Abstract
Objective
A high-viscosity consistency of the glass-ionomer cement (GIC) may lead to poor adaptation into the cavity. The use of a flowable GIC layer seemed to improve its adaptation in approximal restorations in vitro. In this study we assessed the flexural strength of a two-layered GIC, using a flowable GIC as a liner (two-layer technique). Additionally, finite element analysis on standardized bar-shaped models and on a representative tooth model was performed to rationalize the obtained results.
Methods
The flexural strength and Young’s modulus were calculated from the results of a three-point-bending test. Bar-shaped specimens were prepared either with a conventional GIC, with a flowable GIC (powder/liquid ratio 1:2), or with two-layers (either with the flowable layer down or on the top of the specimen). Three dimensional FEA models of the bar-shaped specimens and a model of tooth 46 provided information on the stress distribution of each component of the specimen and on the restoration.
Results
The apparent flexural strength and Young’s modulus of both two-layered groups were significantly lower than that of the conventional group. FEA showed that the layers of the two-layer specimens with the flowable GIC down separated from each other under load. The tooth model showed better stress distribution for the two-layer restorations.
Significance
The two-layer GIC showed inferior flexural strength, which might be explained by the detachment of the layers under load. Nevertheless the tooth model showed that the two-layer GIC provides a lower stress concentration on the occlusal surface of the material.
1
Introduction
Glass ionomer cements (GIC) are the most used filling material for minimal invasive restorations done by Atraumatic Restorative Treatment (ART) . Essentially, this is due to the combination of its adhesive properties with a relatively slow chemical setting reaction, and a possible therapeutic effect on demineralized enamel and dentin provided by the fluoride released from the GIC . Specially developed for ART, high-viscosity GICs have better mechanical properties than their predecessors , but because of their brittleness and difficult handling, their performance on multi-surface cavities is far from optimal .
With regard to the adaptation of any restorative material in a posterior proximal cavity, the cervical margin is the weakest point. A high-viscosity material may lead to difficult insertion and cervical gaps, both of which contribute to restoration failure . Aiming to improve cervical adaptation and reduce secondary caries occurrence, GIC were often used as liners before the insertion of a resin composite or amalgam restoration in a technique called “open sandwich” . Laboratory studies suggested that a flowable GIC layer as a liner within proximal cavities before the insertion of a regular high-viscosity GIC (bi-layer technique) improved the adaptation of the material to teeth structures, reduced microleakage and allowed a better adhesion in sound dentin than in carious dentin .
However, a flowable GIC contains less reinforcing glass filler particles, thereby reducing the fracture resistance. We, therefore, assessed the load bearing capacity, the ability to withstand an applied stress, of a bi-layered GIC, using a flowable GIC as a liner (bi-layer technique). Additionally, finite element analysis (FEA) on standardized bar-shaped specimens model and on a representative tooth model were performed to rationalize the obtained results.
2
Materials and methods
The load bearing capacity and Young’s modulus were determined for conventional GIC, flowable GIC, and layered specimens where the flowable GIC was supposedly under tension (flowable GIC down) and compression (flowable GIC up), Bar-shaped specimens (25 mm × 2 mm × 2 mm; n = 20 per group) were made according to the ISO Standard ISO9917-2. The load bearing capacity and the Young’s modulus were determined using a three-point bending test. Hand mixed Fuji IX GP (GC Europe, Leuven, BE; batch nr 1105131) was used as GICs for all specimens.
Group 1 (conventional GIC only – C): specimens were prepared with a uniform layer of the GIC mixed according to the manufacturer’s instructions (conventional GIC, powder/liquid ratio 1:1).
Group 2 (bi-layer flowable GIC down – FD): specimens were prepared using a bi-layer technique. In the first layer one powder portion was mixed with two liquid drops (powder/liquid ratio 1:2). A flowable consistency mixture was achieved and the first layer inserted in the mold. Immediately thereafter, a second layer was mixed according to the manufacturer’s instructions (powder/liquid ratio1:1) and applied before the setting of the first layer.
Group 3 (bi-layer flowable GIC up – FU): specimens were prepared as in group 2, but were tested up side-down, to allow tensile stress to occur in the conventional layer.
Group 4 (flowable GIC only – F): specimens were prepared with a uniform flowable GIC layer mixing one powder portion with two liquid drops (powder liquid ratio 1:2).
Acetate strips covered with microscope slides were placed on the top and the bottom of the filled mold and the mold assembly was clamped and left to set for 15 min. All groups were stored at 37 °C in liquid paraffin. After 24 h the height and width of the specimens were measured using a digital calliper. The specimens were subjected to a three-point bending test on a universal testing machine (Hounsfield Ltd, Redhill, Surrey, UK) at a crosshead speed of 1.0 mm/min.
The load at failure ( F ) was used as load bearing capacity.
The Young’s modulus was determined using the stress and displacement values of the three point bending test. The displacement value (Δ z ) was calculated out of the displacement speed of the universal testing machine. The Young’s modulus ( E M ) was calculated using the following equation:
where ΔF is the increase of applied force, l the distance between the supports ( l = 20.0 mm), Δ z the increase in deflection at the increase of force, w the specimen width, and h the specimen height.
2.1
Finite element analysis (FEA)
The finite element modeling was carried out using FEMAP software (FEMAP 10.1.1; Siemens PLM software, Plano, TX, USA) and the analysis was done with NX Nastran software (NX Nastran; Siemens PLM Software, Plano, TX, USA). Three-dimensional FEA models of the three-point bending test set-up and of a proximal cavity in a permanent molar were created. For the Young’s modulus the following data were used: conventional GIC 12.6 GPa ( Table 1 ), flowable GIC 7.2 GPa ( Table 1 ), dentin 18.6 GPa, and a Poisson ratio of 0.3 was used for all materials. The tested models of the bi-layer specimens were analyzed with the two layers attached and detached from each other (groups 2a and 3a). The friction coefficient for the contact surfaces was 0.45. For the models with the two layers detached, the interface between the two layers was designed as a contact surface. The bar-shaped models (25 mm × 2 mm × 2 mm) were composed of 46,353 parabolic tetrahedron solid elements. The models were loaded with the experimental determined average fracture load ( Table 1 ). The nodes at the bottom of the model at the points of support were not allowed to move vertically. The nodes in the center of the models were only allowed to move in the vertical plane.
Group | Load at failure ( N ) | E (GPa) |
---|---|---|
1 (C) | 6.7 (2.6) a | 12.6 (5.1) a |
2 (FD) | 2.8 (0.6) c | 4.2 (2.2) c |
3 (FU) | 4.4 (2.1) bc | 5.5 (3.5) b |
4 (F) | 5.1 (1.9) ab | 7.2 (4.6) b,c |
In a model of tooth 46, an ocluso-mesial cavity of height 2.0 mm and depth 2.0 mm with a width of 2.5 mm was created. Two types of fillings were made: one of conventional GIC only and another of conventional GIC with a liner of 1 mm of a flowable GIC (two layers attached to each other). The models were composed of 52,365 parabolic tetrahedron solid elements. The cavity was loaded with a load perpendicular on the surface, where the vertical component was made 50 N. The resulting horizontal component was 10 N. A load perpendicular to the cavity surface is most likely the load direction in clinical situations. The nodes at the bottom of the tooth were fixed.
2.2
Statistical analysis
One-way ANOVA and Tukey post hoc were used to test differences for the load bearing capacity and the Young’s modulus. The data were analysed with SPSS 12.0.1 software (SPSS Inc., Chicago, IL, USA) using a 95% confidence interval.
2
Materials and methods
The load bearing capacity and Young’s modulus were determined for conventional GIC, flowable GIC, and layered specimens where the flowable GIC was supposedly under tension (flowable GIC down) and compression (flowable GIC up), Bar-shaped specimens (25 mm × 2 mm × 2 mm; n = 20 per group) were made according to the ISO Standard ISO9917-2. The load bearing capacity and the Young’s modulus were determined using a three-point bending test. Hand mixed Fuji IX GP (GC Europe, Leuven, BE; batch nr 1105131) was used as GICs for all specimens.
Group 1 (conventional GIC only – C): specimens were prepared with a uniform layer of the GIC mixed according to the manufacturer’s instructions (conventional GIC, powder/liquid ratio 1:1).
Group 2 (bi-layer flowable GIC down – FD): specimens were prepared using a bi-layer technique. In the first layer one powder portion was mixed with two liquid drops (powder/liquid ratio 1:2). A flowable consistency mixture was achieved and the first layer inserted in the mold. Immediately thereafter, a second layer was mixed according to the manufacturer’s instructions (powder/liquid ratio1:1) and applied before the setting of the first layer.
Group 3 (bi-layer flowable GIC up – FU): specimens were prepared as in group 2, but were tested up side-down, to allow tensile stress to occur in the conventional layer.
Group 4 (flowable GIC only – F): specimens were prepared with a uniform flowable GIC layer mixing one powder portion with two liquid drops (powder liquid ratio 1:2).
Acetate strips covered with microscope slides were placed on the top and the bottom of the filled mold and the mold assembly was clamped and left to set for 15 min. All groups were stored at 37 °C in liquid paraffin. After 24 h the height and width of the specimens were measured using a digital calliper. The specimens were subjected to a three-point bending test on a universal testing machine (Hounsfield Ltd, Redhill, Surrey, UK) at a crosshead speed of 1.0 mm/min.
The load at failure ( F ) was used as load bearing capacity.
The Young’s modulus was determined using the stress and displacement values of the three point bending test. The displacement value (Δ z ) was calculated out of the displacement speed of the universal testing machine. The Young’s modulus ( E M ) was calculated using the following equation:
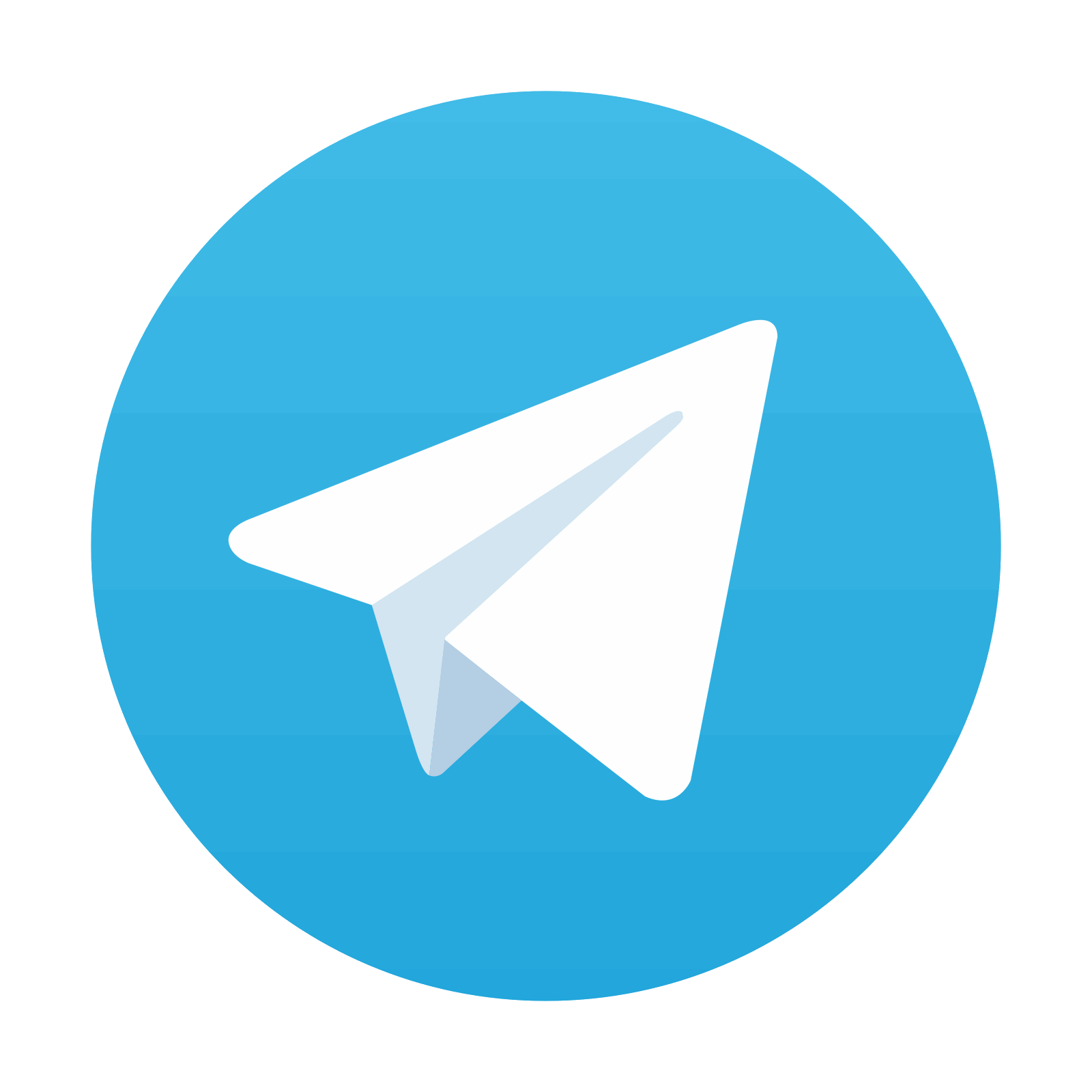
Stay updated, free dental videos. Join our Telegram channel

VIDEdental - Online dental courses
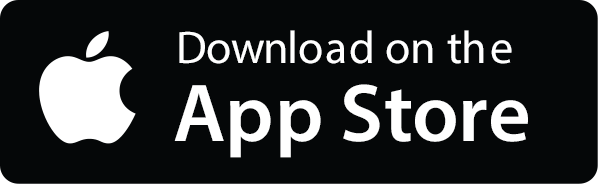
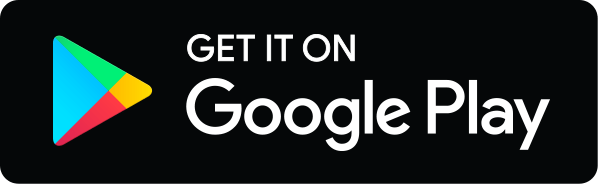