Abstract
Introduction
The aim was to determine the magnitude of ceramic resin-strengthening with resin-based materials with varying flexural moduli using a regression technique to assess the theoretical strengthening at a ‘zero’ resin-coating thickness. The hypothesis tested was that experimentally, increasing resin flexural modulus results in increased resin-strengthening observed at a theoretical ‘zero’ resin-coating thickness.
Method
Vitadur Alpha dentin porcelain disk ( n = 250) were condensed, fired, alumina particle air abraded and randomly assigned into ten groups. Groups were resin-coated at 50, 100 and 150 μm with Venus Flow, Rely-X Veneer and Clearfil AP-X before biaxial flexure testing at 24 h and the stress at failure calculated using a multilayer analysis. An analytical methodological approach was undertaken to predict the biaxial flexure stresses under boundary conditions that reflected the experimental test and a finite element model was used to verify the analytical prediction.
Results
The magnitude of resin-reinforcement was significantly influenced by resin-coating type ( P < 0.001) and resin-coating thickness ( P = 0.013), however, a significant interaction was observed between resin-coating type and thickness ( P = 0.048). Linear regression identified a 17, 38 and 47% biaxial flexure strength contribution when Venus Flow, Rely-X Veneer and Clearfil AP-X were used, respectively. The finite element model determined the maximum principal stress was within 3.3% of the predicted analytical solution.
Significance
Experimentally, the flexural modulus and thickness of resin-based material used to cement DBC or PLV restorations have a significant impact on the magnitude of resin-strengthening observed. However, for resin-based materials with different flexural moduli the variability in the relationships between thickness and observed increases in biaxial flexure strength of the ceramic requires careful characterization to optimize clinical performance.
1
Introduction
In 1999 and now more than a decade ago, the 14 year findings of a comprehensive prospective clinical study into the variables determining the survival of 1444 restorations fabricated from a single glass-ceramic substrate were reported . The authors demonstrated the glass-ceramic restorations were significantly more resistant to fracture when adhesively bonded to the prepared tooth structure using resin-based materials compared with when luted with acid–base cements . The clinical outcome data supported the proposals made previously by Marquis and Nathanson that adhesive cementation strengthens feldspathic porcelain and dental glass-ceramic restorations . Although the two independent investigators provided different hypotheses for the exact resin-strengthening mechanism, both authors agreed the interaction of the resin-based material with the critical flaws on the ceramic surface conferred the magnitude of observed strengthening . Over the last decade there has been a significant increase in the use of porcelain laminate veneer (PLV) and dentin bonded crown (DBC) restorations which combine conservative tooth preparation ideals with adhesive bonding strategies to esthetic but relatively weak dental ceramic materials . The clinical success of these restorations fabricated from feldspathic porcelains or glass-ceramics is remarkable given that failure rates in the anterior dentition do not differ dramatically from restorations constructed from ceramic substrates with up to ten-fold increased flexural strengths .
In attempts to identify operative strategies available to practitioners’ to maximize the magnitude of resin-strengthening, investigators have demonstrated the reinforcement achieved was significantly modified by the choice of resin-based material. Resin-based materials have evolved considerably since Bowens’ resin-based composite (RBC) formulation and in recent years non-methacrylate monomeric resins and filler technologies encompassing nanotechnology have been developed. Manufacturers have increased the filler volume fraction, thereby increasing the mechanical properties (flexural strength, flexural modulus and wear resistance) since in terms of mechanical properties the filler is dominant over the resin . Today, the performance of RBCs which has been reported in a prospective clinical study to be superior to dental amalgam restorations, albeit when placed by an experienced operator . However, while the promotion of superior mechanical properties appears to be the goal in RBC development for restorative applications, the improvement of resin-based luting materials appears to be governed by optimization of the resin viscosity and resin film thickness in efforts to simplify restoration seating and improve marginal adaptation. As a result, the resin-based materials routinely employed for luting feldspathic porcelain and dental glass-ceramic restorations (PLVs or DBCs) are limited to flowable and luting resin formulations which have significantly reduced filler loading, lower flexural moduli and increased viscoelasticity when compared with commercial RBC restorative formulations .
The magnitude of ceramic strengthening following resin-coating has been demonstrated to be a function of the resin’s ability to interpenetrate surface defects forming a ‘hybrid-layer’ . The reinforcement achievable is therefore dependent on the ceramic surface texture, but is also influenced by intrinsic and mechanical properties of the resin-based material and the ultimate resin-coating thickness . A near-linear relationship between the flexural modulus of the resin-coating and ceramic reinforcement has been reported . Consideration of the transient and residual stresses in a dental ceramic before and after resin-coating has shown that resin-based materials exert a compressive stress state across the entire ceramic surface defect integral due to the volumetric shrinkage associated with polymerization . Although the shrinkage stress generated during polymerization of resin-based materials is a function of flexural modulus, the relationship is complicated by differing shrinkage volumes in materials with similar flexural moduli due to their different formulations. The near-linear relationship between resin flexural modulus and ceramic reinforcement is therefore under scrutiny. In a simple bilayered structure such as a dental ceramic disk coated on one surface with resin, the relative stiffness of the variable layer (the resin), alters the position of the neutral plane of the specimen in bending and therefore alters the stress gradient between the neutral plane and the ceramic surface. As the critical flaw is likely to vary in its pre-loaded length, location and orientation the gradient of an increasing tensile stress within the loaded structure is an important consideration alongside the terminal stress value at failure . To isolate the absolute reinforcement conferred by resin-based materials with different flexural moduli, the creation of a thin resin-coating (<10 μm) is required, however, this is impracticable . As an alternative the authors have developed a regression technique to approximate the effect of a theoretical ‘zero’ resin-coating thickness .
The aim of the current study was to determine the magnitude of ceramic resin-strengthening with resin-based materials with varying flexural moduli using a regression technique to assess the theoretical strengthening at a ‘zero’ resin-coating thickness. The hypothesis tested was that experimentally, increasing resin flexural modulus results in increased resin-strengthening observed at a theoretical ‘zero’ resin-coating thickness.
2
Materials and methods
2.1
Experimental approach
Two hundred and fifty nominally identical Vitadur Alpha dentin porcelain disk-shaped specimens (13.0 (0.2) mm diameter, 1.90 (0.06) mm thickness) were formed using the condensation method outlined by Fleming et al. . An optimum powder to liquid slurry consistency was obtained by manipulating 1.05 g of powder (Shade A2, Lot #62167, Vita, Bad Säckingen, Germany) with 0.3 mL of Vita Modeling Fluid (Lot #14209R, Vita, Bad Säckingen, Germany) in a porcelain mixing tray. The porcelain slurry was condensed into a Perspex mold (15.0 mm diameter, 2.0 mm thickness) secured to a glass slide using clamps. The Perspex mold was vibrated for 90 s, on an ultrasonic condenser (Ceramosonic II, Shofu Dental Corporation, Kyoto, Japan), to displace the excess modeling fluid, which was manually blotted dry with tissue paper. The excess condensed porcelain slurry was removed from the top of the specimen using a razor blade and this was termed the leveled surface .
The specimen was removed from the mold and the leveled surface placed in contact with a silicon nitride firing slab. The specimens were fired in a vacuum furnace (Vita Vacumat 40T, Bad Säckingen, Germany) in accordance with manufacturer’s guidelines. The specimens were pre-dried at 600 °C for 6 min, fired under vacuum from 600 to 960 °C at a heating rate of 60 °C/min, air fired at 960 °C for a further 60 s and cooled at 20 °C/min to room temperature. After cooling, the leveled surface was marked and the specimens were stored at 22 °C in a desiccator to prevent hydrolysis . The leveled surface of each specimen was alumina particle air abraded (Eco Dry Oxide System, Dentalfarm, Torino, Italy) with 50 μm alumina particles, at a distance of 2 cm from the nozzle of the abrader under a pressure of 2 bar . The alumina particle air abrasion technique was performed orthogonal to the disk surface in a smooth linear sweeping motion for a maximum of 5 s to prevent excessive localized abrasion of the disk surface. The specimens were randomly assigned into ten groups ( n = 25) and the central thicknesses were measured using a screw gauge micrometer accurate to 10 μm (Mitutoyo Corp., Tokyo, Japan). One specimen group (Group A) was stored in a desiccator prior to testing and analysis while the remaining nine groups (Groups B–J) were prepared for resin-coating.
A bespoke Perspex jig used a fixed displacement offset, governed by feeler gauges at the three prescribed thicknesses to enable the application of discrete resin-coating thicknesses (50, 100 and 150 μm) to the alumina particle air abraded disk-surface. The Perspex base plate was covered with a polyester film (Mylar™, DuPont Teijinn Films, Middlesbrough, UK) to prevent resin-adhesion to the base plate. Prior to resin-coating, the alumina particle air abraded surface was silanated with a 3-methacryloxypropyl-trimethoxysilane (Rely-X™ Ceramic Primer, 3M ESPE, St. Paul, MN, USA) and allowed to air dry, in accordance with the manufacturers recommendations. Three different resins were used to coat the disk-shaped specimens. Specimens were resin-coated with Venus Flow (Heraeus, Hanau, Germany) at resin-coating thicknesses of 50 ± 25 μm (Group B), 100 ± 25 μm (Group C) and 150 ± 25 μm (Group D), Rely-X Veneer (3M ESPE, St. Paul, MN, USA) at 50 ± 25 μm (Group E), 100 ± 25 μm (Group F) and 150 ± 25 μm (Group G) and Clearfil AP-X (Kuraray, Kurashiki, Japan) at 50 ± 25 μm (Group H), 100 ± 25 μm (Group I) and 150 ± 25 μm (Group J). For the Clearfil AP-X resin-coated specimens (Groups H–J), the resin was first placed on a glass-slide and heated to 50 °C prior to resin-coating to reduce viscosity of the resin thereby enabling the achievement of the required discrete resin thicknesses. Following resin-coating with Clearfil AP-X, the specimens were allowed to cool to ambient room temperature prior to light irradiation through the Perspex base plate. The specimens were light irradiated using an Optilux 501 (SDS Kerr, Danbury, CT, USA) through the Perspex base plate for 20 s with a 13 mm light tip diameter and the output intensity at the resin surface was 380 ± 21 mW/cm 2 – determined using a radiometer.
The failure loads (N) of the control (Group A) and the resin-coated (Groups B–J) porcelain disk-shaped specimens were determined using a ball-on-ring test configuration in a universal testing machine (Instron Model 5565, Instron Ltd., High Wycombe, UK) employing a 2 kN load cell following 24 h dry storage. The specimens were tested with the alumina particle air abraded surface (Group A) or the resin-coated surface (Groups B–J) in contact with a flat sheet of latex (0.2 mm thickness) which covered the 10 mm diameter knife-edge ring support. The latex compensated for variations in geometric flatness and allowed small lateral displacements, thereby, minimizing friction and shear . The disk-shaped specimens were centrally loaded with a 4 mm diameter ball indenter at 1 mm/min. The central thickness of each fracture fragment was measured and the average resin thickness was determined for each disk.
The stress at failure was calculated using a multilayer analysis . The position of the neutral plane ( t n ) was determined using the flexural modulus of the dentin porcelain ( E 1 ) – 64 GPa , the resin flexural moduli ( E 2 – assessed experimentally below) and the thicknesses of the porcelain and resin layers ( t 1 and t 2 , respectively) from Eq. (1) .
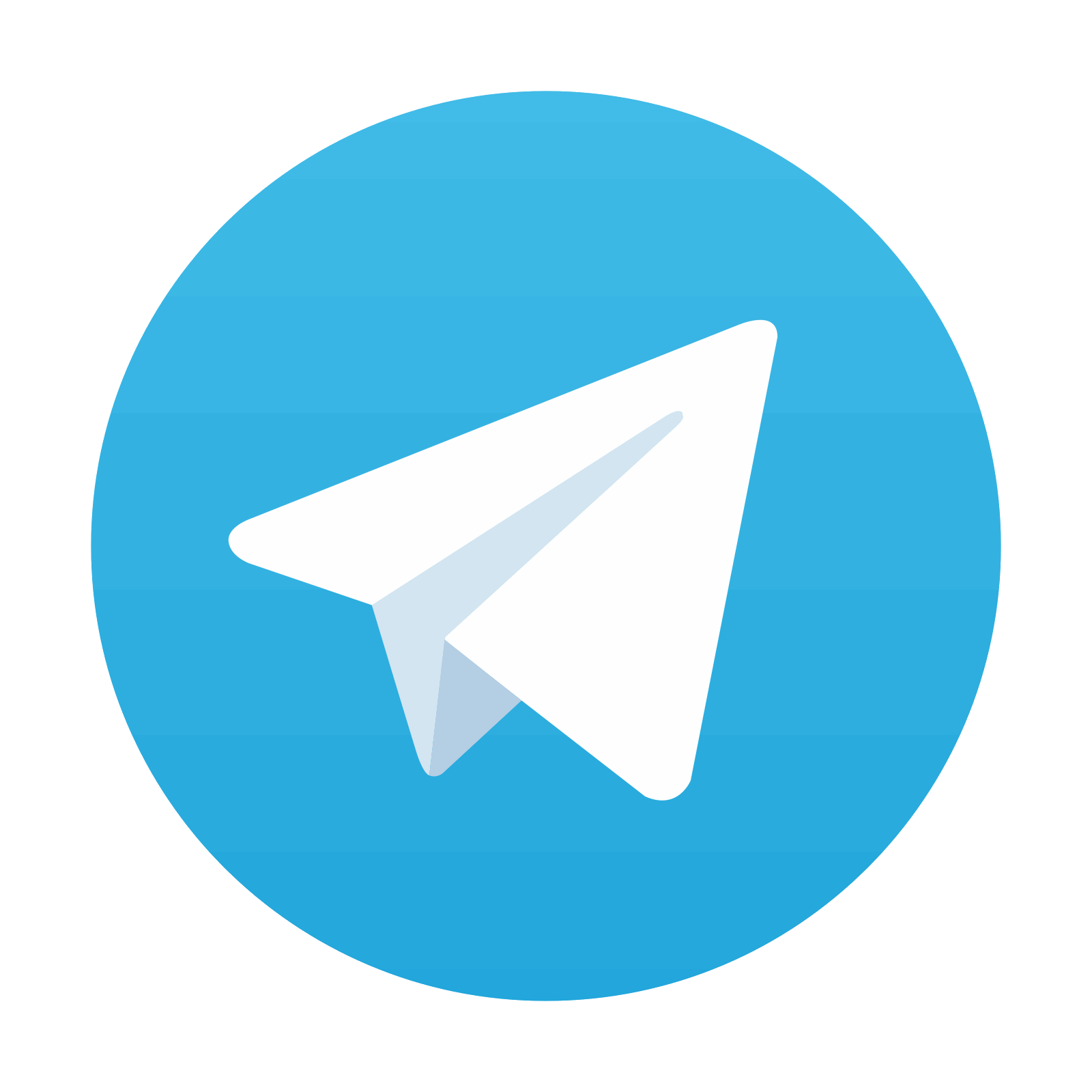
Stay updated, free dental videos. Join our Telegram channel

VIDEdental - Online dental courses
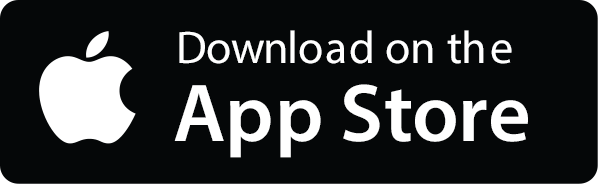
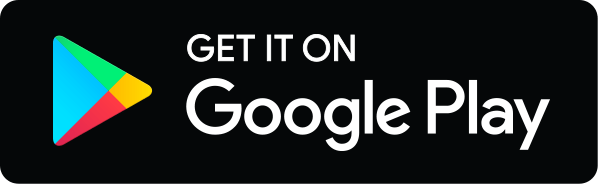