Abstract
Objectives
The initial adhesion of microorganisms to clinically used dental biomaterials is influenced by physico-chemical parameters like hydrophobicity and pre-adsorption of salivary proteins. Here, polymethyl methacrylate (PMMA), polyethylene (PE), polytetrafluoroethylene (PTFE), silicone (Mucopren soft), silorane-based (Filtek Silorane) and methacrylate-based (Tetric EvoCeram) dental composites, a conventional glassionomer cement as well as cobalt–chromium–molybdenum (Co28Cr6Mo) and titanium (Ti6Al4V) were tested for adsorption of salivary proteins and adhesion of Streptococcus gordonii DL1.
Methods
Wettability of material surfaces precoated with salivary proteins or left in phosphate-buffered saline was determined by the measurement of water contact angles. Amounts of adsorbed proteins were determined directly on material surfaces after biotinylation of amino groups and detection by horseradish peroxidase-conjugated avidin-D. The same technique was used to analyze for the binding of biotinylated bacteria to material surfaces.
Results
The highest amount of proteins (0.18 μg/cm 2 ) adsorbed to hydrophobic PTFE samples, and the lowest amount (0.025 μg/cm 2 ) was detected on silicone. The highest number of S. gordonii (3.2 × 10 4 CFU/mm 2 ) adhered to the hydrophilic glassionomer cement surface coated with salivary proteins, and the lowest number (4 × 10 3 CFU/mm 2 ) was found on the hydrophobic silorane-based composite. Hydrophobicity of pure material surfaces and the number of attached microorganisms were weakly negatively correlated. No such correlation between hydrophobicity and the number of bacteria was detected when surfaces were coated with salivary proteins.
Significance
Functional groups added by the adsorption of specific salivary proteins to material surfaces are more relevant for initial bacterial adhesion than hydrophobicity as a physical property.
1
Introduction
The development of biofilms or dental plaque in the oral cavity on dental hard and soft tissues, as well as on a multitude of clinically used biomaterials for restorative purposes, is a main cause of common dental diseases including caries, gingivitis, periodontitis, and peri-implantitis . It is a unique feature in the oral cavity that aggregates of microorganisms form on natural and artificial surfaces which are persistently covered with salivary proteins . Clinically applicable dental materials such as alloys, metals, composites, acrylic resin dentures, glass ionomer cements or silicones cover a wide range of distinct surface properties including topography, element composition, functionality, electrical charge, or wettability. These various properties often combine in an individual material resulting in a highly complex set of parameters which determine biofilm formation.
The formation of biofilms begins with the initial reversible adhesion of microorganisms from saliva, which is driven by the surface energies of material surfaces and organisms in a particular environment. The relevance and effectiveness of Van der Waals forces and Coulomb forces as well as hydrophobic, electrostatic, or Lewis acid–base interactions depends on the range of interactions. Among these non-covalent forces, hydrophobic interactions in the medium range of the nanometer scale are considered most relevant in water, and electrostatic interactions are principal forces in the short range as well . These forces also influence the adsorption of salivary constituents and carbohydrates to form an acquired pellicle on oral surfaces, a proteinaceous layer which contains enzymes, glycoproteins and other macromolecules . It was reported that hydrophobic surfaces may attract higher amounts of salivary proteins than hydrophilic materials, suggesting a high affinity of salivary proteins to polymer materials . Yet, the parameters influencing the adsorption of protein to surfaces appear to be more complex since contradictory results have also been previously reported . The highest amounts of proteins were found to adsorb to hydrophilic model surfaces and the lowest amounts were detected on hydrophilic PEG- and hydrophobic CF 3 -modified surfaces. It was also suggested that surface-dependent adsorption of salivary proteins might vary widely with the adsorption of particular proteins . Most relevant, depending on the different physicochemical properties of various surfaces, the composition of a thin layer of proteins differentially adsorbed from complex fluids like saliva is most likely more important than the absolute amount of proteins adsorbed to a specific biomaterial surface. There is experimental evidence which suggests that specific ligands of salivary proteins support the initial adhesion of bacteria to surfaces. For instance, two dominant and unique groups of salivary proteins, proline-rich proteins (PRPs) and salivary mucins, are compounds of the acquired pellicle and provide adhesion sites for receptor proteins of oral bacteria . Nevertheless, it has been previously suggested that the adsorption of proteins from saliva onto surfaces leveled out differences in surface properties of different materials depending on the thickness, composition, and conformation of proteins in the adsorbed layer . Most noteworthy, it was observed that, depending on the protein species, wettability of hydrophilic surfaces with water decreased after adsorption of proteins, whereas water contact angles decreased on hydrophobic surfaces .
It was the aim of the present investigation to gain more insight into the parameters responsible for the initial adhesion of an oral microorganism to a variety of clinically relevant dental restorative materials. The formation of an artificial model biofilm was beyond the scope of this investigation. We hypothesized that hydrophilicity/hydrophobicity of a dental material surface influences the initial adhesion of an early colonizer like Streptococcus gordonii . We first analyzed the water contact angle of pure and protein-coated surfaces of various dental restorative materials, amounts of human salivary proteins adsorbed to material surfaces, and the initial adhesion of S. gordonii. Finally, these data were used to calculate the correlation between hydrophilicity/hydrophobicity of a material surface, protein adsorption, and bacterial adhesion.
2
Materials and methods
2.1
Materials and chemicals
Polymethylmethacrylate (PMMA, Palapress) was obtained from Heraeus Kulzer GmbH (Hanau, Germany), polyethylene (PE; ultra high molecular weight polyethylene), cobalt–chromium–molybdenum (Co28Cr6Mo), and titanium (Ti6Al4V) were purchased from Zimmer GmbH (Winterthur, Switzerland). The silicone Mucopren soft came from Kettenbach GmbH (Eschenburg, Germany), a silorane-based dental composite (Filtek Silorane) and a glassionomer cement (Ketac Molar) was obtained from 3M ESPE AG (Seefeld, Germany), the methacrylate-based composite material Tetric EvoCeram came from Ivoclar Vivadent (Schaan, Lichtenstein), and polytetrafluoroethylene (PTFE) specimens were provided by the Institute of Chemistry (University of Regensburg). Caso-bouillon was obtained from VWR International (Darmstadt, Germany). Biotinamidohexanoic acid 3-sulfo-N-hydroxysuccinimide ester (sulfo-NHS-LC-biotin) was obtained from Pierce (Rockford, IL USA) and fluorescein isothiocyanate (FITC)-labeled avidin-D was bought from Vector Laboratories Inc. (Burlingame, CA, USA). Tween 20 was purchased from Sigma–Aldrich (Taufkirchen, Germany). All other chemicals and organic solvents were purchased in the highest available purity from VWR.
2.2
Sample preparation
Polytetrafluoroethylene (PTFE), polyethylene (PE), titanium (Ti6Al4V) and cobalt–chromium–molybdenum (Co28Cr6Mo) samples were cut from rods with a diameter of 11.3 mm and a thickness set to 2 mm. Titanium and CoCrMo sample surfaces were cleaned by sonication in acetone, toluol, acetone, ethanol, and water for 3 min each; PTFE and PE were sonicated in ethanol and water only. Teflon rings with an inner diameter of 11.3 mm and 2 mm in height were used to prepare specimens between two glass plates to obtain flat surfaces of PMMA, silicone (Mucopren soft), silorane-based and methacrylate-based dental composite as well as a glass ionomer cement (Ketac Molar). Surface roughness expressed as median peak-to-valley values ( R a ) were found between 0.04 μm (PMMA) and 0.53 μm (PE) using a perthometer (S6P, Mahr GmbH, Göttingen, Germany). PMMA, silicone (Mucopren soft), silorane- and methacrylate-based dental composite materials were prepared according to the manufacturer’s instructions. These specimens were stored in demineralized water at 25 °C for seven days. Glass ionomer cement (Ketac Molar) specimens were first allowed to set for seven minutes when prepared between glass plates, and then stored for twenty-four hours in a humidified chamber.
2.3
Salivary protein preadsorption to material surfaces
Unstimulated human whole saliva collected from two healthy males (24 and 29 years) was pooled and sterile filtered using membrane filters of 0.2 μm pore size (Sartorius, Germany). The protein content was determined by the bicinchoninic acid protein assay (BCA, Pierce, Rockford, IL USA) and samples were then stored at −20 °C until use. Material specimens were separately placed into each well of a 24-well plate filled with 1.2 ml saliva (0.6 mg/ml protein) and incubated for 30 min at room temperature. For measuring wettability of the materials surfaces as well as bacterial adhesion (see Sections 2.4 and 2.6 ) after protein adsorption, the specimens were washed three times for 10 min with phosphate-buffered saline (PBS) to remove excess protein and with water to remove salt. Specimens covered with PBS were used as controls. Detection of the amounts of adsorbed salivary proteins was performed directly on material surfaces by a chemiluminescence-based detection method as described below.
2.4
Wettability of material surfaces
Material specimens precoated with salivary proteins or left in phosphate-buffered saline were first dried in a desiccator over night. Water contact angles on material surfaces were then measured using the sessile drop method with a P1 goniometer of Erna (Tokyo, Japan). Two microliter-droplets were advanced toward the samples by a syringe tip until the droplets made contact with the sample surfaces. Left and right contact angles were read for each droplet exactly 30 s after deposition at the surface and these values were then averaged. Droplets were analyzed on six randomly selected pure surfaces and twelve surfaces coated with salivary proteins for each material. Material surfaces with contact angles higher than 63° were considered hydrophobic .
2.5
Detection of salivary protein adsorption on material surfaces
Amounts of adsorbed proteins were determined directly on material surfaces by chemiluminescence as described by us in detail elsewhere . Amino groups were first biotinylated with sulfo-NHS-LC-biotin (Pierce, Rockford, IL, USA), and then detected by binding of horseradish peroxidase-conjugated avidin-D. For detection of chemiluminescence, each material surface was overlaid with a luminol-containing solution and immediately exposed to a light-sensitive film (X-ray film). For establishment of standard curves, serial dilutions of salivary proteins were dotted in duplicate onto nitrocellulose membranes (Minifold I dot-blot system (Schleicher & Schuell, Dassel, Germany)) to immobilize the proteins onto nitrocellulose membranes (Schleicher & Schuell). Signals on X-ray films were densitometrically analyzed using Optimas software (version 6.2; Optimas Corporation, Bothell, WA, USA) after scanning the films utilizing an image scanner. A standard curve between amounts of salivary proteins and inverse grey levels was then fitted to the data (TableCurve 2D V5.01; Systat Software Inc., Chicago, IL, USA). The amounts of salivary proteins attached to material surfaces were calculated after densitometric analyses of signals on X-ray films obtained from standard curves and specimens as described.
2.6
Bacterial culture and detection of adherent bacteria
Cultures of S. gordonii strain DL1 (Challis) were grown as stationary suspension cultures in brain heart infusion (VWR) in a microaerophilic atmosphere (5% CO 2 ) for 24 h at 37 °C. Then, the cells were washed in PBS, and suspensions of 10 8 cells per ml in PBS containing 1 mM CaCl 2 and 1 mM MgCl 2 were prepared after measuring the optical density at 600 nm. Biotinylation and in situ detection of surface-attached bacteria was performed as described . The microorganisms were labeled with sulfo-NHS-LC-biotin, and the number of bacteria attached to each material surface was calculated. Material specimens precoated with salivary proteins or left in PBS were incubated for one hour at 4 °C with biotinylated bacteria to allow for binding and the analysis of initial bacterial adhesion. The specimens were then washed with PBS to remove unbound bacteria, and subsequently incubated with a solution of horseradish peroxidase-conjugated avidin-D in TBS/0.1% Tween20 for 30 min. After that, the specimens were washed again with TBS-Tween20, for detection of chemiluminescence, each material surface was overlaid with a luminol-containing solution and immediately exposed to a light-sensitive film (X-ray film). Standard curves of labeled bacteria were prepared from serial dilutions of bacterial suspensions and immobilized on nitrocellulose membranes using a Minifold I dot-blot system (Schleicher & Schuell). The numbers of microorganisms identified on material surfaces were calculated after densitometric analyses of signals on X-ray films obtained from standard curves and specimens as described using the Optimas software (version 6.2, Optimas Corporation, Bothell, WA, USA). Standard curves between numbers of microorganisms and inverse grey levels were then fitted to the data (TableCurve 2D V5.01; Systat Software Inc., Chicago, IL, USA).
2.7
Scanning electron microscopy (SEM)
Incubation of materials with S. gordonii was performed as described. The specimens were then washed with PBS, fixed for 30 min by the addition of glutaraldehyde to a final concentration of 2.5%, washed again with PBS and water, and air dried. Specimens were mounted on stubs and tilted by 30° in the SEM chamber. Attached bacteria were visualized directly by a Quanta 400 FEG scanning electron microscope (FEI, Frankfurt, Germany) in low vacuum mode (0.08 Torr) using a large-field detector.
2.8
Statistical analysis
Water contact angles on material surfaces, amounts of adsorbed salivary proteins, and numbers of attached S. gordonii on material surfaces are shown as medians including the 25–75 % quartiles summarized from measurements in repeated independent experiments. The number of individual values is indicated in the figure legends. Differences between median values were statistically analyzed using the Mann–Whitney U -test (SPSS/PC+, Version 18.0 SPSS, Chicago, IL, USA) for pairwise comparisons among groups at the 0.05 level of significance. Correlation analyses were performed using best fits and corresponding equations (TableCurve 2D V5.01; Systat Software Inc., Chicago, IL, USA). Correlations are presented in graphs created using SigmaPlot 8.0 (Systat Software Inc.)
2
Materials and methods
2.1
Materials and chemicals
Polymethylmethacrylate (PMMA, Palapress) was obtained from Heraeus Kulzer GmbH (Hanau, Germany), polyethylene (PE; ultra high molecular weight polyethylene), cobalt–chromium–molybdenum (Co28Cr6Mo), and titanium (Ti6Al4V) were purchased from Zimmer GmbH (Winterthur, Switzerland). The silicone Mucopren soft came from Kettenbach GmbH (Eschenburg, Germany), a silorane-based dental composite (Filtek Silorane) and a glassionomer cement (Ketac Molar) was obtained from 3M ESPE AG (Seefeld, Germany), the methacrylate-based composite material Tetric EvoCeram came from Ivoclar Vivadent (Schaan, Lichtenstein), and polytetrafluoroethylene (PTFE) specimens were provided by the Institute of Chemistry (University of Regensburg). Caso-bouillon was obtained from VWR International (Darmstadt, Germany). Biotinamidohexanoic acid 3-sulfo-N-hydroxysuccinimide ester (sulfo-NHS-LC-biotin) was obtained from Pierce (Rockford, IL USA) and fluorescein isothiocyanate (FITC)-labeled avidin-D was bought from Vector Laboratories Inc. (Burlingame, CA, USA). Tween 20 was purchased from Sigma–Aldrich (Taufkirchen, Germany). All other chemicals and organic solvents were purchased in the highest available purity from VWR.
2.2
Sample preparation
Polytetrafluoroethylene (PTFE), polyethylene (PE), titanium (Ti6Al4V) and cobalt–chromium–molybdenum (Co28Cr6Mo) samples were cut from rods with a diameter of 11.3 mm and a thickness set to 2 mm. Titanium and CoCrMo sample surfaces were cleaned by sonication in acetone, toluol, acetone, ethanol, and water for 3 min each; PTFE and PE were sonicated in ethanol and water only. Teflon rings with an inner diameter of 11.3 mm and 2 mm in height were used to prepare specimens between two glass plates to obtain flat surfaces of PMMA, silicone (Mucopren soft), silorane-based and methacrylate-based dental composite as well as a glass ionomer cement (Ketac Molar). Surface roughness expressed as median peak-to-valley values ( R a ) were found between 0.04 μm (PMMA) and 0.53 μm (PE) using a perthometer (S6P, Mahr GmbH, Göttingen, Germany). PMMA, silicone (Mucopren soft), silorane- and methacrylate-based dental composite materials were prepared according to the manufacturer’s instructions. These specimens were stored in demineralized water at 25 °C for seven days. Glass ionomer cement (Ketac Molar) specimens were first allowed to set for seven minutes when prepared between glass plates, and then stored for twenty-four hours in a humidified chamber.
2.3
Salivary protein preadsorption to material surfaces
Unstimulated human whole saliva collected from two healthy males (24 and 29 years) was pooled and sterile filtered using membrane filters of 0.2 μm pore size (Sartorius, Germany). The protein content was determined by the bicinchoninic acid protein assay (BCA, Pierce, Rockford, IL USA) and samples were then stored at −20 °C until use. Material specimens were separately placed into each well of a 24-well plate filled with 1.2 ml saliva (0.6 mg/ml protein) and incubated for 30 min at room temperature. For measuring wettability of the materials surfaces as well as bacterial adhesion (see Sections 2.4 and 2.6 ) after protein adsorption, the specimens were washed three times for 10 min with phosphate-buffered saline (PBS) to remove excess protein and with water to remove salt. Specimens covered with PBS were used as controls. Detection of the amounts of adsorbed salivary proteins was performed directly on material surfaces by a chemiluminescence-based detection method as described below.
2.4
Wettability of material surfaces
Material specimens precoated with salivary proteins or left in phosphate-buffered saline were first dried in a desiccator over night. Water contact angles on material surfaces were then measured using the sessile drop method with a P1 goniometer of Erna (Tokyo, Japan). Two microliter-droplets were advanced toward the samples by a syringe tip until the droplets made contact with the sample surfaces. Left and right contact angles were read for each droplet exactly 30 s after deposition at the surface and these values were then averaged. Droplets were analyzed on six randomly selected pure surfaces and twelve surfaces coated with salivary proteins for each material. Material surfaces with contact angles higher than 63° were considered hydrophobic .
2.5
Detection of salivary protein adsorption on material surfaces
Amounts of adsorbed proteins were determined directly on material surfaces by chemiluminescence as described by us in detail elsewhere . Amino groups were first biotinylated with sulfo-NHS-LC-biotin (Pierce, Rockford, IL, USA), and then detected by binding of horseradish peroxidase-conjugated avidin-D. For detection of chemiluminescence, each material surface was overlaid with a luminol-containing solution and immediately exposed to a light-sensitive film (X-ray film). For establishment of standard curves, serial dilutions of salivary proteins were dotted in duplicate onto nitrocellulose membranes (Minifold I dot-blot system (Schleicher & Schuell, Dassel, Germany)) to immobilize the proteins onto nitrocellulose membranes (Schleicher & Schuell). Signals on X-ray films were densitometrically analyzed using Optimas software (version 6.2; Optimas Corporation, Bothell, WA, USA) after scanning the films utilizing an image scanner. A standard curve between amounts of salivary proteins and inverse grey levels was then fitted to the data (TableCurve 2D V5.01; Systat Software Inc., Chicago, IL, USA). The amounts of salivary proteins attached to material surfaces were calculated after densitometric analyses of signals on X-ray films obtained from standard curves and specimens as described.
2.6
Bacterial culture and detection of adherent bacteria
Cultures of S. gordonii strain DL1 (Challis) were grown as stationary suspension cultures in brain heart infusion (VWR) in a microaerophilic atmosphere (5% CO 2 ) for 24 h at 37 °C. Then, the cells were washed in PBS, and suspensions of 10 8 cells per ml in PBS containing 1 mM CaCl 2 and 1 mM MgCl 2 were prepared after measuring the optical density at 600 nm. Biotinylation and in situ detection of surface-attached bacteria was performed as described . The microorganisms were labeled with sulfo-NHS-LC-biotin, and the number of bacteria attached to each material surface was calculated. Material specimens precoated with salivary proteins or left in PBS were incubated for one hour at 4 °C with biotinylated bacteria to allow for binding and the analysis of initial bacterial adhesion. The specimens were then washed with PBS to remove unbound bacteria, and subsequently incubated with a solution of horseradish peroxidase-conjugated avidin-D in TBS/0.1% Tween20 for 30 min. After that, the specimens were washed again with TBS-Tween20, for detection of chemiluminescence, each material surface was overlaid with a luminol-containing solution and immediately exposed to a light-sensitive film (X-ray film). Standard curves of labeled bacteria were prepared from serial dilutions of bacterial suspensions and immobilized on nitrocellulose membranes using a Minifold I dot-blot system (Schleicher & Schuell). The numbers of microorganisms identified on material surfaces were calculated after densitometric analyses of signals on X-ray films obtained from standard curves and specimens as described using the Optimas software (version 6.2, Optimas Corporation, Bothell, WA, USA). Standard curves between numbers of microorganisms and inverse grey levels were then fitted to the data (TableCurve 2D V5.01; Systat Software Inc., Chicago, IL, USA).
2.7
Scanning electron microscopy (SEM)
Incubation of materials with S. gordonii was performed as described. The specimens were then washed with PBS, fixed for 30 min by the addition of glutaraldehyde to a final concentration of 2.5%, washed again with PBS and water, and air dried. Specimens were mounted on stubs and tilted by 30° in the SEM chamber. Attached bacteria were visualized directly by a Quanta 400 FEG scanning electron microscope (FEI, Frankfurt, Germany) in low vacuum mode (0.08 Torr) using a large-field detector.
2.8
Statistical analysis
Water contact angles on material surfaces, amounts of adsorbed salivary proteins, and numbers of attached S. gordonii on material surfaces are shown as medians including the 25–75 % quartiles summarized from measurements in repeated independent experiments. The number of individual values is indicated in the figure legends. Differences between median values were statistically analyzed using the Mann–Whitney U -test (SPSS/PC+, Version 18.0 SPSS, Chicago, IL, USA) for pairwise comparisons among groups at the 0.05 level of significance. Correlation analyses were performed using best fits and corresponding equations (TableCurve 2D V5.01; Systat Software Inc., Chicago, IL, USA). Correlations are presented in graphs created using SigmaPlot 8.0 (Systat Software Inc.)
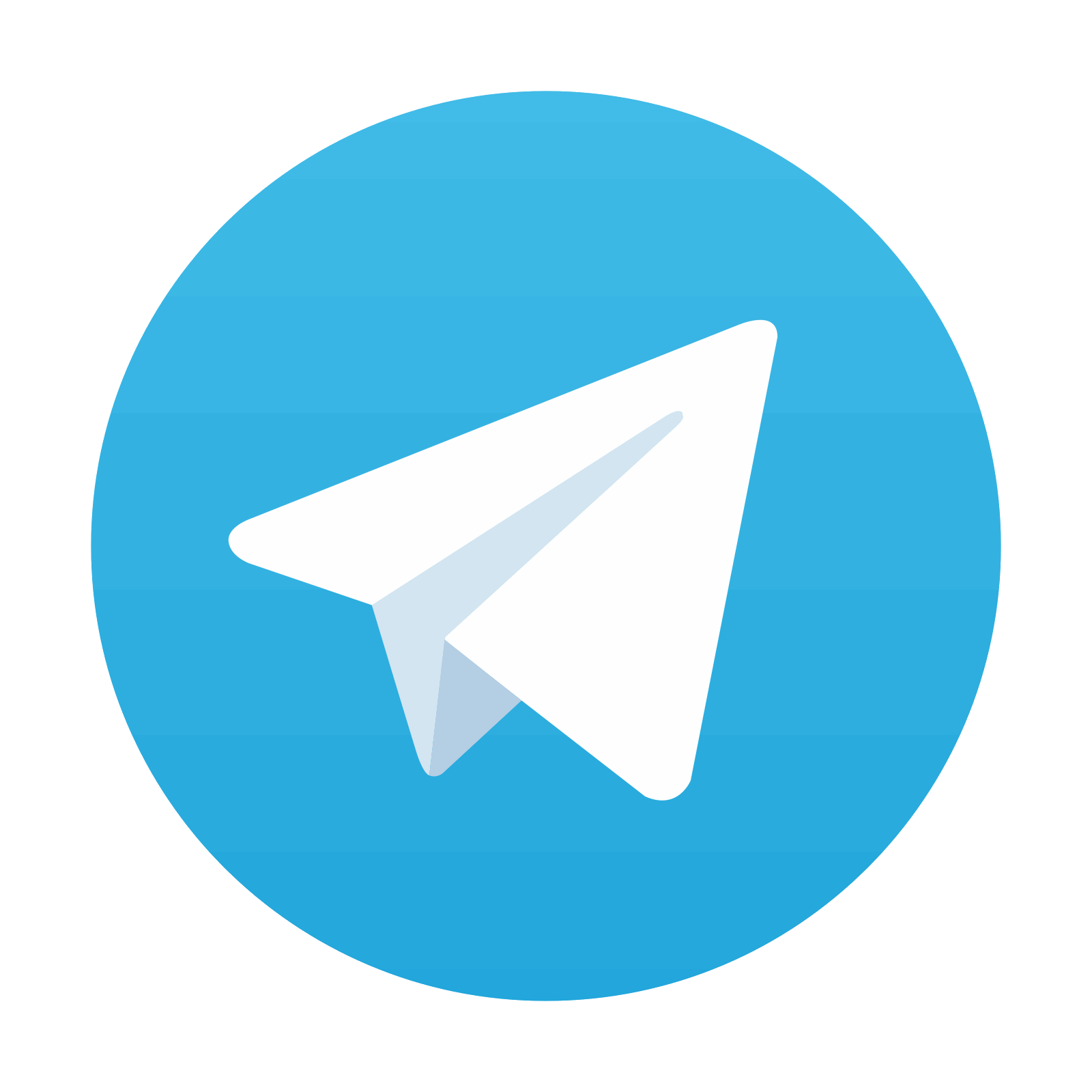
Stay updated, free dental videos. Join our Telegram channel

VIDEdental - Online dental courses
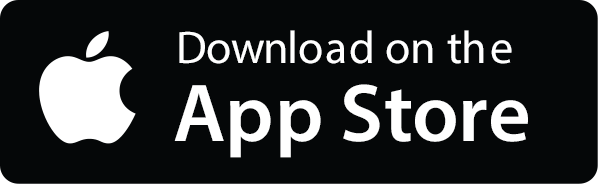
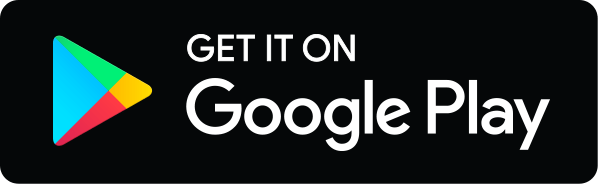