Highlights
- •
7.5% DHMAI composite showed a reduction in residual functions rate, release of monomers.
- •
7.5% DHMAI composite had preserved structural properties after 3 months of in vitro biological aging.
- •
7.5% DHMAI composite could enhance cytocompatibility behavior and improved longevity.
Abstract
Objective
To assess the impact of the quaternary ammonium antibacterial agent, Dimethyl-Hexadecyl-Methacryloxyethyl-Ammonium Iodide (DHMAI), on structural stability of an experimental resin composite after biological aging.
Methods
Experimental resin composites containing 7.5% of DHMAI were incubated in a biological medium in the presence of a Streptococcus Mutans (SM) strain during 3 months. The physicochemical, mechanical, and thermal properties, before and after 3 months of aging, were evaluated using: Degree of Conversion (DC), Residual Functions (RF), Vitreous Transition (Tg), Thermal Expansion Coefficient (CTE) and thermal degradation using Fourier Transform Infrared Spectroscopy Analysis (FTIRATR), Differential Scanning Calorimetry (DSC), Thermo Mechanical analyses (TMA) and Thermo Gravimetric Analysis (TG).
Results
Incorporation of DHAMI increased DC and decreased RF. After aging, DHMAI decreased and slowed RF release. Incorporation of 7.5% DHAMI provided significant modification of the thermal behavior (Tg and thermal degradation) but did not affect CTE. After aging, DHMAI enhanced the structural stability and improved resistance against biodegradation compared to the control composite.
Significance
The development of an antibacterial dental composite based on DHMAI improved its physical, mechanical, and thermal behaviors, possibly enhancing dental composite longevity. Results suggest that DHMAI could be used in the composition of other bioactive dental materials.
1
Introduction
The development of new and improved dental composites entails assessing whether they present good properties and stability during the period of use without influencing their effectiveness [ ]. Methacrylate-based composites are the most esthetic restorations currently used in direct techniques. The arrangement of the three-dimensional network of resin composites depends on the monomer structure that constitutes the resinous matrix [ ]. The dense network undergoes structural changes during the photopolymerization process. The methacrylate monomer system is transformed from a liquid state to a rubbery state, and then to a solid glass state. In addition, the structure of the monomers as well as the incomplete polymerization can greatly impact the final properties of the material [ ]. Incomplete polymerization may have potential disadvantages. The release of residual monomers from composite resins can stimulate the growth of cariogenic microorganisms around restoration [ , ]. Furthermore, several components were shown to induce allergic reactions and/or are potentially cytotoxic, genotoxic or mutagenic [ ]. Thus, knowledge concerning the structural properties and behavior of dental composites is necessary to improve their performance [ , ].
The stability of dental composites during function is defined by the extent to which they preserve the same characteristics and properties they had at the time of manufacture [ ]. The polymeric structure of dental composites can be degraded in aqueous media, either by a hydrolysis mechanism or an enzymatic reaction [ ]. A recent study has shown that the cariogenic Streptococcus mutans ( S. mutans ) bacteria has an esterase activity comparable to that of human saliva attaining levels that could degrade dental composites [ ]. This is particularly relevant since microorganisms have a high affinity for dental composites and can colonize their surfaces [ ].
The development of antibacterial dental composites aims to fight secondary caries and increasing dental restoration longevity [ ]. Among the strategies established to achieve this objective, is the current use of the Quaternary Ammonium Methacrylate (QAM) as an antibacterial monomer. This approach has great potential for decreasing biofilm formation around restoration. On dental composite surfaces, the immobilized QAM causes lysis of bacteria by contact of their positive charge with the negative charge of the bacterial wall [ , ]. Studies have shown that QAMs with 16 carbons in their alkyl chain are the most effective. A number of mono- and di-methacrylate monomers have been developed including N-DiMethylAmino-HexaDecyl Methacrylate (DMAHDM) [ , ], methacryloxylethylcetyl Dimethyl Ammonium Chloride (DMAE-CB) [ , ] and 2-methacryloxylethylhexadecyl Methyl Ammonium Bromide (MAE-HB) [ ]. The inhibitory force of these monomers depends on the halogen counter ion of QAM and the optimum amount which can be incorporated into the dental composite [ ]. The Dimethyl Hexadecyl Methacryloxyethyl Ammonium Iodide (DHMAI) was recently incorporated in dental composite formulation (75/25, wt/wt %) Bisphenol A Glycidyl Methacrylate and Triethylene Glycol Dimethacrylate (Bis-GMA/TEGDMA)). Our previous study of series of concentrations showed that 7.5% DHMAI had good mechanical properties and strong antibacterial activity against the cariogenic bacteria strain S. mutans [ ].
The aim of this in vitro study was to investigate the structural stability, thermal, and physicochemical properties of a 7.5% DHMAI experimental dental composite, after 3 months of aging, in the presence of S. mutans. Two hypotheses were suggested: (1) DHMAI would not affect the properties of the dental composite; (2) the 7.5% DHMAI composite would remain stable after 3 months of aging in the presence of S. mutans and would preserve its structural and physicochemical properties.
2
Materials and methods
2.1
Synthesis procedure of quaternary ammonium salt monomers (DHMAI)
The DHMAI (C 24 H 48 O 2 N + I − ) was produced using the Menschutkin synthesis which converts a tertiary amine to quaternary ammonium salts by reaction with an alkyl halide: 0.06 mol of 2-DiMethylAmino Ethyl Methacrylate (DMAEMA) was added to 0.05 mol of Iodohexadecane and 0.05 % of hydroquinone. All reagents were purchased from (Sigma-Aldrich, Saint-Quentin Fallavier, Isère, France) and were used without purification. The mixture was agitated under inert atmosphere at 50 °C for 12 h. The formed paste was washed with diethyl ether and then dried. DHMAI was obtained as a white powder [ ].
2.2
Preparation of resin composites
According to the results of our previous study [ ], two formulations ( Table 1 ) were selected for thermal analysis. DHMAI was added into (75/25, wt/wt %) (Bis-GMA/TEGDMA) with mass ratios of 7.5 wt%. The initiator system (Comphoroquinone (CQ) and DMAEMA), was added at mass ratio of 1 wt%. All reagents were purchased from (Sigma-Aldrich, Saint-Quentin Fallavier, Isère, France). Then, resin was filled with 71 wt% of silanized glass particles 8235-SCHOTT® (SCHOTT AG, Landshut, Bavaria, Germany) consisting of 10 % Al 2 O 3 , 10 % B 2 O 3 , 30 % BaO, and 50 % SiO 2 , (mean size 0.7 μm). The compounds were well blended using SpeedMixer™ (Hauschild, Hamm, North Rhine-Westphalia,Germany) to obtain homogeneous pastes, and then stored in sealed containers in darkness. Resin composite without DHMAI was prepared as a control.
Formulations | Organic phase 29 wt% | |||||
---|---|---|---|---|---|---|
BT (75/25) wt% |
MPC wt% |
DHMAI wt% |
CQ wt% |
DMAEMA wt% | ||
F1 | Control | 28.42 | 0 | 0 | 0.29 | 0.29 |
F2 | Antibacterial 7.5 % DHMAI | 26.29 | 0 | 2.13 | 0.29 | 0.29 |
2.3
Specimen preparation and biological aging
Pellet samples (wt 80−90 mg) of each composite ( Table 1 ) were prepared in a Teflon mold of 6 mm diameter and 1 mm thickness. Samples were irradiated on both sides under LED lamp Elipar™ S10 (3 M ESPE, Seefeld, Bavaria, Germany) for 40 s and then polished with 120-grit silicon carbide abrasive paper. The samples of each formulation were divided into two groups for before and after biological aging analysis.
For aging, S.mutans strain ATCC 25175 (Institut Pasteur, Paris, Île-de-France, France) were cultured in a brain heart infusion (BHI) medium. Pellet samples (n = 9) of each formulation ( Table 1 ) were UV sterilized (40 min) and placed in sterile flasks containing 50 mL BHI. Then, 10 mL of bacteria suspension at the optical density of DO 600 = (0.6 – 0.7) was added to each flask. The flasks were then incubated aerobically at 37 °C for 3 months. The culture media were renewed every 3 days and the purity of the bacteria was systematically checked by gram staining throughout the incubation period.
After 3 months of aging, the samples were collected and washed three times under sonication in sterile Dulbecco’s Phosphate Buffered Saline (DPBS), to eliminate the dense biological deposits from the surface. The samples were then dried in air and protected from light.
2.4
Degree of conversion and residual functions rate of resin composites
DC measure [ ] and the rate of RF were measured using FTIR spectroscopy IR 700 (Safas, Principauté, Monaco) with an attenuated total reflectance (ATR) sampling accessory. First, the spectrum of uncured formulations (F1 control- F2 antibacterial) was obtained using 4 scans at 4 cm −1 resolution. Then, the cured samples before and after aging ( n = 6), prepared as described above, were analyzed. Fig. 1 shows the absorbance intensities of the aliphatic double bond (C C) peak at 1638 cm −1 and the internal aromatic (C C) reference at 1607 cm −1 before photopolymerization, after photo polymerization and after aging. The DC (%) and RF (%) were calculated according to the following equations:
where P1 , P 1′ and P 1′ ‘ are the absorbance peaks at 1638 cm −1 of (C = C) aliphatic before photopolymerization, after photopolymerization, and after aging respectively. P2 , P 2′ and P 2′ ‘ are the absorbance peaks at 1608 cm −1 of (C = C) aromatic before photopolymerization, after photopolymerization and after aging respectively.

2.5
Thermal properties
2.5.1
Characteristic properties assessed by DSC
DSC 820 (Mettler Toledo, Viroflay, Île-de-France, France) was used to identify the characteristic properties of experimental composites before and after aging. Samples (n = 1) of 20−25 mg weight and 4 mg DHMAI particles were placed in aluminum crucibles and heated from 25 °C to 200 °C at a rate of 10 °C/min under 30 mL/min air flow.
2.5.2
Thermal expansion coefficient assessed by TMA
The CTE of experimental composites (n = 3) was evaluated using a Thermo-Mechanical Analyzer TMA/SDTA 840 (Mettler Toledo, Viroflay, Île-de-France, France) before and after aging. The linear expansion (dimensional changes) was carried out by contact of a quartz probe with samples at 0.02 N under heat treatment ranging from 25−80 °C. Each sample was subjected to two heating runs at a 5 °C/min rate. After each measurement, the machine was cooled until it reached 25 °C over a period of 10 min; the CTE was calculated at the second heating run between 25–60 °C using internal software.
2.5.3
Thermal characterization, stability and degradation behavior assessed by TGA
Thermal characterization, stability and degradation behavior were studied using the TGA/DSC2 STAR e System (Mettler Toledo, Viroflay, Île-de-France, France). Samples of experimental composites before and after aging (n = 1) were heated in an aluminum crucible (range 25−1000 °C), at a heating rate of 10 °C/min, under 50 mL/min air flow. The mass losses of sample composition were measured by the TG curves as a function of the temperature. The DTG curves were obtained by derivative TG curves to determine the most obvious temperatures of mass losses.
2.6
Statistical analysis
Due to the small number of our samples, non-parametric tests were used; the Mann-Withney Wilcoxon test for comparison between-group analyses (F1 group vs F2 group at t = 0 then at t = 3 months), and the Wilcoxon signed rank test for within-group analyses (t = 0 vs t = 3 months for F1 and F2 groups respectively). Statistical significance was accepted at p < 0.05.
3
Results
3.1
Degree of conversion and residual functions rate of resin composites
The DC and RF rates of experimental dental composites are shown in Fig.2 A and B respectively.
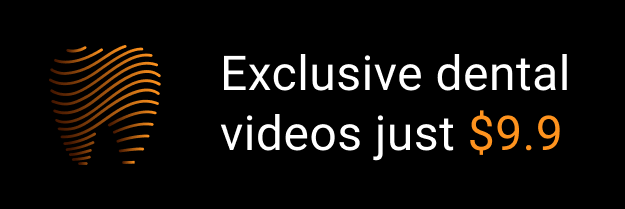