Abstract
Objective
The purpose of this investigation was to compare stresses within bonded porcelain and composite resin ultra-thin occlusal veneers to restore advanced erosive lesions.
Materials and methods
A sound maxillary molar was digitized with a micro-CT scanner. The 2D image data were converted in a 3D model using an interactive medical image processing software (Mimics). Standard triangle language files (STL files) of enamel and dentin surfaces were then exported to the software 3-matic to execute design and meshing operations. Solid 3-dimensional (3-D) models acquired in a finite element software (Marc/Mentat) were subjected to nonlinear contact analysis to simulate occlusal loading at 200N and 800N. Values of maximum principal stress and ultimate tensile strength were used to calculate the risk of fracture and for validation with existing experimental data.
Results
There were marked differences in stress distributions both at 200N (maximum peak values of 21.59, 28.63, 31.04 MPa) and 800N (96.16, 115.73, 134.90 MPa) for all restorative materials (MZ100, Empress CAD and e.max CAD, respectively). High tensile stresses (measured in the central groove) were found at 800N with the ceramic occlusal veneers showing occlusal stress peaks 17–29% higher than composite resin. The estimated risk of fracture was decreased for ultrathin composite resin occlusal veneers, which correlated with the existing validation data.
Significance
Ultra-thin composite resin (MZ100) and lithium disilicate (e.max CAD) occlusal veneers represent a conservative alternative to traditional onlays and complete coverage crowns for the treatment of severe erosive lesions in the posterior dentition.
1
Introduction
Enamel, as the outer barrier, is designed to resist the wide array of aggressions from the oral environment (mechanical, chemical, biological and thermal) for one’s entire life. It acts as a shield protecting the softer vital underlying dentin against wear . The reduction of the enamel’s thickness along its life is a biological condition resulting from the aging process . However, the premature and accelerated loss of enamel by gastro-esophageal reflux disease (GERD) or bulimia nervosa may happen in adolescence or even in childhood, with destructive consequences .
Ultra-thin bonded posterior occlusal veneers have been demonstrated as a conservative alternative to traditional onlays and complete coverage crowns for the treatment of severe erosive lesions . Evidence and common sense seem to indicate that severely eroded teeth require nonretentive preparations and stronger materials. The combination of CAD/CAM technologies and a state of the art bonding protocol (such as immediate dentin sealing) seems to be the right approach for this paradigm shift .
Only bonded ceramics and composite resins can potentially conform with the aforementioned requirements for utmost tissue conservation and esthetics . The development of ceramics that are stronger (e.g. lithium disilicate glass ceramic) but still etchable and machinable has extended the spectrum of indications for bonded ceramic restorations. The performance of composite resins have also improved considerably during the last decade , through superior bond between the different constituting phases (enabling appropriate stress transfer) and various post-cure treatments .
It is noteworthy that knowledge of stress distribution under cyclical activity in a highly complex and anisotropic structure such as a restored tooth is quite important, particularly for the clinical prediction of novel restorative procedures. Experimental load-to-failure should be accompanied by non-destructive approaches such as fatigue tests and finite element (FE) method.
Therefore, the aim of the present study was to assess the influence of CAD/CAM restorative material (ceramic vs. composite resin) on the stress distribution of ultra-thin veneers. The null hypothesis was that there would be no influence of material selection on the stress distribution of ultra-thin (0.6 mm thick) occlusal veneers.
2
Materials and methods
A 3-D FE model of an extracted human maxillary first molar was generated in three steps according to a previous and validated protocol . A raw micro-CT set of slices was provided by Digisens (Ferney-Voltaire, France) with a voxel dimension of 13.67 microns.
Second, an interactive medical image processing software (Mimics 9.0; Materialise, Leuven, Belgium) allowed identifying the different hard tissues visible on the scans. Mimics features extended visualization and segmentation functions based on image density thresholding. Each 3-D object is created by growing a threshold region on the entire stack of scans. Each resulting mask (enamel, dentin) is then converted into a 3-D file (STL, bilinear and interplane interpolation algorithm) using Mimics STL + module. The pulp chamber and root canals were generated as an empty space (no elastic modulus) in the dentin.
Because of the aspect ratio and connectivity of the triangles in the native STLs, these files are improper for use in FEA. Reducing the amount of triangles and simultaneously improving the quality of the triangles while maintaining the geometry is automatically achieved with the Remesh module included with Mimics.
Third, an advanced STL design and meshing software (3-matic 4.2, Materialise, Leuven Belgium) was used to generate additional intersection parts simulating the occlusal veneer preparation and establish perfect congruence of the interfacial mesh between the different objects (enamel, dentin, restorative material, cylindrical fixation base simulating the bone). The following sequence was applied, starting by the merging of all 4 parts into a single STL file, called assembly. Self-intersecting curves were then created and used to split all triangles at the intersections within the assembly. Using a section view to look inside the assembly, all unwanted surfaces were deleted. The definitive assembly was then remeshed using the Remesh module of 3-matic. Self-intersecting curves were maintained and the tolerance variation from the original data was specified (quality of triangles does not mean tolerance variation from the original data). As in Mimics Remesh, the quality is defined as a measure of triangle height/base ratio (defined at 0.3) so that the file can be imported in the finite element analysis software package without generating errors. Finally, the remeshed assembly was split again by indicating the proper surfaces constituting each part (occlusal veneer, enamel, dentin and bone), separating them and copying them as a new and definitive STL part.
The exact design and dimensions of the occlusal veneer reproduced an existing experiment , which was used in the validation process of the FEA model (see Section 3 ). The assembly consists of a prepared tooth (occlusal reduction by the complete removal of the occlusal enamel) and a 0.6 mm-thick onlay restoration ( Fig. 1 A and B)

Fourth, the definitive STL files of all parts were then imported in a finite element analysis software package (MSC.Marc/MSC.Mentat; MSC Software, Santa Ana, Calif) for the generation of a volumetric mesh (total 131,853 elements/26,770 nodes) and attribution of material properties according to existing data ( Table 1 ). Automatic mesh generation using a tetrahedral mesher (tetrahedron elements with pyramid-like shape and 4 nodal points) is ideally achieved using the triangulated files generated in 3-matic.
Source | Elastic modulus (GPa) | Poisson’s ratio |
---|---|---|
Enamel | 84.1 a | 0.30 b |
Dentin | 18.6 c | 0.3 d |
Base (cortical bone) | 14.7 e | 0.30 d |
MZ100 | 16 f | 0.24 f |
Empress CAD | 62 g | 0.21 h |
e.max CAD | 95 g | 0.30 i |
g Data from manufacturer of Empress CAD and e.max CAD (Ivoclar Vivadent, Schaan, Liechtenstein).
The nodes at the bottom surface of the fixation base were assigned fixed zero-displacement in the 3 spatial dimensions. To simulate usage of adhesive luting cements, the tooth and restorative materials were considered to be bonded. A uniform ramp loading was applied to the mesiobuccal, mesiolingual and distobuccal cusps (tripod contact) through a rigid body, that is a 7 mm-diameter ball positioned as close as possible to the tooth ( Fig. 1 B and C). The tooth was defined as a deformable contact body. Contact between these bodies was determined automatically by the FEA simulation during the static mechanical loadcase (no inertia effects). A motion was applied to the rigid ball along the z -axis until the target load was obtained, measured by 200N or 800N on the ball. The stress and strain distributions were solved using the MSC.Marc solver (MSC Software). These specific boundary conditions, load protocol and configuration were selected because they reproduce a previous experiment .
2
Materials and methods
A 3-D FE model of an extracted human maxillary first molar was generated in three steps according to a previous and validated protocol . A raw micro-CT set of slices was provided by Digisens (Ferney-Voltaire, France) with a voxel dimension of 13.67 microns.
Second, an interactive medical image processing software (Mimics 9.0; Materialise, Leuven, Belgium) allowed identifying the different hard tissues visible on the scans. Mimics features extended visualization and segmentation functions based on image density thresholding. Each 3-D object is created by growing a threshold region on the entire stack of scans. Each resulting mask (enamel, dentin) is then converted into a 3-D file (STL, bilinear and interplane interpolation algorithm) using Mimics STL + module. The pulp chamber and root canals were generated as an empty space (no elastic modulus) in the dentin.
Because of the aspect ratio and connectivity of the triangles in the native STLs, these files are improper for use in FEA. Reducing the amount of triangles and simultaneously improving the quality of the triangles while maintaining the geometry is automatically achieved with the Remesh module included with Mimics.
Third, an advanced STL design and meshing software (3-matic 4.2, Materialise, Leuven Belgium) was used to generate additional intersection parts simulating the occlusal veneer preparation and establish perfect congruence of the interfacial mesh between the different objects (enamel, dentin, restorative material, cylindrical fixation base simulating the bone). The following sequence was applied, starting by the merging of all 4 parts into a single STL file, called assembly. Self-intersecting curves were then created and used to split all triangles at the intersections within the assembly. Using a section view to look inside the assembly, all unwanted surfaces were deleted. The definitive assembly was then remeshed using the Remesh module of 3-matic. Self-intersecting curves were maintained and the tolerance variation from the original data was specified (quality of triangles does not mean tolerance variation from the original data). As in Mimics Remesh, the quality is defined as a measure of triangle height/base ratio (defined at 0.3) so that the file can be imported in the finite element analysis software package without generating errors. Finally, the remeshed assembly was split again by indicating the proper surfaces constituting each part (occlusal veneer, enamel, dentin and bone), separating them and copying them as a new and definitive STL part.
The exact design and dimensions of the occlusal veneer reproduced an existing experiment , which was used in the validation process of the FEA model (see Section 3 ). The assembly consists of a prepared tooth (occlusal reduction by the complete removal of the occlusal enamel) and a 0.6 mm-thick onlay restoration ( Fig. 1 A and B)
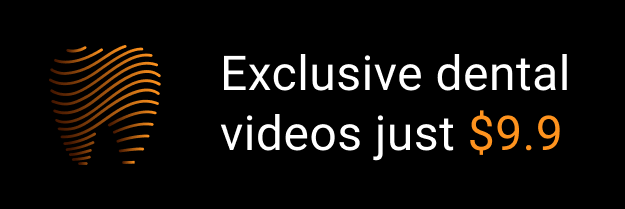