salivary flow. The fine nanoHA crystals dissolve preferentially at the expense of the enamel apatite when the pH is reduced and thus have a protective function. In order to be effective for occluding the dentine tubules, particles <5 μm are required (in the formulation) that are capable of entering the dentine tubules.
Table 11.1
Commercially available hydroxyapatite containing toothpaste formulations
Toothpaste I
|
Company
|
Principal active ingredients
|
---|---|---|
UltraDEX recalcifying and whitening
|
Periproducts
|
Hydroxyapatite
|
Sodium monofluorophosphate
|
||
BioRepair plus
|
BioRepair/ACDOCO
|
Zinc-substituted hydroxyapatite
|
Pepsodent ultra complete sensitive
|
Unilever
|
Hydroxyapatite
|
MentaDent P
|
Unilever
|
Hydroxyapatite
|
Megasonex
|
Goldspire
|
Hydroxyapatite
|
Apagard
|
Sangi Co
|
Hydroxyapatite
|
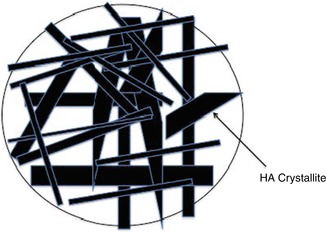
Fig. 11.4
Schematic of aggregated nanocrystals of hydroxyapatite forming a large particle
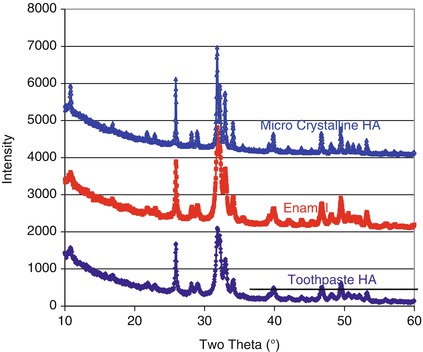
Fig. 11.5
XRD patterns for a microcrystalline hydroxyapatite, Enamel and Ultradex. Note the broader diffraction lines for Enamel and the Ultradex hydroxyapatite indicating nanocrystals. Note the almost identical diffraction patterns for Ultradex and Enamel
Figure 11.6 shows the particle size distribution of a nanoHA toothpaste suitable for treating DH. The shaded area indicates the particles smaller than the dentine tubule size.
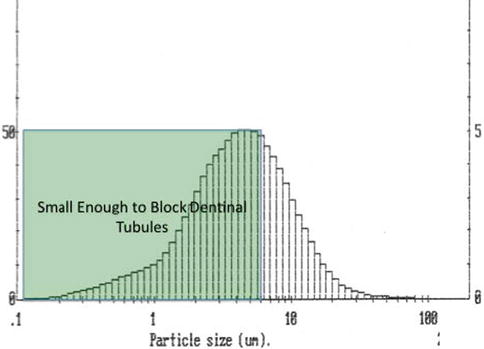
Fig. 11.6
Ultradex hydroxyapatite particle size distribution
The crystallite size for nanoHA can be obtained from Scherrer’s line-broadening analysis of the X-ray diffraction pattern. Crystallites larger than about 200 nm result in broadening of the diffraction lines. Figure 11.5 shows the X-ray diffraction patterns for (a) enamel, (b) a microcrystalline HA and (c) a nanoHA. The broadening of the diffraction lines is clearly seen on comparing the microcrystalline and nanocrystalline HAs. It is worth noting the similarity of the nanoHA diffraction pattern with that of enamel.
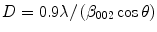
where D is the crystallite size in nm, λ is the wavelength of the incident X-rays (0.154 nm), β 002 is the width at half height of the 002 reflection and cos θ is the cosine of the X-ray incident angle.
Most nanoHA containing toothpaste formulations do not contain a source of fluoride. However incorporation of fluoride is considered to be beneficial. For example following the acid dissolution of the nanoHA particles in the presence of fluoride these particles are reprecipitated as a fluoridated apatite. This reprecipitation process probably aids both remineralisation and tubule occlusion.
Figure 11.7 shows the 19F MAS-NMR spectra of a tooth demineralised at the surface and subsequently treated with a nanoHA toothpaste (with 1,000 ppm fluoride) under acidic conditions. (0.1 M acetic acid pH = 4.5). There is no significant fluoride in the tooth before treating and after demineralisation. However after treating with the Ha toothpaste with fluoride, the 19F spectrum shows a peak at −103 ppm, corresponding to the formation of fluorapatite (FAP).
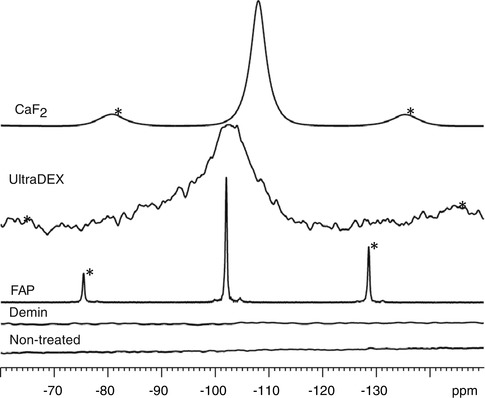
Fig. 11.7
19F MAS-NMR spectra for the enamel samples (non-treated, demineralised and nHA oral rinse treated)
In addition to Ha containing toothpaste formulations, there is also one oral rinse that contains both nanoHA and fluoride. Figure 11.8 shows the tubule occlusion that occurred following a series of demineralisation-remineralisation and oral rinse cycles with a nanoHA mouthrinse. The tubules which were initially open in this etched mid-coronal section are partially occluded by needle-like crystals of fluoridated apatite.
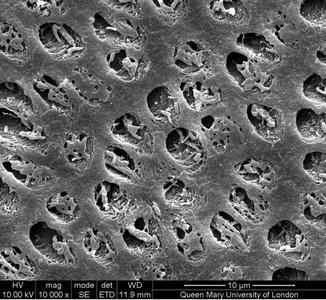
Fig. 11.8
Occlusion of dentine tubules following exposure to a nanoHA oral rinse
Calcium Phospho-silicate (NovaMin®)
NovaMin®, which is based on the 45S5 bioactive glass composition (Table 11.2), has been used successfully in toothpaste formulations as a remineralising additive and for treating DH (see Chap. 8). The 45S5 glass particles dissolve in the mouth releasing Ca2+ and PO4 3− ions and are thought to form hydroxycarbonated apatite (HCA) on the tooth surface.
Table 11.2
45S5 glass composition in mole percent
SiO2
|
P2O5
|
CaO
|
Na2O
|
NC
|
---|---|---|---|---|
46.10
|
2.50
|
26.90
|
24.4
|
2.10
|
It is worth pointing out that the 45S5 composition (see Table 11.2) was designed as a bone substitute and was never designed or intended for use originally as an additive for the treatment of DH in a toothpaste formulation.
In a NovaMin® toothpaste, some of the bioactive glass particles are small enough (<5 μm) to enter the dentine tubules and occlude them (Fig. 11.9). In the presence of saliva, which is a supersaturated calcium phosphate solution, the bioactive glass particles dissolve releasing Ca2+ and PO4 3- ions, which is believed to result in the formation of HCA (Jones 2013). The NovaMin® particle size distribution is broad and shown in Fig. 11.10. Particles smaller than about 5 μm can occlude the dentine tubules, whilst the larger particles in the distribution take much longer to dissolve and provide longer-term release of Ca2+ and PO4 3− ions.
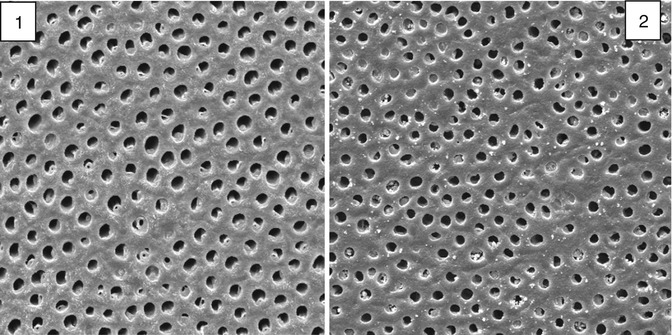
Fig. 11.9
SEM of the open dentine tubules from a mid-coronal section (1) before and (2) after brushing with a bioactive glass toothpaste
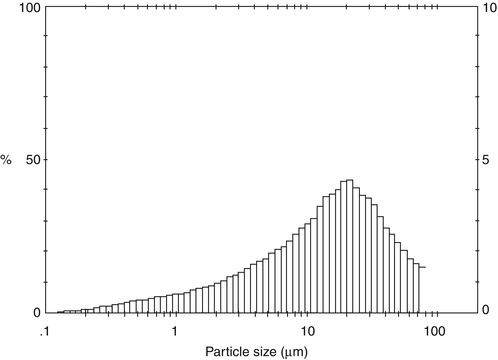
Fig. 11.10
Particle size distribution of NovaMin
NovaMin® toothpaste formulations are similar to the HA toothpaste formulations and contain a carboxyl functional polymer to provide the required viscosity to the toothpaste. However the polymer also plays an important role in chelating calcium ions in apatite and calcium ions in the NovaMin® glass and acts to promote the adhesion of the glass particles to the tooth and prevents the particles from being washed away by salivary flow. In most cases the polymer used is poly(acrylic acid) which is the same polymer used in glass ionomer cements and which enables them to bond to the apatite phase of enamel and dentine.
The NovaMin® toothpaste formulations are glycerol rather than water based, and this is to prevent the glass reacting with water during storage in the toothpaste tube before use. In the early NovaMin® toothpaste formulations, the 45S5 glass reacted with small amounts of water in the glycerol during storage. Approximately 50 % of the phosphate content of the glass can convert to HCA in the toothpaste during storage. 31P solid-state nuclear magnetic resonance spectra for the glass extracted from the toothpaste and the 45S5 glass synthesised in the laboratory are shown in Fig. 11.11.
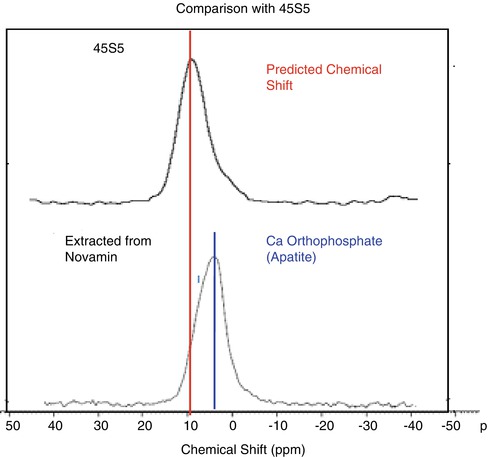
Fig. 11.11
31P ssNMR spectra of 45S% glass and glass extracted from NovaMin® toothpaste
The latest test versions of NovaMin® toothpaste formulations contain a fine particulate silica gel to preferentially absorb any water in the toothpaste, thereby preventing it from reacting with the bioactive glass.
There is increasing evidence that bioactive glasses preferentially form an apatite-like material both on the tooth surface and within the dentine tubules. The apatite-like material grows preferentially on the highly mineralised peritubular dentine on the inside of the dentine tubule. It is postulated that the HCA in the peritubular dentine preferentially nucleates apatite crystals. Bioactive glasses have also been shown to remineralise demineralised dentine (Mneimne et al. 2014; Mneimne 2014) (Figs. 11.12, 11.13 and 11.14).
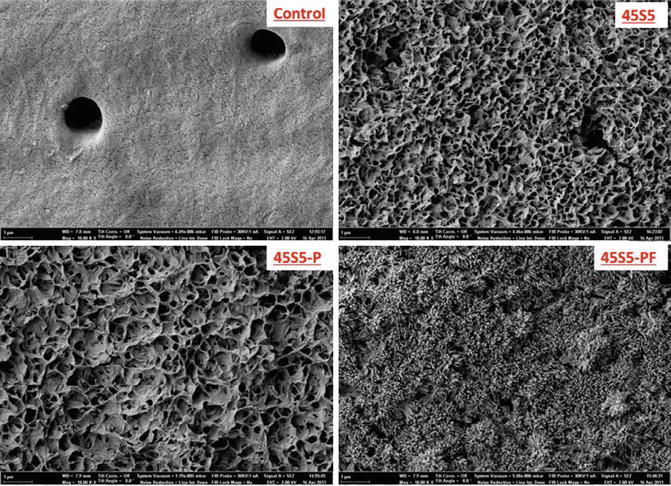
Fig. 11.12
Dentine surfaces treated with different bioactive glass toothpaste formulations. (a) Control, (b) 45S5, (c) 45S5-P, (d) 45S5-PF
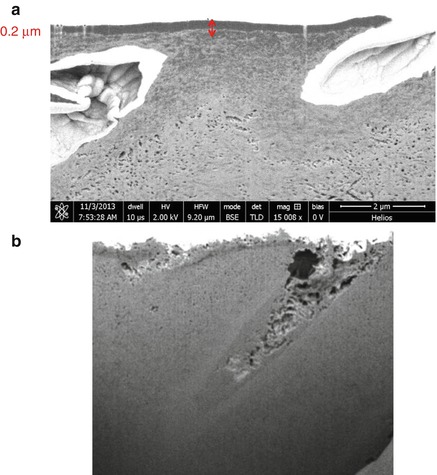
Fig. 11.13
FIB-SEM images of a dentine cross section showing the remineralisation of etched dentine surfaces following application of a bioactive glass and immersion in artificial saliva. (a) Control section, the arrows depict the demineralised layer. (b) Bioactive glass treated section
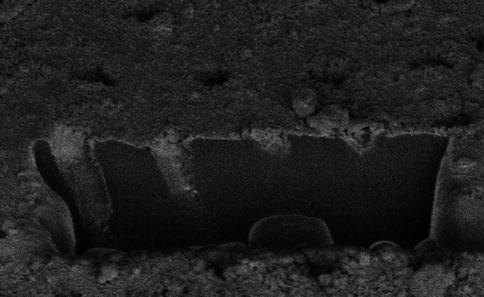
Fig. 11.14
Focused ion beam scanning electron micrograph (FIB-SEM) showing tubule occlusion following the application of a bioactive glass toothpaste
There is also increasing evidence that bioactive glasses dissolve more rapidly and also form apatite more rapidly at acidic pHs associated with caries rather than at pH = 7 or higher. This feature is particularly attractive as bioactive glasses will act to mitigate the pH fall during a caries or acid erosion challenge and will simultaneously release Ca2+ and PO4 3− ions. In this sense bioactive glasses are considered to be smart materials that respond to their environment.
Self-Assembly Peptides
The self-assembly of biological macromolecules is widespread in nature. Examples include the spontaneous folding of proteins and the formation of a double helix from two strands of DNA. Molecular self-assembly enables production on the nanometre scale and is a form of nanotechnology and therefore may have potential for a number of applications in dentistry. Biological mineralisation and especially odontogenesis involves biological self-assembly of proteins prior to mineralisation.
Enamel formation, for example, occurs via the 3D self-assembly of amelogenin which then acts as a nucleating template for apatite crystal formation. There is therefore enormous scope for self-assembling proteins that promote mineralisation.
A self-assembling peptide system was recently introduced as a non-invasive treatment for early-stage dental caries (Kirkham et al. 2007; Brunton et al. 2013). The self-assembling peptides diffuse into the subsurface micropores and form a 3D scaffold, mimicking the proteins’ function during tooth development to support apatite crystallisation and reverse early tooth decay (Fig. 11.15).
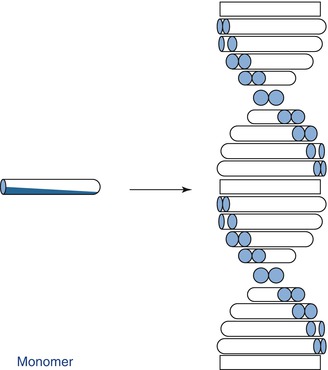
Fig. 11.15
Schematic of the self-assembling process
The peptide P11-4 (Ace-Gln-Gln-Arg-Phe-Glu-Trp-Glu-Phe-Glu-Gln-Gln-NH2) can self-assemble into a matrix, triggering a biomimetic mineralisation and repair mechanism. The glutamic acid residues on the surface act as nucleation sites for apatite formation and result in remineralisation of the lesion. The process of self-assembly is however quite sensitive to pH and ionic strength. Within the carious lesion, the pH and ionic strength favour the self-assembly process.
Recently the first self-assembled peptide product for DH has been launched, called Curodont DeSenz®. This product is thought to use the same peptide as used in the remineralisation and repair of carious lesion, but because the conditions for self-assembly of the peptide at the tooth surface and within the dentine tubules may not be ideal, the current product uses pre-self-assembled particles.
However it may be possible that following treatment with the self-assembled peptide, the majority of tubules are occluded as there was a corresponding 64 % reduction in hydraulic conductance following the application of Curodont DeSenz® on to the dentine section (Fig. 11.16) (Chen et al. 2014).
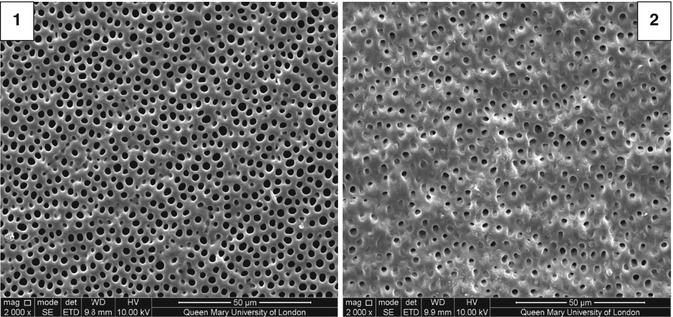
Fig. 11.16
SEM of etched mid-coronal dentine (1) before and (2) after treatment with Curodont DeSenz®
Figure 11.17 shows a SEM cross section as opposed to a top-down view of a self-assembled peptide particle occluding a dentine tubule following immersion in artificial saliva. The particle contains numerous plate-like crystals with a Ca:P ratio close to the apatite stoichiometry which indicates the self-assembled peptide particle is providing a surface for the preferential nucleation of apatite.
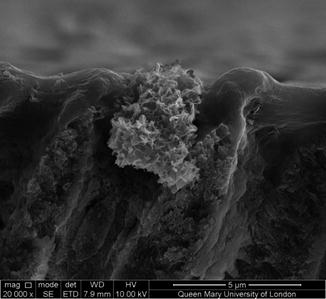
Fig. 11.17
SEM showing a remineralised Curodont DeSenz® particle occluding a dentine tubule following immersion in artificial saliva
Future Innovations
There are relative few options for improving on the existing desensitising products, for example, arginine, strontium and Ha toothpaste formulations, as these are fairly well-established technologies. However there is considerable potential for improving on the existing calcium phospho-silicate or bioactive glass toothpaste formulations. This is largely a result of the bioactive glass that is currently used having been developed and optimised as a bone substitute.
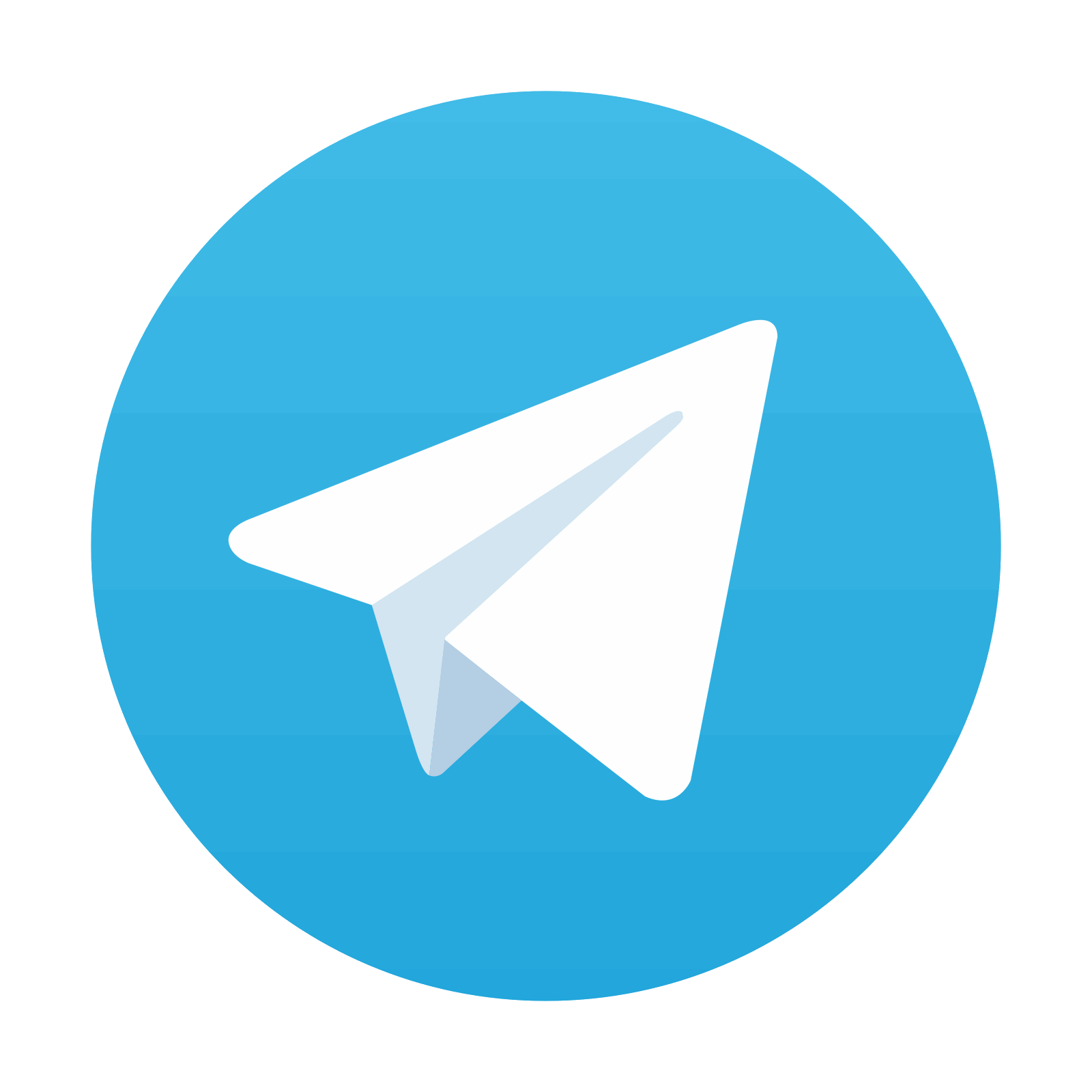
Stay updated, free dental videos. Join our Telegram channel

VIDEdental - Online dental courses
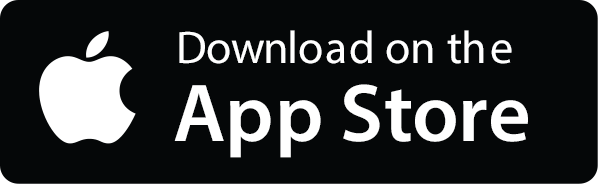
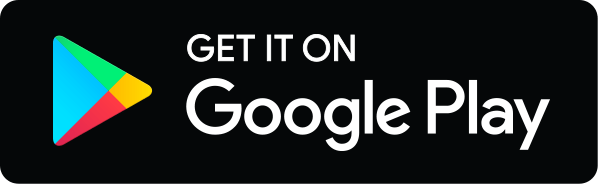