Highlights
- •
The optical and mechanical properties of pure zirconia are affected by low temperature degradation.
- •
First and second generation 3Y-TZP subjected to autoclave aging undergoes significant t-m phase transformation.
- •
t-m Phase transformation in ZTA composites is limited after hydrothermal aging.
- •
ZTA’s optical and mechanical properties remain stable after aging.
Abstract
Objective
To synthesize a zirconia toughened alumina (ZTA) composite with 70% alumina reinforced by 30% zirconia for dental applications and to characterize its microstructure and optical properties for comparison with the isolated counterpart materials and a first-generation 3Y-TZP.
Methods
Disc-shaped specimens were divided in four groups (n = 70/material): (1) 3YSB-E (first generation 3Y-TZP), (2) Zpex (second generation 3Y-TZP), (3) alumina, and (4) ZTA-Zpex 70/30. After synthesis, ceramic powders were pressed, and green-body samples sintered following a predetermined protocol. Specimens were polished to obtain a mirror surface finish. Apparent density was measured by Archimedes principle. X-ray diffraction (XRD) and scanning electron microscope (SEM) were used to characterize the crystalline content and microstructure. Reflectance tests were performed to determine the contrast-ratio (CR) and translucency-parameter (TP). Mechanical properties were assessed by biaxial flexural strength (BFS) test. All analyses were conducted before and after artificial aging (20 h, 134 °C, 2.2 bar). Optical parameters were evaluated through repeated-measures analysis of variance and Tukey tests (p < 0.05). BFS data were analyzed using Weibull statistics (95% CI).
Results
High density values (95–99%) were found for all ceramic materials and SEM images exhibited a dense microstructure. While XRD patterns revealed the preservation of crystalline content in the ZTA composite, an increase in the monoclinic peak was observed for pure zirconias after aging. Significantly higher CR and lower TP values were observed for the ZTA composite, followed by alumina, 3YSB-E, and Zpex. The highest characteristic stress was recorded for 3YSB-E, followed by intermediate values between ZTA and Zpex, and the lowest for alumina. Aging affected the optical and mechanical properties of both zirconias, while remained stable for ZTA composite and alumina.
Significance
The synthesis of experimental 70−30% ZTA composite was successful and its relevance for dental applications relies on its higher masking ability, aging resistance, and strength similar to zirconia.
1
Introduction
Among the available ceramic materials in dentistry, yttrium-stabilized tetragonal zirconia polycrystals (3Y-TZP) has been broadly used for manufacturing infrastructures of single crowns [ , ] and fixed dental prostheses [ , ] for both tooth and implant-supported reconstructions. 3Y-TZP has also been indicated for bonded bridges [ ], full arch implant supported prostheses [ ], root posts [ ], implant abutments [ ], and dental implants [ ].
3Y-TZP’s capability of stress mediated phase transformation from tetragonal to monoclinic (t-m) phase, as a mechanism to resist the crack propagation in the core (transformation toughening) remains as one of its most interesting properties for load bearing applications [ ]. When submitted to mechanical stress, zirconia grains around the crack tip undergoes a martensitic t-m transformation with a volumetric expansion of 3–5% of the grain size. Thus, the compressive stress produced during t-m transformation limits crack propagation increasing the fracture toughness of 3Y-TZP, a phenomenon known as R-curve behavior [ ]. As a result, with a fracture toughness of 5−10 MPa and flexural strength greater than 1000 MPa [ ], 3Y-TZP offers the highest mechanical properties among all ceramic materials along with excellent biocompatibility.
The highlighted mechanical properties of 3Y-TZP have led to a broad indication spectrum for long-span prostheses in dentistry, nevertheless clinical studies commonly do not involve follow ups longer than 10 years, but it has been reported that for long span applications including partial and full-arch fixed dental prostheses the survival rates are still lower than for metal ceramics, considered a gold-standard material [ ]. Although the main issue reported in up to 5 year follow up studies is porcelain veneer cohesive fractures, known to be multifactorial [ ], other problems have emerged as fatigue accumulates, including framework fractures, as reported in some clinical studies [ , ].
One important aspect that opens the field for innovations is the fact that zirconia is a metastable material where phase transformation may alter its properties. Within this context, variations in fabrication methods (like pressing method, sintering protocol, cooling rate and even milling parameters) [ ] may lead to materials with varying properties. It has been reported, for instance, that phase transformation from tetragonal to monoclinic may vary from 2.13% to 81.4% in zirconia stabilized materials, as a function of variability in several parameters including microstructure, grain size, manufacturing and processing methods, along with laboratory aging protocols [ ], suggesting that varying cumulative success rates from long-term clinical trials may be expected in the future also for framework survival [ , ].
Although 3Y-TZP was first introduced in Orthopedics in the 1990s for the manufacture of femoral head prostheses, the critical events in 2001 where hundreds of prostheses fractured due to an accelerated LTD process, raised concerns about long-term hydrothermal stability of zirconia [ ]. For dental applications, zirconia also remains in direct contact with body fluids making it susceptible to tetragonal to monoclinic phase transformation due to stress and low temperature degradation (LTD) [ ].
LTD process is accompanied by the appearance of micro cracks by the accumulation of stress within the material and the progressive loss of mechanical properties [ ]. In addition, during the fabrication of porcelain fused to zirconia prostheses, moisture of the porcelain suspension and its subsequent heating during the sintering process have been shown to trigger tetragonal to monoclinic phase transformation of 3Y-TZP [ ], which may compromise the porcelain stability due to increased residual stress [ , ]. Lastly, phase transformation at porcelain veneer/framework and intaglio surfaces may result in alterations in optical properties that may hamper long-term esthetic results, considering the role of the prostheses framework in the optical properties of the final bilayered restoration [ ].
To improve the hydrothermal stability of 3Y-TZP at room temperature, the combination of zirconia particles within an alumina matrix has been proposed [ , ]. Zirconia toughened alumina (ZTA) are polycrystalline ceramic composites composed of an alumina matrix and a secondary phase of metastable tetragonal zirconia combining the advantageous properties of both materials [ ]. Studies in orthopedics have revealed an increase in strength and fracture toughness of the composite material with little reduction in hardness and elastic modulus compared to monolithic alumina. In addition, the alumina matrix contributes to the stability of the tetragonal phase of zirconia by decreasing the LTD phenomena of 3Y-TZP [ , ].
As transformation mechanism propagates through the nucleation of a zirconia grain and the growth and transformation of neighboring grains, it has been suggested that the maximum fraction of zirconia in a ZTA composite to limit the propagation of the transformation is related to the interconnectivity of the zirconia grains within the alumina matrix [ ].This fraction, known as percolation threshold, has been suggested to be up to 16% zirconia in 84% alumina matrix [ , ]. Nevertheless, studies on Y-TZP content between 15 and 30% have presented promising results for ZTA composites that encourage future investigation [ ].
This study sought to develop a synthesis method for a ZTA composite in 70% Al 2 O 3 and 30% Zr 2 O and characterize its aging resistance along with possible changes in optical, mechanical and microstructural properties of ZTA after an accelerated aging protocol. We hypothesized that the formulated ZTA composite would be resistant to the accelerated aging protocol and that optical and mechanical properties would only be altered in the pure zirconia materials.
2
Materials and methods
2.1
Experimental ceramic materials fabrication
The four experimental groups were: (1) 3YSB-E: first generation 3Y-TZP with a particle size of 90 nm (TZ-3YSB-E, Tosoh Corporation, Tokyo, Japan); (2) Zpex: second generation 3Y-TZP with enhanced translucency when compared to first generation 3Y-TZP, with a particle size of 40 nm (Zpex, Tosoh Corporation); (3) alumina: high purity alumina with a particle size of 350 nm (α-Al 2 O 3, Baikalox Regular CR10, Baikowski, Poisy, France); and (4) ZTA-Zpex: ZTA composite comprised by 70% alumina (α-Al 2 O 3 , Baikalox Regular CR10, Baikowski) and 30% zirconia (Zpex, Tosoh Corporation). The composition of the ceramic powders is detailed in Tables 1 and 2 .
3Y-TZP powders chemical composition [wt.-%] | |||||||
---|---|---|---|---|---|---|---|
Particle size (nm) | Y 2 O 3 | Al 2 O 3 | Na 2 O | SiO 2 | FeO 2 O 3 | HfO 2 | |
3YSB-E | 90 | 5.35 | ≤0.1–0.4 | ≤0.04 | ≤0.02 | ≤0.01 | <5.0 |
Zpex | 40 | 5.2 ± 0.2 | ≤0.1 | ≤0.04 | ≤0.02 | ≤ 0.01 | <5.0 |
Al powder composition | |||||||||
---|---|---|---|---|---|---|---|---|---|
Particle size (nm) | Crystal structure | Chemical analyses ICP (ppm) | |||||||
Alpha | Gamma | Fe | Na | Si | Ca | K | Mg, Ti, Cr, Mn, Ni, Cu, Zn | ||
Baikalox Regular CR10 | 350 | 95% | 5% | 6 | 13 | 18 | 2 | 22 | <1 each |
For alumina specimen synthesis, an ethanol suspension containing the high purity alumina powder, 1% of polyvinyl butyral binder (Sigma-Aldrich, St. Louis, Missouri, USA) and 500 ppm of magnesium oxide was prepared. The suspension was homogenized and mixed in a friction mill for 4 h using high-purity alumina spheres. The slurry was dried in rotary evaporator (801, Fisaton, São Paulo, Brazil) and the powders were manually granulated and sieved.
For ZTA composite synthesis, a suspension in ethanol containing alumina (70%) and zirconia (30%) powders were prepared. The suspension was homogenized and mixed, then the slurry was dried, and the powder was granulated and sieved as previously described for pure alumina powder.
Pure zirconia (3YSB-E and Zpex), alumina, and experimental ZTA powders were uniaxially pressed at 1148 kgf for 30 s in a tungsten carbide matrix with 15 mm of diameter to obtain disc shaped green body samples with 1.8 mm thickness. Green body samples were double wrapped and sealed in a vacuum sealer (Jumbo Plus, Globavac, Itajai, SC, Brazil) and subjected to isostatic pressing performed on cold isostatic press (National Forge, Irvine, PA, USA) at room temperature for 30 s at 30000 psi.
Samples were sintered at 1500°C (Zpex and 3Y-SBE) and 1600°C (Alumina and ZTA composite) for 1 h (Zyrcomat Furnace, Vita Zahnfabrink, Bad Säckingen, Germany) with heating and cooling rate of 4 °C per minute. Polishing of the two flat surfaces was performed in a semi-automatic polishing machine (Automet 2000, Buehler, Lake Buff, IL, USA) with 220, 120, 90, 40, 25, 15, 9, 6 and 1 μm granulated diamond disks (ALLIED High Tech Products, Rancho Dominguez, CA, USA) with diamond suspensions. Forty specimens with 12 mm of diameter and 1 mm thickness were prepared for optical and microstructural characterization (n = 10/per material), and two hundred and forty specimens with the same dimensions were prepared for mechanical characterization through biaxial flexural strength (BFS) test (n = 60/per material).
The following characterization tests were performed before and after autoclave accelerated artificial aging (20 h, 134°C, 2.2 bar).
2.2
Density
The relative density was determined based on the Archimedes’ principle for all experimental materials after sintering. The density of the specimens was measured using an analytical balance (XS64 Analytical balance, Mettler Toledo, Columbus, OH, USA) and theoretical density kit accessory (Mettler Density Kit, Mettler Toledo, USA).
2.3
Scanning electron microscope (SEM)
The micromorphology of the experimental materials was analyzed at high-resolution Scanning Electron Microscope by Field Emission Gun (FEG-SEM) (MIRA3-TESCA, Brno-Kohoutovice, Czech Republic) with a secondary electron (SE) detector under magnification of 20,000× at 15–20 kV. Before evaluation, samples were submitted to thermal treatment at 1520 °C for Alumina and ZTA composites, and 1420 °C for Y-TZP specimens during one hour with heating and cooling rate of 4 °C per minute (Zyrcomat Furnace, Vita Zahnfabrink, Bad Säckingen, Germany). Subsequently, all samples were gold coated for 60 s at 20 mA (Quantum Sputter Q16OR ES).
2.4
Phase analysis
Crystalline spectra were determined for all experimental materials by X-ray diffraction (XRD, Miniflex, Rigaku, Tokyo, Japan). The scanning was performed on the Bragg θ-2 θ geometry, equipped with a graphite monochromator and Cu Kα radiation ( λ = 1.5406 Å), operating at a voltage of 40 kV and a current emission of 40 mA. Data were obtained in periods of 1.0 s and steps of 0.020 (2 θ ) of 10–80°. Baseline subtraction was performed in HighScore Plus Software (Malvern Panalytical Ltd, Westborough, Massachusetts, USA) for all XRD spectra. Monoclinic and tetragonal peaks were identified, and peaks intensity were recorded for monoclinic phase calculation. The monoclinic phase content (%) was quantified through formulas introduced by Toraya et al. [ ], as follows: <SPAN role=presentation tabIndex=0 id=MathJax-Element-1-Frame class=MathJax style="POSITION: relative" data-mathml='Xm=Im-111+Im111Im-111+Im111+It101′>??=(??(−111)+??(111))(??(−111)+??(111)+??(101))Xm=Im-111+Im111Im-111+Im111+It101
X m = I m – 111 + I m 111 I m – 111 + I m 111 + I t 101
, where I m (−111) and I m (111) represents the monoclinic peaks intensity (2 θ = 28° and 31.2°, respectively), and I t (101) represents the intensity of the tetragonal peak (2 θ = 30°); and, <SPAN role=presentation tabIndex=0 id=MathJax-Element-2-Frame class=MathJax style="POSITION: relative" data-mathml='Vm=1.311×Xm1+(0.311×Xm)’>??=1.311×(??1)+(0.311×??)Vm=1.311×Xm1+(0.311×Xm)
V m = 1.311 × X m 1 + ( 0.311 × X m )
, where Vm represents the monoclinic volumetric content.
2.5
Optical properties
Ten specimens of each ceramic material were analyzed in spectrophotometer CM 3700d (Konica Minolta) that operates in the wavelengths range of visible light (400–700 nm). After cleaning in 80% isopropyl alcohol ultrasonic bath for 10 min, reflectance tests were performed over white (Yw) and black (Yb) backgrounds, and the values were used to determine the contrast ratio (CR) and translucency parameter (TP).
CR is the property that measures the transparency or opacity of materials by the ratio of the reflectance of the specimen over a black background (Yb) to the reflectance of the same specimen over a white background (Yw), which is given per: <SPAN role=presentation tabIndex=0 id=MathJax-Element-3-Frame class=MathJax style="POSITION: relative" data-mathml='CR=YbYw’>??=????CR=YbYw
C R = Y b Y w
.
TP, which defines the masking ability of the material, was obtained by calculating the color difference (ΔE) of the specimens on black and white backgrounds, according to the equation: <SPAN role=presentation tabIndex=0 id=MathJax-Element-4-Frame class=MathJax style="POSITION: relative" data-mathml='ΔE=Lb*-Lw*2+ab*-aw*2+bb*-bw*212′>Δ?=((?*?−?*?)2+(?*?−?*?)2+(?*?−?*?)2)12ΔE=Lb*-Lw*2+ab*-aw*2+bb*-bw*212
Δ E = L b * – L w * 2 + a b * – a w * 2 + b b * – b w * 2 1 2
. Where the subscripts b* (black) and w* (white) indicate the background color, and the coordinates L*, a*, and b* correspond to the lightness, chromaticity on the red/green axis, and chromaticity on the yellow/blue axis, respectively.
2.6
Biaxial flexural strength (BFS)
Sixty specimens of each material group were randomly divided for immediate and aged conditions (n = 30) for BFS test using a piston-on-three balls device, according to ISO 6872−2015. Testing was performed in an ElectroPuls™ E3000 Linear-Torsion (Instron, Norwood, MA, EUA) equipment at a crosshead speed of 0.5 mm/min until fracture. The maximum load was recorded for each specimen (N), and the following equations were used to calculate biaxial flexural strength (MPa):
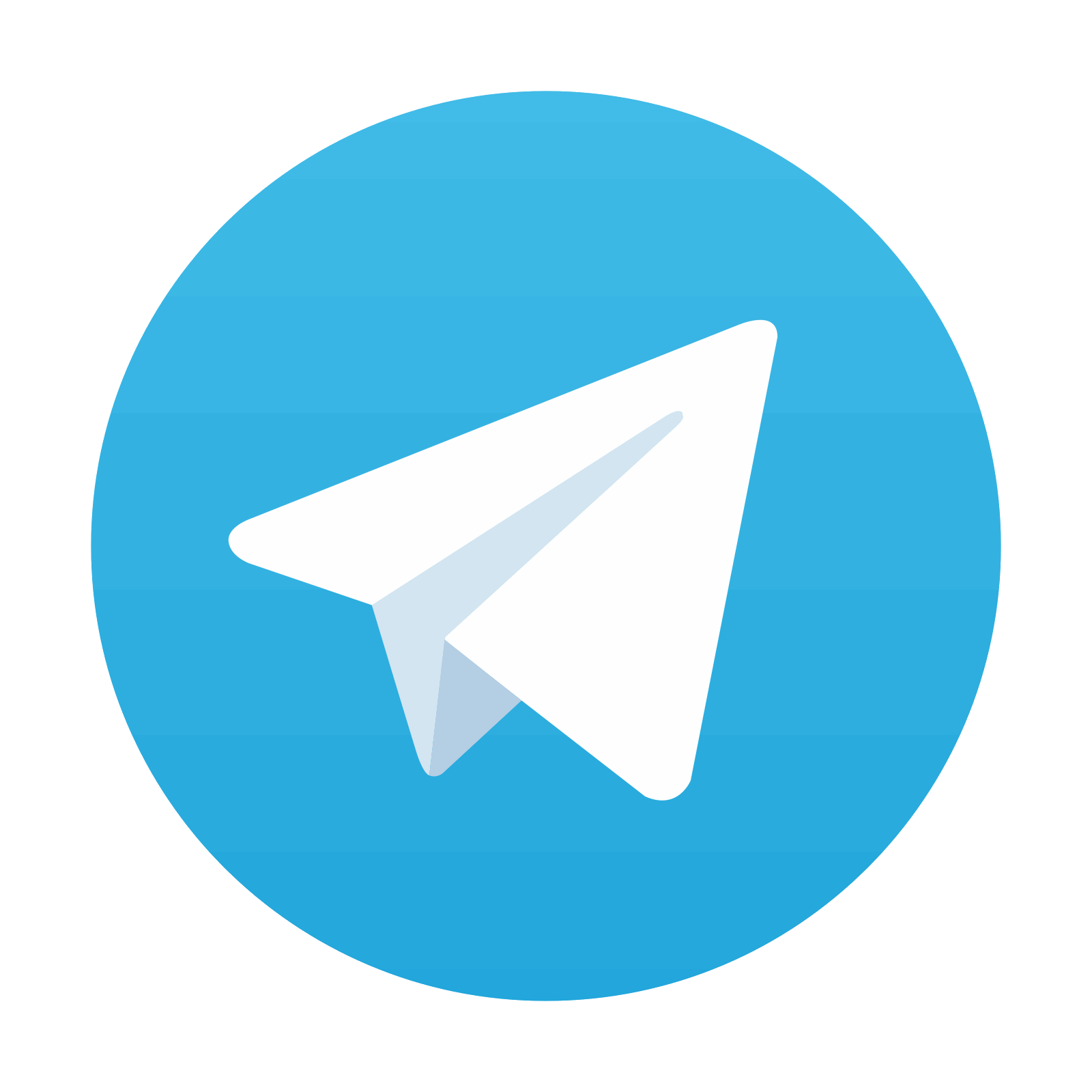
Stay updated, free dental videos. Join our Telegram channel

VIDEdental - Online dental courses
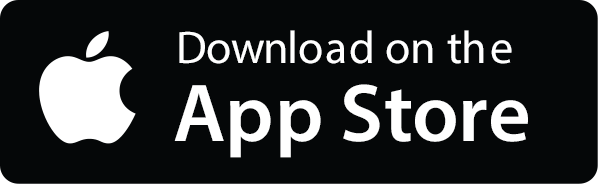
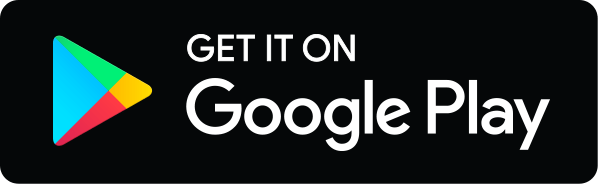
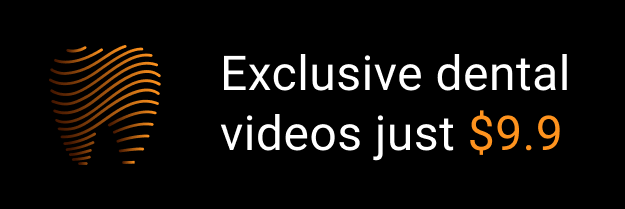