Abstract
Objective
The objective was to examine the effect of a solvent dimethyl sulfoxide (DMSO) on resin–dentin bond durability, as well as potential functional mechanisms behind the effect.
Methods
Microtensile bond strength (μTBS) was evaluated in extracted human teeth in two separate experiments. Dentin specimens were acid-etched and assigned to pre-treatment with 0.5 mM (0.004%) DMSO as additional primer for 30 s and to controls with water pre-treatment. Two-step etch-and-rinse adhesive (Scotchbond 1XT, 3M ESPE) was applied and resin composite build-ups were created. Specimens were immediately tested for μTBS or stored in artificial saliva for 6 and 12 months prior to testing. Additional immediate and 6-month specimens were examined for interfacial nanoleakage analysis under SEM. Matrix metalloproteinase (MMP) inhibition by DMSO was examined with gelatin zymography. Demineralized dentin disks were incubated in 100% DMSO to observe the optical clearing effect.
Results
The use of 0.5 mM DMSO had no effect on immediate bond strength or nanoleakage. In controls, μTBS decreased significantly after storage, but increased significantly in DMSO-treated group. The control group had significantly lower μTBS than DMSO-group after 6 and 12 months. DMSO also eliminated the increase in nanoleakage seen in controls. 5% and higher DMSO concentrations significantly inhibited the gelatinases. DMSO induced optical clearing effect demonstrating collagen dissociation.
Significance
DMSO as a solvent may be useful in improving the preservation of long-term dentin–adhesive bond strength. The effect may relate to dentinal enzyme inhibition or improved wetting of collagen by adhesives. The collagen dissociation required much higher DMSO concentrations than the 0.5 mM DMSO used for bonding.
1
Introduction
The durability of the dentin bond strength with resin composites is one of the main research topics in adhesive dentistry today . Several approaches to improve long-term bonding have been developed and tested. These include inhibition of dentinal endogenous proteases, matrix metalloproteinases (MMPs) and cysteine cathepsins, thought to be responsible for degrading the hybrid layer collagenous matrix, and improved penetration and impregnation of the adhesive monomers into ethanol-saturated exposed dentin matrix . However, despite promising results in in vitro research, clinically feasible and commonly accepted methods to improve long-term bonding are still lacking.
Dimethyl sulfoxide (DMSO: (CH 3 ) 2 SO), is polar aprotic solvent dissolving both polar and non-polar compounds. It is a polyfunctional molecule with a highly polar S O group and two hydrophobic CH 3 groups ( Fig. 1 ). Its amphiphilic nature, small size and dipolar aprotic nature make it perhaps the best currently known penetration enhancer for medical purposes . It is fully miscible in all solvents used in adhesive dentistry, and its ability to penetrate biological surfaces is at least partially caused by dissociation of extracellular matrix collagen . These effects may be related either to DMSO’s ability to compete with water molecules in interpeptide hydrogen bonding in collagen matrix or the ability to change the collagen interfibril spacing on a submicrometer scale . DMSO has also been used to solvate several dental monomers and other adhesive components in toxicological testing of adhesive components , demonstrating its ability to solvate commonly used adhesive constituents.

The tissue penetration capacity and excellent solvent properties make DMSO an attractive solvent candidate also for the dental adhesives. The aim of this study was to measure the effect of dentin pre-treatment with DMSO on immediate and long term adhesive bond strength and nanoleakage of commercially available simplified etch-and-rinse adhesive. The hypotheses set were that, pretreatment of dentin with DMSO would not affect dentin bond strength or nanoleakage. Since previous studies have indicated that DMSO may inhibit MMP activity , and that MMP inhibition improves long term bond strength , the effect of DMSO on MMP activity was evaluated with gelatin zymography. The effect of DMSO on demineralized dentin collagen dissociation was also examined.
2
Materials and methods
Intact human third molars extracted as part of the normal clinical treatment were used for the study with the patients’ consent and approval from the Ethical Committee, Faculty of Medicine, University of Oulu (register #23-2003). Teeth were stored in 0.2% sodium azide at 4 °C until used. All adhesives and the resin composites were unaltered commercially available products from 3M ESPE (Seefeld, Germany).
2.1
Microtensile bond strength evaluation
Microtensile bond strength was evaluated in two separate experiments, performed in two different laboratories, following the same bonding procedures and using same materials. The teeth were sectioned under water cooling coronally to remove occlusal enamel and to expose flat dentin surface, and at the dentin–enamel junction. Exposed dentin surface was ground with 180-grit abrasive paper to create uniform smear layer, and the teeth were randomly divided into two groups. In the control specimens, the adhesives were used as recommended by the manufacturer. Briefly, the dentin surface was first acid-etched with 37% phosphoric acid for 15 s, rinsed, and gently dried with compressed air to achieve slightly dry surface. Then water was scrubbed into the surface with cotton pellet for 30 s and gently dried, leaving the surface slightly moist, and 1-step etch-and-rinse adhesive Scotchbond 1XT was used as per manufacturer’s recommendations, and polymerized with 20 s blue light irradiation (Elipar Freelight 2, 3M ESPE, output 1200 mW/cm 2 ). In experimental group, instead of water the surface was scrubbed with 0.5 mM (0.004%) DMSO in water after acid-etching and drying with all other steps similar to those with controls. A 4-mm resin composite restoration (Filtek Supreme XT) was then built on top of the adhesive-treated dentin with 1-mm increments that were separately polymerized with a blue light.
The teeth were stored in artificial saliva for 24 h to allow water sorption and postoperative polymerization of the adhesive and resin composite to take place. Then the teeth were longitudinally sectioned across the bonded interface in 0.9 mm slabs perpendicular to the pulpal wall with a diamond saw. The slabs, in turn, were cut into 0.9 mm sticks to create 0.9 mm × 0.9 mm × 8 mm beams . The beams were randomly divided into three groups and placed in 37 °C in artificial saliva. Bond strength was tested within a week from making the filling, using Bisco Micro Tensile Tester (Bisco, Schaumburg, IL, USA) in the Experiment 1, and universal testing machine (3342; Instron Co., Canton, MA, USA) in the Experiment 2. Approximately one-third of the beams were tested immediately, while the rest were stored for 6 or 12 months to evaluate the durability of bond strength. In bond strength testing, each specimen was individually fixed to a testing jig with cyanoacrylate glue and subjected to tensile load at a crosshead speed of 0.5 mm/min until failure. The force required to fracture the sample and the exact dimensions of the fracture site were recorded with a digital caliber to the nearest 0.01 mm, and tensile bond strength (in MPa) was calculated for each beam. The mean bond strength of all the beams was averaged to represent an average bond strength of each tooth. Five teeth were in each subgroup. Repeated-measures analysis of variance (ANOVA) with related-samples pairwise t -tests were used to compare the bond strengths between the time points within the groups, and independent-samples t -test to compare the effects of treatments within each time point. Because the number of specimens and bond strengths achieved differed marked between the experiments, z -transform was calculated to allow the comparison of the laboratories, using the formula z = ( x − mean)/SD, and independent-samples t -test and 95% confidence intervals of the differences were calculated for each time point separately.
2.2
Interfacial nanoleakage evaluation
Three beams, prepared for the microtensile testing in Experiment 2, from DMSO and control group were used for nanoleakage evaluation immediately and after 6-month storage, using method described by Klein-Júnior et al. . The beams were placed in the 50 mass% ammoniacal AgNO 3 in darkness for 24 h, rinsed thoroughly in distilled water, and immersed in photo developing solution for 8 h under a fluorescent light to reduce silver ions into metallic silver grains within voids along the bonded interface. The specimens were embedded in epoxy resin and polished with SiC paper and diamond paste, ultrasonically cleaned, air-dried, mounted on stubs, and coated with carbon air-dried for the analysis with scanning electron microscope (SEM) operated in the backscattered electron mode under low vacuum (JSM 5600LV, JEOL, Tokyo, Japan). Randomly selected four areas of each sample’s hybrid layer were photographed. From the photographs, the total length of the dentin–adhesive interface and the length of interface with silver particles (demonstrating nanoleakage) were measured with ImageJ program (ImageJ 1.42q: NIH). The percentage of the total hybrid layer length with nanoleakage was calculated. Repeated-measures analysis of variance (ANOVA) with related-samples pairwise t -tests were used to compare the bond strengths between the time points within the groups, and independent-samples t -test to compare the effects of treatments within each time point.
2.3
Zymographic analysis
The effect of DMSO on MMP activities was evaluated following the protocol described previously . Briefly, gelatin zymography was performed in 10% SDS-PAGE containing 1 mg/mL labeled gelatin in a usual fashion (Invitrogen, Carlsbad, CA, USA). Both non-activated and 4-aminophenylmercuric acetate (APMA)-activated samples of in-house purified human gelatinases MMP-2 and -9 were loaded into the gels. After the electrophoresis, SDS was removed by 2.5% Triton X-100 to renature the gelatinases. Gels were then incubated overnight at 37 °C in 50 mM Tris–HCl zymography activation buffer (5 mM CaCl 2 , 1 μM ZnCl 2 , 0.02% NaN 3 , pH 7.5) with 0%, 0.1%, 2.5%, 5%, 10% or 20% of DMSO to observe the effect of DMSO concentration on the MMP activity. The degradation of gelatin was visualized under UV light. Gels were also stained with 0.5% Coomassie Blue R-250. The intensities of the bands were quantified by ScionImage software (Scion Corporation, Frederick, MA, USA). Three separate gels were incubated with each DMSO concentration, and relative amounts of gelatinase inhibition against 0% DMSO were calculated. Because the zymography data did not follow the normal distribution, Kruskal–Wallis test with Mann–Whitney U -test were used to analyze the differences in band intensities.
2.4
DMSO optical clearance of demineralized dentin
Dissociative effect of DMSO on demineralized dentin collagen was examined as described in skin by Bui et al. . Three completely demineralized 1 mm thick human dentin disks were incubated in 100% DMSO for 30 min, and optical clearance was observed against ruler. Then the disks were incubated in water for additional 15 min and optical clearance was rechecked.
2
Materials and methods
Intact human third molars extracted as part of the normal clinical treatment were used for the study with the patients’ consent and approval from the Ethical Committee, Faculty of Medicine, University of Oulu (register #23-2003). Teeth were stored in 0.2% sodium azide at 4 °C until used. All adhesives and the resin composites were unaltered commercially available products from 3M ESPE (Seefeld, Germany).
2.1
Microtensile bond strength evaluation
Microtensile bond strength was evaluated in two separate experiments, performed in two different laboratories, following the same bonding procedures and using same materials. The teeth were sectioned under water cooling coronally to remove occlusal enamel and to expose flat dentin surface, and at the dentin–enamel junction. Exposed dentin surface was ground with 180-grit abrasive paper to create uniform smear layer, and the teeth were randomly divided into two groups. In the control specimens, the adhesives were used as recommended by the manufacturer. Briefly, the dentin surface was first acid-etched with 37% phosphoric acid for 15 s, rinsed, and gently dried with compressed air to achieve slightly dry surface. Then water was scrubbed into the surface with cotton pellet for 30 s and gently dried, leaving the surface slightly moist, and 1-step etch-and-rinse adhesive Scotchbond 1XT was used as per manufacturer’s recommendations, and polymerized with 20 s blue light irradiation (Elipar Freelight 2, 3M ESPE, output 1200 mW/cm 2 ). In experimental group, instead of water the surface was scrubbed with 0.5 mM (0.004%) DMSO in water after acid-etching and drying with all other steps similar to those with controls. A 4-mm resin composite restoration (Filtek Supreme XT) was then built on top of the adhesive-treated dentin with 1-mm increments that were separately polymerized with a blue light.
The teeth were stored in artificial saliva for 24 h to allow water sorption and postoperative polymerization of the adhesive and resin composite to take place. Then the teeth were longitudinally sectioned across the bonded interface in 0.9 mm slabs perpendicular to the pulpal wall with a diamond saw. The slabs, in turn, were cut into 0.9 mm sticks to create 0.9 mm × 0.9 mm × 8 mm beams . The beams were randomly divided into three groups and placed in 37 °C in artificial saliva. Bond strength was tested within a week from making the filling, using Bisco Micro Tensile Tester (Bisco, Schaumburg, IL, USA) in the Experiment 1, and universal testing machine (3342; Instron Co., Canton, MA, USA) in the Experiment 2. Approximately one-third of the beams were tested immediately, while the rest were stored for 6 or 12 months to evaluate the durability of bond strength. In bond strength testing, each specimen was individually fixed to a testing jig with cyanoacrylate glue and subjected to tensile load at a crosshead speed of 0.5 mm/min until failure. The force required to fracture the sample and the exact dimensions of the fracture site were recorded with a digital caliber to the nearest 0.01 mm, and tensile bond strength (in MPa) was calculated for each beam. The mean bond strength of all the beams was averaged to represent an average bond strength of each tooth. Five teeth were in each subgroup. Repeated-measures analysis of variance (ANOVA) with related-samples pairwise t -tests were used to compare the bond strengths between the time points within the groups, and independent-samples t -test to compare the effects of treatments within each time point. Because the number of specimens and bond strengths achieved differed marked between the experiments, z -transform was calculated to allow the comparison of the laboratories, using the formula z = ( x − mean)/SD, and independent-samples t -test and 95% confidence intervals of the differences were calculated for each time point separately.
2.2
Interfacial nanoleakage evaluation
Three beams, prepared for the microtensile testing in Experiment 2, from DMSO and control group were used for nanoleakage evaluation immediately and after 6-month storage, using method described by Klein-Júnior et al. . The beams were placed in the 50 mass% ammoniacal AgNO 3 in darkness for 24 h, rinsed thoroughly in distilled water, and immersed in photo developing solution for 8 h under a fluorescent light to reduce silver ions into metallic silver grains within voids along the bonded interface. The specimens were embedded in epoxy resin and polished with SiC paper and diamond paste, ultrasonically cleaned, air-dried, mounted on stubs, and coated with carbon air-dried for the analysis with scanning electron microscope (SEM) operated in the backscattered electron mode under low vacuum (JSM 5600LV, JEOL, Tokyo, Japan). Randomly selected four areas of each sample’s hybrid layer were photographed. From the photographs, the total length of the dentin–adhesive interface and the length of interface with silver particles (demonstrating nanoleakage) were measured with ImageJ program (ImageJ 1.42q: NIH). The percentage of the total hybrid layer length with nanoleakage was calculated. Repeated-measures analysis of variance (ANOVA) with related-samples pairwise t -tests were used to compare the bond strengths between the time points within the groups, and independent-samples t -test to compare the effects of treatments within each time point.
2.3
Zymographic analysis
The effect of DMSO on MMP activities was evaluated following the protocol described previously . Briefly, gelatin zymography was performed in 10% SDS-PAGE containing 1 mg/mL labeled gelatin in a usual fashion (Invitrogen, Carlsbad, CA, USA). Both non-activated and 4-aminophenylmercuric acetate (APMA)-activated samples of in-house purified human gelatinases MMP-2 and -9 were loaded into the gels. After the electrophoresis, SDS was removed by 2.5% Triton X-100 to renature the gelatinases. Gels were then incubated overnight at 37 °C in 50 mM Tris–HCl zymography activation buffer (5 mM CaCl 2 , 1 μM ZnCl 2 , 0.02% NaN 3 , pH 7.5) with 0%, 0.1%, 2.5%, 5%, 10% or 20% of DMSO to observe the effect of DMSO concentration on the MMP activity. The degradation of gelatin was visualized under UV light. Gels were also stained with 0.5% Coomassie Blue R-250. The intensities of the bands were quantified by ScionImage software (Scion Corporation, Frederick, MA, USA). Three separate gels were incubated with each DMSO concentration, and relative amounts of gelatinase inhibition against 0% DMSO were calculated. Because the zymography data did not follow the normal distribution, Kruskal–Wallis test with Mann–Whitney U -test were used to analyze the differences in band intensities.
2.4
DMSO optical clearance of demineralized dentin
Dissociative effect of DMSO on demineralized dentin collagen was examined as described in skin by Bui et al. . Three completely demineralized 1 mm thick human dentin disks were incubated in 100% DMSO for 30 min, and optical clearance was observed against ruler. Then the disks were incubated in water for additional 15 min and optical clearance was rechecked.
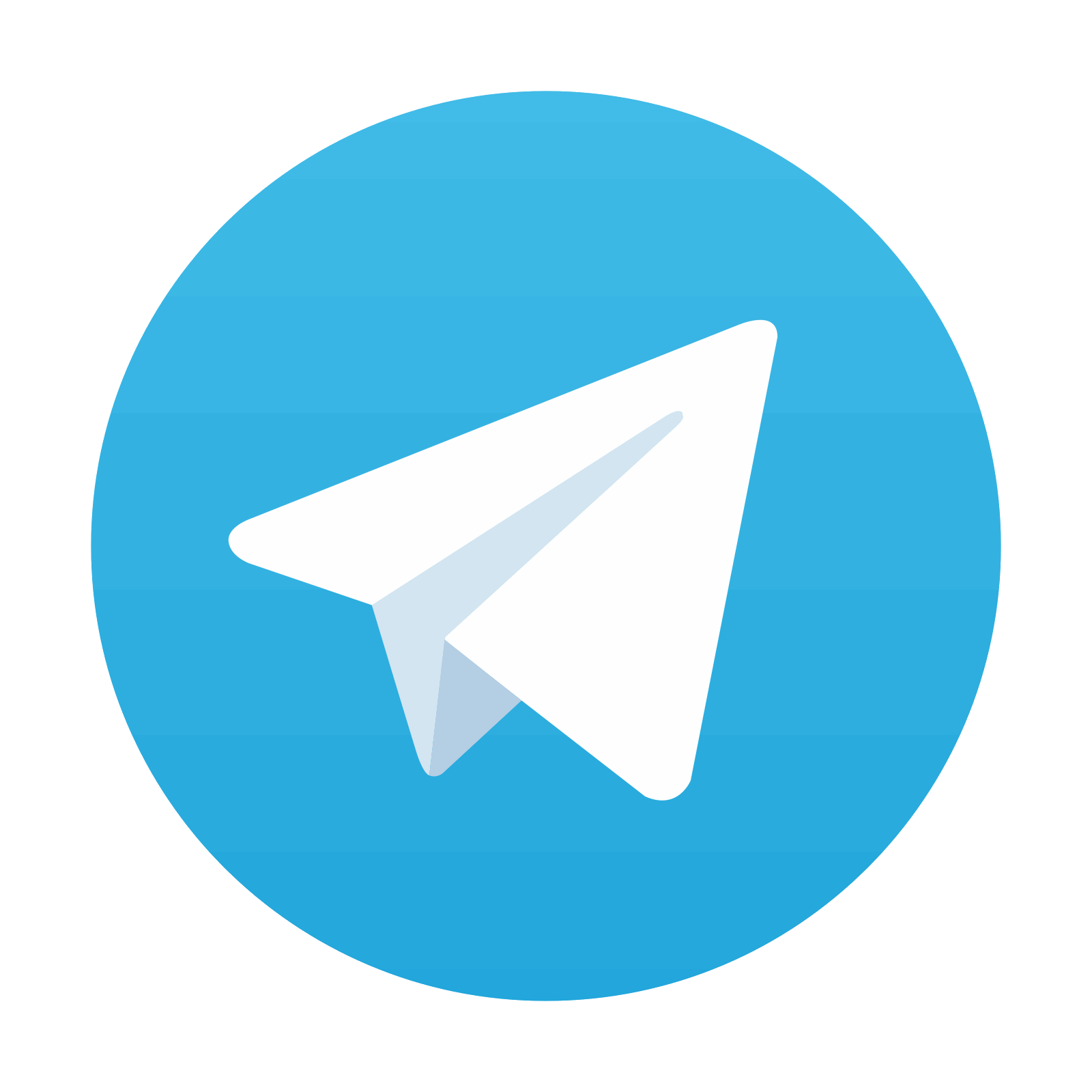
Stay updated, free dental videos. Join our Telegram channel

VIDEdental - Online dental courses
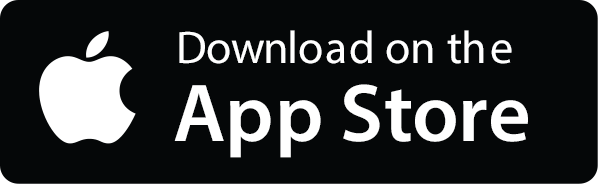
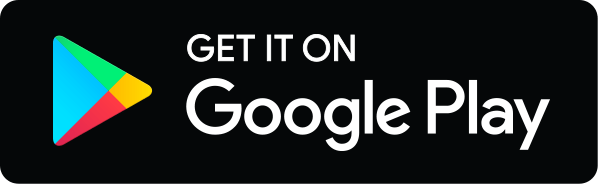