Fig. 1
Schematic representation of (a) cranial neural crest cell populations (red arrows) represented by the various colors and their migratory paths towards the oral cavity territory (blue–green arrows), and (b) the various stages of embryonic tooth development. fnp frontonasal process, 1st ba first brachial arch
At the bell stage, the mesenchyme gives rise to the dental pulp. Pulp cells adjacent to the dental epithelium start to differentiate into odontoblasts, while epithelial cells facing the dental pulp differentiate into ameloblasts [11, 12]. Odontoblasts are polarized columnar cells with processes that participate in the secretion of dentin matrix and minerals. Dentin matrix is composed mainly of collagen (90 %) and various non-collagenous proteins such as dentin sialophosphoprotein (DSPP) and dentin matrix protein 1 (DMP1) that represent the 10 % of the matrix. Apatite minerals are deposited on the matrix forming the mature calcified dentin. Following initial dentin deposition, the ameloblasts polarize and start to secrete the enamel matrix along the dentin-enamel junction. Enamel matrix is essentially composed of tissue specific hydrophobic proteins such as amelogenin, ameloblastin, enamelin, amelotin, tuftelin and ODAM (odontogenic ameloblast associated proteins ) [12, 13].
Molecular Control of Odontogenesis
Over the last years a big effort has been made to understand the molecular mechanisms that dictate tooth development, pathology, and repair [1, 14]. To this contributed the constantly increasing knowledge on genetics, stem cells, and tissue engineering.
Signaling molecules control all steps of odontogenesis by coordinating cell proliferation, differentiation, apoptosis, extracellular matrix synthesis, and mineral deposition. The same molecules are repetitively used during the different stages of odontogenesis and are regulated according to a precise timing mechanism [1, 15–17]. Signals produced at a wrong time lead to inappropriate cell proliferation, differentiation, and apoptosis, thus affecting the overall tooth development and morphology [1].
The territory where teeth will form is defined early, before any obvious sign of tooth development. The earliest marker of the odontogenic territory within the oral epithelium is the transcription factor Pitx2 [18, 19]. Dental fields are also established by morphogenic signals that determine the display and fate of the CNC cells, thus leading to the generation of distinct tooth shapes [20, 21]. For example, ectodysplasin (Eda) molecules are involved in the determination of dental fields size that controls teeth number [22]. In fact, increased Eda signaling leads to supernumerary teeth formation, while Eda deletion often leads to tooth number reduction [1, 22].
Bone morphogenic proteins (BMPs) , Wnts, sonic hedgehog (shh), and fibroblast growth factors (FGFs) regulate the epithelial-mesenchymal interactions during tooth initiation [1, 17]. BMP4 and FGF8 molecules constitute essential early oral epithelial signals that activate tooth specific genes (e.g. Msx1, Msx2, Barx1, Dlx1, Dlx2, Pax9) in the underlying mesenchyme [1, 18, 19, 23], thus controlling tooth patterning (i.e., BMP4 directs incisors shape while FGF8 molars shape) [2, 21].
Signals Specifying Dental Cell Fates
The specification of the various dental cell-types involves genes with restricted expression patterns during odontogenesis. It has been proposed that the determination of cell fates in teeth occurs via inhibitory interactions between adjacent cells through the Notch signaling pathway [17, 24]. Indeed, previous studies have shown that molecules of the Notch signaling pathway are involved in tooth development and dental cell specification [25–29]. In order to influence developmental decisions, molecules of the Notch signaling pathway must obviously interact with other signaling pathways. Notch-dependent cell fate acquisition between non-equivalent dental precursor cells is influenced by extrinsic signals such as BMP and FGF molecules. BMP and FGF molecules have opposite effects on the expression of Notch receptors and ligands in dental tissues [25, 26, 29], indicating that cell fate choices during odontogenesis are under the concomitant control of the Notch and BMP/FGF signaling pathways. Furthermore, it has been shown that Notch-mediated lateral inhibition has a pivotal role in the establishment of the tooth morphology, as shown in Jagged2 mutant mice where the overall development and structure of their teeth is severely affected [28, 29]. Several genetic findings have shown that Tbx1 plays a significant role for the early determination of epithelial cells to adopt the ameloblast fate. Indeed, hypoplastic incisors that lack enamel are observed in mice lacking the Tbx1 gene [30, 31]. Mesenchyme-derived FGF molecules activate the expression of Tbx1 in dental epithelium [32, 33].
Dental Pathologies
Traumatic injuries and external harmful agents such as bacteria and acids jeopardize tooth integrity. Periodontal disease and caries are mainly responsible for pathologies affecting the dental and alveolar bone tissues. Dental pathologies, combined with age, constitute important factors that can trigger tooth loss [34].
In most cases, the reparative mechanisms following dental and periodontal injuries involve a series of highly conserved processes that share genetic programs that occur throughout embryogenesis [14]. For example, after severe injury or inflammation of the dental pulp (i.e., pulpitis), the dying odontoblasts are replaced by progenitor cells, which differentiate into a new generation of odontoblasts and produce the reparative dentin [14, 35]. Signaling molecules released at the injury sites may attract progenitor cells, thus initiating the healing processes.
The increased knowledge on the reparative events within dental tissues has contributed to the proposal of alternative methods for the treatment of dental pathologies. However, traditional treatments continue to be applied in dental clinics since most of these novel approaches are at the experimental level and not well established yet.
Traditional Dental Treatments and Main Limitations
Restorative dentistry has undergone significant advances in recent years [36, 37]. Contemporary techniques to replace partially lost hard tissues consist of direct or indirect tooth-colored restorations (e.g., resin-based composites, porcelain inlays and onlays). The resin-based composites are bonded to the dental tissues through elaborated adhesive materials. While adhesion to enamel is stable over time, adhesion to dentin is weaker and unstable, mainly because of the higher levels of organic matrix of dentin [38]. Therefore, the goal of the adhesive procedures is the widespread infiltration and encapsulation of the demineralized collagen fibrils of dentin with the monomeric resin [37, 39]. Dentin biomodification strategies have as goal to enhance the properties of dentin matrix, thus inducing remarkable resin resistance against degradation that leads to short durability of restorations [39–41].
Endodontic therapy is a procedure implying the removal of contaminated or necrotic dental tissues within the pulp, thus eliminating future tooth contamination. Clinically, vital pulp therapy can be divided into two main groups: indirect and direct pulp capping. Indirect pulp capping is achieved by applying a protective agent on the thin layer of dentin remaining over a nearly exposed pulp, in order to allow the underlying dental pulp to repair [42]. Direct pulp capping necessitates the use of a protective agent directly on the exposed pulp, thus allowing pulp regeneration [43]. In case of large pulp exposure or infection, the coronal pulp has to be removed before direct pulp capping at the tooth root level (i.e., pulpotomy) [44]. This method preserves the vitality of pulp located at the root canal, thus allowing the accomplishment of the root growth [45]. Apexogenesis is another procedure of vital pulp treatment that promotes physiological development and formation of the root using mineral trioxide aggregates [46]. When the whole pulp is damaged or infected is replaced with inorganic materials such as guttapercha after thorough root canal treatment . However, root canal treatment results in the loss of dental pulp vitality. Dental pulp is an important element of the tooth since it provides nutrition, sensation, and defense against the various pathogens. Therefore, devitalized teeth are subsequent to various complications causing tooth strength reduction, increased fragility, and predisposition to postoperative fracture [47]. In terms of aesthetics, endodontic treatment often results in tooth crown discoloration due to either the filling material or the unsuccessful pulp chamber cleaning [48]. Thus, it becomes obvious that the maintaining of dental pulp vitality is of prime importance and this is highlighted by the emergence of new cell-based techniques focusing on partial or total pulp regeneration.
Missing teeth have traditionally been replaced with removable dentures , fixed bridges , and dental implants [49]. Currently, dental implants offer the most valuable option for tooth replacement, but a prerequisite for successful implant treatment is the quantity and quality of the remaining alveolar bone. Indeed, dental implant retention requires the close contact between the alveolar bone and the surface of the implant, a process called osseointegration . Dental implants are made of biocompatible titanium and they are inserted into the bone after surgical intervention. Survival rates of osseointegrated dental implants have been reported to be of over 95 % [50]. Apart alveolar bone quality and dimensions, the clinical success of implants also depends on primary implant stability, surgical methods, time of loading, infections, and implant surface characteristics [51]. When the dental implant is not well anchored to the bone, soft tissue encapsulation can occur, thus leading to unsuccessful treatment. Nanotechnology offer the opportunity to develop new implant surfaces that increased implant osseointegration [52], thus allowing the shortening of healing period without jeopardizing implant success [49]. However, the risk of infection of the tissues surrounding the implant (i.e., peri-implantitis) is still high and has to be taken seriously into account [53].
Recently, dental implants have been benefited from outcomes from stem cell biology and tissue engineering in order to maximize their survival rate in cases of poor bone quality. New regenerative technologies using scaffolds, stem cells, drug and growth factor delivery, and gene therapy are expected to enhance host tissue response and implant osseointegration [54]. Indeed, numerous pre-clinical studies have been performed already in various large animal models for guided bone regeneration around implants using growth factors and protein delivery [55, 56]. More recently, clinical studies have been realized using growth factor delivery for osseous and periodontal regeneration [57–59]. Pre-clinical applications for oral tissue regeneration using stem cell and gene therapies have been realized in rat models [60, 61]. Presently, safe and effective cell-based regenerative therapies have started to be applied in the dental clinics [54]. Clinical trials have documented that stem cells seeded in specific scaffolds are capable of generating sufficient amounts of bone in order to achieve primary implant stability [62–66]. However, all these new approaches should be further studied using controlled randomized clinical trials .
Alternative Dental Treatments
Novel cell-based therapeutic approaches have been proposed the last two decades [34, 67–69]. These treatments are promising, challenging, and complement traditional restorative or surgical techniques that are currently used in dentistry. The administration to patients of stem cells in conjunction with scaffolds and bioactive molecules might accelerate and increase the repair process of the impaired dental tissues. Although most of the studies focus on partial dental tissue regeneration, few attempts have been also made for the regeneration of entire teeth.
Here we report on selected dental stem cell populations that are currently used for experimental regenerative purposes in dentistry.
Stem Cells in Tooth Homeostasis and Regeneration
Stem cells play a critical role in tissue homeostasis and repair . Their fate is regulated by cell intrinsic determinants and signals from a specialized microenvironment [70–73]. Molecules of the Wnt, Notch, and BMP pathways have been shown to control stem cell fate specification [71, 74, 75]. The growing interest in the molecular regulation of dental stem cells arises from the potential to influence their fate and consequently their functions during tooth repair.
A considerable effort has been produced the last years to isolate epithelial and mesenchymal stem cell populations from deciduous and adult human teeth. Dental mesenchymal stem cells (DMSCs) were found in the dental pulp of permanent and exfoliated deciduous human teeth (Fig. 2) [11, 34, 69]. DMSCs were also identified in the apical part of dental papilla, the dental follicle, and the periodontal ligament. Because dental epithelial stem cells (DESCs) are very rare in adult human teeth, the current knowledge on DESCs has been obtained from studies in rodent incisors, which are continuously growing teeth [24, 76].
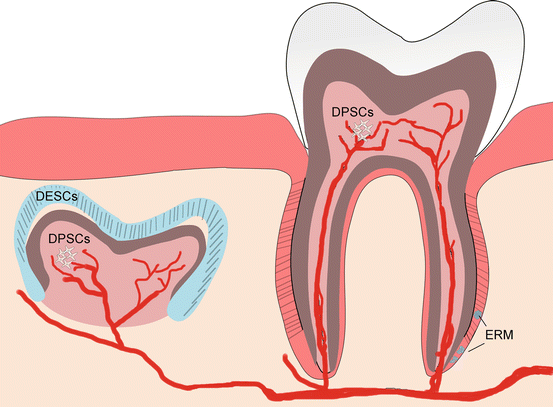
Fig. 2
Schematic representation of the locations of epithelial stem cells (i.e., hDESCs and ERM) and dental pulp stem cells that can be found in unerupted and erupted teeth of adult humans. ERM epithelial rests of Malassez, hDESCs human dental epithelial stem cells, DPSCs dental pulp stem cells
Stem Cell Populations Within the Dental Pulp
Activation of Notch signaling in various dental pulp cell populations during the dynamic processes triggered by dental injury may reflect the existence of specific stem cell pools [71, 77, 78]. Indeed, Notch expression is activated in endothelial cells, in cells located close to injury sites, as well as in cells located at the apex of the roots [71, 77]. Currently there are available protocols for the isolation and in vitro culture adult DMSCs [79–81]. These protocols are continuously being improved in order to further enrich the DMSC populations. Mesenchymal stem cell markers such as STRO-1, CD146 and CD44 are commonly used for the isolation of dental pulp stem cells (DPSCs) [11, 34, 79]. These cells are capable to differentiate into odontogenic, osteogenic, chondrogenic, adipogenic, myogenic, and neurogenic cells in vitro and in vivo [11, 34]. Pulp-dentin tissues have been generated after ectopic transplantation of DPSCs mixed with hydroxyapatite/tricalcium phosphate [79]. Furthermore, clinical trials using autologous human DPSCs combined with collagen scaffolds for alveolar bone reconstruction have been successfully performed several years ago [82].
Dental Epithelial Stem Cells
In mice, cells responsible for the continual growth of the incisor are located in the cervical loop area (posterior part of the incisor), which is a putative stem cell niche [76]. Cells originated from the cervical loop region are clonogenic and express Notch receptors and stem cell markers such as Sox2 and p75NTFR [26, 28, 76, 83]. This expression pattern indicates that Notch signaling plays a role in the maintenance of the dental epithelial stem cells. FGF3 and FGF10 are important mesenchymal signals involved in the maintenance and creation of the adult stem cell compartment within the cervical [84]. In the FGF10 null mice, the incisors are smaller due to the lack of the stem cell compartment in the cervical loop [84]. Therefore, FGF and Notch signaling pathways appear to interact for the maintenance of dental epithelial stem cells [76, 83].
In humans, dental epithelial stem cells (hDESCs) can be isolated from the third molars that develop after birth (Fig. 2) [11, 68]. Another source of hDESCs is the epithelial root sheath that disintegrates and forms the epithelial rests of Malassez (ERM) (Fig. 2) [11, 68]. ERM cells express epithelial stem cell markers such as Bmi-1, E-CAM, and p75, as well as embryonic stem cell markers such as Oct-4 and Nanog [24, 69].
Induced Pluripotent Stem Cells
Somatic cells can be reprogrammed and turned into pluripotent cells by the expression of a four transcription factors cocktail (i.e., Oct4, Sox2, c-Myc and Klf4) [85]. Similar to embryonic stem cells (ESCs) , induced pluripotent stem cells (iPS) can generate cells from all germ layers [85]. This discovery opened new perspectives for the field of regenerative medicine, thus allowing the conception of new treatment scenarios for the replacement of damaged or pathological tissues and organs. Therefore, a plethora of efforts have been produced the last years in order to design and adapt protocols that redirect iPS to specific cell types that could be used for the regeneration of a precise organ. In fact, the first clinical trial using iPS technology has been recently initiated: a sheet of iPS-derived retinal cells has been transplanted in a patient for the successful treatment of the age-related macular degeneration (AMD) pathology [86].
For dental-linked purposes, iPS have been generated from different dental cell types. Furthermore, iPS derived from skin fibroblasts have been used to produce various dental mesenchymal and epithelial cell populations [87].
Experimental Approaches for Tooth Regeneration
Regeneration of Dentin-Pulp Complex
Dental pulp is composed by a highly innervated and vascularized connective tissue containing a variety of cell types such as fibroblasts, immune cells, odontoblasts, and stem cells [88]. DPSCs show a limited potential to regenerate dentin, which becomes insufficient after tooth damage such as to caries or trauma. Several attempts have already been made in order to use DMSCs for the regeneration of the dentin-pulp complex in a variety of animal models. The first ever experiment realized using DMSCs isolated from human teeth has demonstrated that these cells are able to give rise to odontoblast-like cells, which form a dentin-like structure when transplanted together with HA/TCP ceramic powder into the dorsal surface of immunocompromised mice (Fig. 3a) [79]. Infiltration of mouse-derived blood vessels was observed in the dentin-pulp complex generated under these conditions.
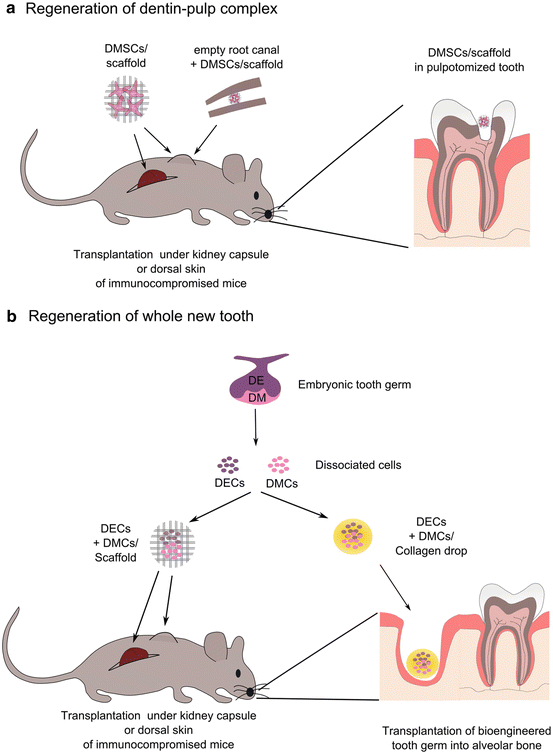
Fig. 3
Summary of different strategies for the regeneration of (a) dentin-pulp complex, and (b) an entire tooth. DE dental epithelium, DM dental mesenchyme, DECs dental epithelial cells, DMCs dental mesenchymal cells, DMSCs dental mesenchymal stem cells
More recent studies, using human DPSCs and SCAPs seeded on a poly-d,l-lactide/glycole scaffold and transplanted into the empty root canal space of mouse teeth, have demonstrated the ability of DMSCs to regenerate vascularized dentin-pulp complexes (Fig. 3a) [89–91].
Although being a useful in vivo model to assess the potency of DMSCs to form all or part of the various pulp tissues in vivo, the these experimental strategies were based on the transplantation of DMSCs in ectopic locations (Fig. 3a). Thus, those methods cannot be directly translated into a potential stem cell-based therapy in order to regenerate human dental tissues.
More recent studies have adopted more physiological strategies. For example, an experimental model consists of either the partial or total dental pulp removal (i.e., pulpotomy and pulpectomy, respectively), which is followed by fulfilling the empty space of the pulp chamber with DMSCs either alone or in combination with scaffold and bioactive molecules (Fig. 3a) [92–94]. For instance, autologous DPSCs transplanted together with granulocyte-colony stimulating factor (G-CSF) in pulpectomized teeth of dogs, gave rise to new dentin formation and vascularized and innervated pulp tissues [94]. Interestingly, partial pulp regeneration was also observed in pulpectomized teeth without DPSCs transplantation that were used as control, indicating that an endogenous population of DMSCs may be also participating in the regenerative process.
Regeneration of a Whole New Tooth
Severe dental pathologies can lead to tooth loss. In such cases, whole tooth regeneration could be the considered the right therapeutic approach. Functional teeth have been experimentally bioengineered by the re-association of disaggregated epithelial and mesenchymal cells from mouse embryonic tooth germs [67, 68, 95]. The bioengineered tooth germs can be subsequently transplanted into the alveolar bone and erupt into the oral cavity, thus replacing the missing teeth (Fig. 3b). Bioengineered erupted teeth possess enamel and dentin, are innervated, and can achieve normal occlusion indicating that are fully functional teeth [95]. However, it is quite difficult to control tooth size and shape during the in vitro development of these regenerated teeth. Indeed, the teeth formed using this experimental protocol were smaller when compared to the natural teeth. Thus, a big challenge is the generation of bioengineering functional teeth with the appropriate crown morphology. Therefore, the use of specifically designed scaffolds that will respect the crown anatomy of the various human teeth will greatly help to overcome that limitation [96–99]. Several attempts have been made towards this direction using dental epithelial and mesenchymal stem cells combined with tailor made scaffolds. Nevertheless, the result was the generation of teeth with irregular dental structures [96]. It is obvious that this method needs to be revisited and optimized before any application in dental clinics.
The previously mentioned approaches use embryonic dental stem cells in order to generate bioengineered teeth in the various small animal models. However, for the generation of teeth in humans the use of embryonic stem cells is not possible yet because of the various ethical and regulatory problems [34]. Therefore, the is an absolute need to find alternative sources of human stem cells that could serve for tooth regeneration purposes in the dental clinics. Ideally, those stem cells would be isolated from adult tissues. Although a sufficient number of DPSCs can be collected from the pulp of adult human teeth, harvesting DESCs from adult teeth for the generation of epithelial structures in the bioengineered teeth is almost impossible. In this sense, iPS technology could be of great help for the formation of dental epithelial tissues. Indeed, studies in mice have shown that mouse iPS can form ameloblast-like cells when co-cultured with dental epithelium [100]. In addition, a similar effect has been observed when mouse iPS were cultured in presence of ERM-conditioned medium [101] or recombined with dental embryonic mesenchyme [87]. However, it is still required to identify the precise molecules responsible for the redirection of iPS cells towards a dental epithelial fate. A better understanding of the regulation of iPS reprogramming towards dental cell types is essential in order to develop reproducible and safety strategies for new iPS-based therapies for regenerative purposes in dentistry.
Conclusion
The control of tooth regeneration necessitates a thorough understanding at the cellular and molecular level. The identification and characterization of dental epithelial and mesenchymal stem cell populations is a considerable accomplishment that can offer new perspectives in dentistry. Understanding when and how signaling molecules control the fate, migration, proliferation, and differentiation of dental stem cells will open new unexplored horizons for all dental disciplines. The acquired scientific knowledge together with novel approaches based on tissue engineering and nanotechnology are likely to instruct development of innovative dental therapies and materials.
Acknowledgements
This work was supported by funds of the University of Zurich (TAM and LJR) and the Polytechnic University of Marche (GO). The authors contributed to the planning, writing, critical reading, and editing of the present book chapter. The authors confirm that there are no conflicts of interest associated with this work.
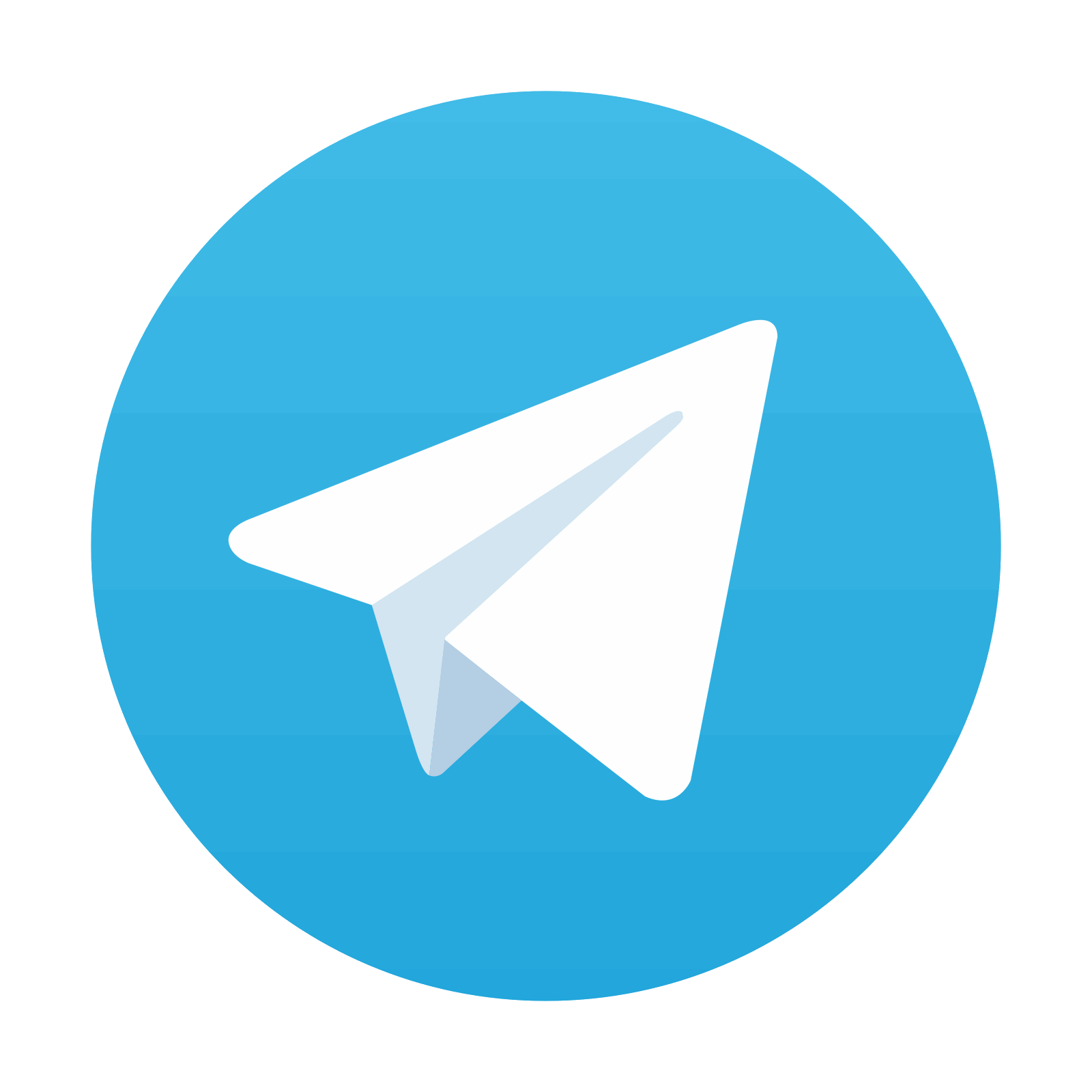
Stay updated, free dental videos. Join our Telegram channel

VIDEdental - Online dental courses
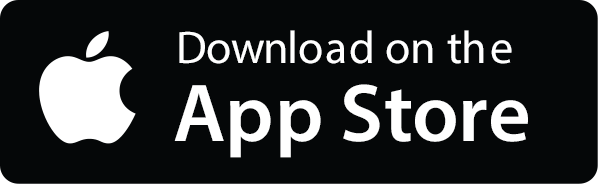
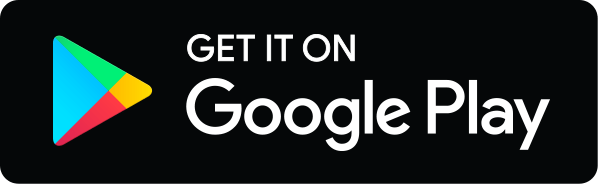