Fig. 1
Embryonic origin of the different tooth components. (Reproduced from www.bronxdentalspa.com/root-canal-bronx.htm)
Dentin comprises the main portion of the tooth. It is a mineralized connective tissue secreted by the odontoblasts, which are specialized cells located at the periphery of the dental pulp. The thickness of dentinal layer increases with age due to the deposition of secondary and tertiary (reparative) dentin, reducing the volume of the pulp chamber and the root canals [2]. It has been shown that the presence of dentin is essential for the differentiation of inner dental epithelial cells into ameloblasts [3]. Ameloblasts start to produce and secrete specific enamel matrix proteins, and soon after tooth eruption in the oral cavity, they completely disappear. During that time, tooth root develops, accompanied by cementum deposition and periodontium formation [4]. The cementum is a mineralized tissue covering the root of the tooth and produced by the cementoblasts . The main role of cementum is to serve as a medium by which the periodontal ligament can attach to the tooth for stability. At the cementum-enamel junction, the cementum is acellular. This acellular type of cementum covers about two-third of the root; the apical portion of the root, that is more permeable, is covered by cellular cementum [5]. The periodontal ligament anchors the tooth to the alveolar bone, which is the bone in which teeth are encased. The outside wall of the alveolar bone is compact bone; the trabecular bone is inside and contains bone marrow. The number and the size of the trabeculae in this bone are determined by the function activity of the organ.
Dental pulp is the soft connective tissue of the tooth. It is located in its central cavity and is surrounded by the hard structures of enamel, dentin and cement. The dental pulp contains four layers. The external layer (odontoblast layer) is made up of odontoblasts producing dentin; the second layer (cell-free zone) is poor in cells and rich in collagen fibers; the third layer (cell-rich zone) contains fibroblasts and undifferentiated mesenchymal cells. From this layer, undifferentiated cells migrate to various districts where they can differentiate under different stimuli and make new differentiated cells and tissues. The innermost layer (core of the pulp) comprises blood vessels and nerves that enter the tooth mostly through the apical foramen. Other cells in the pulp include fibrocytes, macrophages, and lymphocytes [6].
Dental Stem Cells (DSCs)
Unlike other tissues such as bone, which have the ability to repair and remodel throughout life, human teeth have a very limited capacity to regenerate upon injury or disease [7]. Of all the dental components, the acellular enamel is incapable of regenerating its original structure, whereas the remaining dental tissues possess that capacity in varying degrees, dependent on multiple factors [8]. Stem cells have been opening a promising future in regenerative medicine because of their two remarkable features known as self-renewal and multilineage differentiation. Stem cells reside in a dynamic and specialized microenvironment denoted to as niche, which is composed of heterogeneous cell types, extracellular matrix (ECM), and soluble factors [9]. The niche regulates stem cells behavior, by maintaining a balance between quiescence, self-renewal, and differentiation [10]. Based on their origin, stem cells can be generally classified in Embryonic Stem Cells (ESCs) , which differentiate into all cell types found in the human body, and Adult Stem Cells (ASCs) , whose differentiation potential is restricted to certain cell lineages. ASCs have been identified in almost every adult tissues from both epithelial and mesenchymal origin, including skin [11], bone marrow [12], adipose tissue [13], peripheral blood [14], cartilage [15], intestine [16], and periosteum [17]. For this reason, ASCs are also referred to as postnatal stem cells. ASCs are more applicable than ESCs in stem cell-mediated therapies and regenerative medicine because these cells lack ethical concerns. In addition, they have low immunogenicity and less tumorigenic potency than their embryonic counterparts, being promising candidates for regenerative therapies [18].
Several populations of ASCs have been identified also in various dental tissues, and they are collectively referred to as Dental Stem Cells (DSCs) [19]. DSCs are considered a promising source of ASCs since they are easily accessible by tooth extraction with a local anesthetic or when a primary tooth is replaced. More interestingly, DSCs can also be harvested from inflamed or diseased dental tissues, and their properties are similar to those of DSCs obtained from healthy tissues [20–22]. Therefore, it is believed that DSCs could retain, at least to some extent, the stem cell properties and tissue regeneration potential, making them an important tool for future developments in regenerative medicine. Another advantage of teeth as a source of stem cells is that, due to their ectomesenchymal origins, DSCs may display characteristics of both mesoderm and ectoderm [23]. This fact is very important because the association of mesenchymal (that will form odontoblasts, cementoblasts, osteoblasts, and fibroblasts) and epithelial (that will form ameloblasts) stem cells it is necessary for regenerating or building a new tooth.
Classification and Properties of DSCs
To date, seven different human dental stem/progenitor cells have been isolated and characterized: Dental Pulp Stem Cells (DPSCs) [7]; Stem cells from Human Exfoliated Deciduous teeth (SHED) [24]; Periodontal Ligament Stem Cells (PDLSCs) [25]; Dental Follicle Progenitor Cells (DFPCs) [26]; and Stem Cells from Apical Papilla (SCAP) [27]. DPSCs, SHED, and SCAP are generally referred to as dental pulp-related stem cells, PDLSCs and DFPCs as periodontium-related stem cells [28]. Other dental-related stem cells have been identified later. These are Gingival Mesenchymal Stem Cells (GMSCs) [29], and human Natal Dental Pulp Stem Cells (NDP-SCs) [30].
In this section, each type of DSCs will be described following the chronological order of their discovery (Fig. 2). Different biological aspects of DSCs will be discussed, starting from their first identification, the anatomical localization in the tooth (Fig. 3), the methods for their isolation, the peculiar expression of surface markers, and the differentiation potential in vitro and in vivo. The main biological properties of DSCs are summarized in Table 1.

Fig. 2
Timeline in the history of identification of the different DSCs
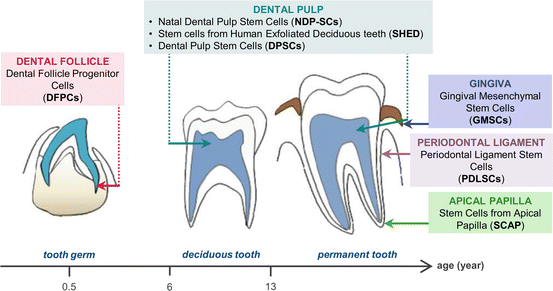
Fig. 3
Tooth developmental stages showing the anatomical localization of the different DSCs. (Reproduced from [31])
Table 1
Properties of DSCs
Type of DSCs
|
Location
|
Cell surface markers
|
Differentiation potential
|
References
|
||
---|---|---|---|---|---|---|
Positive
|
Negative
|
In vitro
|
In vivo
|
|||
DPSCs
|
Dental pulp of permanent tooth
|
CD9, CD10, CD13, CD29, CD44, CD59, CD73, CD90, CD105, CD106, CD146, CD166, STRO-1, Nanog, Oct-4, Sox-2
|
CD14, CD34, CD45, HLA-DR
|
Odontogenic
Osteogenic
Neurogenic
Adipogenic
Myogenic
Chondrogenic
|
Dentin/pulp-like complex
|
|
SHED
|
Dental pulp of deciduous tooth
|
CD13, CD29, CD44, CD73, CD90, CD105, CD106, CD146, CD166, STRO-1, Nanog, Oct-4, SSEA-3, SSEA-4, TRA-1-60, TRA-1-81
|
CD14, CD34, CD45, HLA-DR
|
Odontogenic
Osteogenic
Neurogenic
Adipogenic
Myogenic
Chondrogenic
|
Dentin formation
New bone formation by recruiting host murine cells
|
|
PDLSCs
|
Periodontal ligament
|
CD9, CD10, CD13, CD26, CD29, CD44, CD59, CD73, CD90, CD105, CD106, CD146, CD166, STRO-1, Nanog, Oct-4, Sox-2, Rex-1, SSEA-1, SSEA-3, SSEA-4, TRA-1-60, TRA-1-81
|
CD14, CD34, CD45, HLA-DR
|
Osteogenic
Cementogenic
Adipogenic
Chondrogenic
Insulin-producing cells
|
Cementum/periodontal ligament structure
|
|
DFPCs
|
Dental follicle of developing tooth
|
CD9, CD10, CD13, CD29, CD44, CD53, CD59, CD73, CD90, CD105, CD106, CD146, CD166, STRO-1, Nanog, Oct-4
|
CD34, CD45, CD117, HLA-DR
|
Osteogenic
Adipogenic
Neurogenic
Chondrogenic
|
Mineralized tissue structure
|
|
SCAP
|
Apical papilla of developing tooth
|
CD13, CD24, CD29, CD44, CD73, CD90, CD105, CD106, CD146, CD166, STRO-1, Nanog, Oct-4
|
CD18, CD34, CD45, CD150
|
Odontogenic
Osteogenic
Adipogenic
Chondrogenic
Neurogenic
|
Dentin/pulp-like complex
|
|
GMSCs
|
Gingiva
|
CD13, CD29, CD44, CD73, CD90, CD105, CD146, STRO-1, Oct-4, SSEA-4
|
CD14, CD34, CD45, HLA-DR
|
Osteogenic
Adipogenic
Chondrogenic
Neurogenic
Endothelial
|
Connective-like tissue
|
|
NDP-SCs
|
Dental pulp of newborn
|
CD13, CD29, CD44, CD73, CD90, CD146, CD166, Nanog, Oct-4, Sox-2, Rex-1, FoxD3
|
CD14, CD34, CD45, HLA-DR
|
Osteogenic
Adipogenic
Chondrogenic
Myogenic
Neurogenic
|
Dental Pulp Stem Cells (DPSCs)
DPSCs Identification, Isolation, and Characterization
DPSCs from adult human dental pulp were first identified by Gronthos and colleagues in 2000 [7], even though the existence of stem cells in dental pulp has been reported by Yamamura in 1985 [58]. DPSCs were isolated on the basis of their high proliferation and frequency of colony formation that produced sporadic, but densely calcified nodules. The authors demonstrated that DPSCs could develop in vitro into odontoblasts, the cells that form the mineralized matrix of dentin. In addition, when transplanted into immunocompromised mice, DPSCs mixed with hydroxyapatite/tricalcium phosphate (HA/TCP) were able to generate a dentin/pulp-like complex with a collagen matrix containing blood vessels and lined with odontoblasts [7].
The collection of stem cells from dental pulp is a common noninvasive surgical practice that can be performed in the adult during life. Dental pulp tissue from human third molars represents the most common source for DPSCs harvesting, since these teeth are often extracted and discarded. In order to isolate dental pulp, the tooth has to be sectioned at the cementum/enamel junction using dental instruments. Then, dental pulp tissue is gently separated from the crown and root, and minced into small fragments. From these, DPSCs can be isolated either by the enzymatic digestion (ED) method [7, 59] or by the outgrow (OG) method [60, 61]. Using the first approach, the dental pulp is digested in a solution of collagenase type I and dispase in order to obtain single cell suspensions. Conversely, the second technique is based on the outgrowth of stem cells from tissue fragments. Huang and colleagues compared the properties of DPSCs isolated with the two techniques [62], finding higher proliferation rate for the digested cells than for those isolated by the OG method. In addition, according to Karamzadeh and co-workers, DPSCs isolated by ED showed higher mineralization capacity than those obtained with the OG method [63]. In a later work of Hilkens and colleagues, it has been demonstrated that, when DPSCs were derived from the same donors and kept under the same culture conditions, there were no differences in cellular morphology, proliferation rate, stem cell marker expression and mesenchymal differentiation potential, regardless of the isolation method used [64].
Increasing evidence suggests that DPSCs can survive for long periods and can be passaged several times. Suchanek and colleagues showed that DPSCs achieved 60 population doublings in culture medium designed for bone marrow [65], whereas Laino and co-workers accomplished 80 passages by maintaining the DPSCs substrate interaction and cell-cell communication in the central region of the secreted ECM [66]. DPSCs can also be cryopreserved and stored for long periods without losing their multipotential differentiation ability [66].
In the dental pulp, stem cells are thought to reside in a perivascular niche within the tooth structure, as demonstrated by the expression of the endothelium-associated marker CD146 in blood vessel walls, but not in the surrounding fibrous tissue, odontoblast layer, and perineurium of the nerve [32]. DPSCs also express STRO-1, which is considered an early marker of different mesenchymal stem cells (MSCs). Positive staining for both STRO-1 and CD146 has been widely used to identify DSCs niches. In particular, STRO-1 and CD146 were found to co-localize on the outer walls of blood vessel in dental pulp, thus implying that the majority of DPSCs arise from the microvasculature [32]. Since DPSCs are considered a population of MSCs, markers that have been used for identifying MSCs are also used for DPSCs. Apart from CD146 and STRO-1, DPSCs result positive to other stromal-associated markers, such as CD9, CD10, CD13, CD29, CD44, CD73, CD90, CD105, CD106, and CD166, and negative to hematopoietic markers, such as CD14, CD34, and CD45, and HLA-DR [23, 33]. Nevertheless, different works have reported positive expression for CD34 in DPSCs [66, 67]. In humans, CD34 identifies a cell surface antigen expressed by the most primitive stromal other than hematopoietic stem cells. The controversy concerning CD34 expression among studies can be attributed to the different experimental conditions, mainly the high serum concentration in culture medium, which has been found responsible for the rapid loss of the CD34 antigen during expansion [68]. Undifferentiated DPSCs also express Oct-4, Nanog, and Sox-2, and present the cytoskeletal proteins nestin and vimentin, all of which are characteristic of undifferentiated ESCs. Apart from stemness markers, DPSCs exhibit expression for a variety of markers associated with bone, such as alkaline phosphatase (ALP), collagen type I (COL1), osteonectin (ON), osteopontin (OPN), osteocalcin (OCN), and bone sialoprotein (BSP); smooth muscle, for example α-smooth muscle actin; and fibroblasts, including collagen type III (COL3), and fibroblast growth factor 2 (FGF2) [7]. The lack of expression of the odontoblast-specific dentin sialoprotein (DSP), which is restricted to the outer pulpal layer containing differentiated odontoblasts, implies that DPSCs represent an immature pre-odontogenic population [32]. It is important to remind that, like all MSCs, DPSCs are heterogeneous and the various markers listed above may be expressed by subpopulations of these stem cells [23].
Differentiation Potential of DPSCs
DPSCs are considered similar to MSCs not only because they share a fibroblastic morphology with selective adherence to solid surfaces and good proliferative potential, but also for their ability to differentiate into multiple cell lineages in vitro. After their original characterization, DPSCs have been differentiated into odontogenic, osteogenic, neurogenic, adipogenic, myogenic, and chondrogenic tissues.
Human DPSCs were initially identified on the basis of their differentiation into osteoblasts and odontoblasts, as previously reported [7]. It has been shown that the potentiality of DPSCs differentiation into odontoblasts in vivo is reduced after passage 9, when these cells can only differentiate along the osteoblast lineage [69]. A selected subpopulation of human DPSCs, called Stromal Bone Producing DPSCs (SBP-DPSCs) , has been further isolated by Laino and co-workers [66]. The authors obtained these cells from dental pulps of subjects between 30 and 45 years of age. The aged cells seemed not to be different from those found in younger patients, thus concluding that 30 years should not be considered as a critical age limit for DPSCs isolation. The authors found that SBP-DPSCs, which represent 10 % of dental pulp cells, displayed a great ability to differentiate into osteoblasts, producing a living autologous fibrous bone (LAB) tissue in vitro. When transplanted into immunocompromised rats, SBP-DPSCs formed a lamellar bone containing osteocytes. In this setting, SBP-DPSCs produced bone but not dentin, as shown by mRNA expression of bone markers including OCN, Runt-related transcription factor 2 (RUNX2), COL1, ALP, but not dentin sialophosphoprotein (DSPP), which is specific for dentin. In a later work of the same group, it has been observed that about 30 % of SBP-DPSCs become endothelial cells during their differentiation. These cells were found lining the vessel walls of the newly formed woven bone. Interestingly, a complete integration of vessels within bone chips took place after in vivo transplantation, leading to the formation of a vascularized bone tissue [34].
Although the majority of the studies have focused their attention on the ability of DPSCs to differentiate into odontoblasts or osteoblasts, further characterization revealed that DPSCs also possess adipogenic and neurogenic differentiation capacities [35, 36]. Remarkably, DPSCs are known to produce neurotrophic factors and even rescue motoneurons after spinal cord injury [37]. DPSCs were also found to undergo chondrogenic and myogenic differentiation in vitro. For example, Zhang and colleagues [38] demonstrated that human DPSCs were able to differentiate into neurogenic, osteogenic/odontogenic, adipogenic, myogenic, and chondrogenic lineages, when cultured under the appropriate inductive media. In a recent study of Paino and colleagues, it has been shown that DPSCs spontaneously differentiate in vitro toward the melanocytic lineage too [39]. The authors supposed that this spontaneous differentiation may be ascribed to the fact that both DPSCs and melanocytes arise from the neural crest cell population.
The ability of human DPSCs to differentiate into several cell lineages under defined conditions has been demonstrated also in a recent work [70]. DPSCs seeded onto a hyaluronan-based scaffold in the presence of a sequential cocktail of neuronal, glial, endothelial, and osteogenic factors proved to be a good strategy for the in vitro reconstruction of a dental pulp-like tissue. Once again, the plasticity and multipotential ability of DPSCs could be explained by the fact that dental pulp is made of both ectodermic and mesenchymal components, containing neural crest-derived cells.
Interestingly, Alongi and co-workers evaluated if DPSCs derived from Inflamed Pulps (DPSCs-IPs) could be used for dental tissue regeneration [20]. They found that DPSCs-IPs formed a dentin/pulp-like complex similar to healthy DPSCs when transplanted into immunocompromised mice. This is a very promising result since inflamed pulp, which is routinely discarded after pulpectomy, actually represents a possible source of stem cells.
Stem cells from Human Exfoliated Deciduous teeth (SHED)
SHED Identification, Isolation, and Characterization
Deciduous teeth is the formal name for what most people call baby teeth or, more colloquially, milk teeth. Children normally develop a set of 20 deciduous teeth, which appear after 6 months of life and generally are replaced, one tooth at a time, between age 6 and 13. The remaining crown of exfoliated deciduous teeth contains a living pulp remnant comprised of a normal dental pulp including connective tissue, blood vessels, and odontoblasts. Miura and colleagues were the first to identify in 2003 SHED from the remnant pulp in the crown of deciduous incisors of 7–8 years old children [24]. They isolated these clonogenic, proliferative cells using a technique very similar to the one Gronthos and co-workers used to isolate DPSCs [7]. When cultured under a neuronal differentiation medium, SHED formed sphere-like clusters in which highly proliferative cells aggregated together in clusters that either adhered to the culture dish or floated freely in the culture medium [24]. The dissociation of these sphere-like clusters allowed cells to grow as individual fibroblastic cells. This phenomenon suggested a high proliferative capacity analogous to that of neural stem cells. In effect, SHED are characterized by a higher proliferation rate and increased cell population doublings, which are faster than those of Bone Marrow MSCs (BM-MSCs) [24].
SHED can be isolated using the same procedures described for DPSCs. These stem cells offer attractive advantages over other postnatal DSCs, as they are derived from a source which is non-invasive, readily accessible, naturally being disposed, and with very limited ethical or legal concerns [71]. Bakopoulou and colleagues compared the morphology, growth, immunophenotype, and in vitro osteo/odontogenic differentiation characteristics of SHED obtained using the two isolation methods of ED and OG [67]. They found that the enzymatically digested cells displayed a significant heterogeneity comprising different cell sizes and morphologies even within the same colonies; on the contrary, SHED isolated with the OG method showed a uniform fibroblast-like morphology. The two types of cultures showed differences also in the immunophenotypic profiles and in the mineralization rate, which was lower in SHED obtained with the OG method compared to the digested SHED. Despite these observations, no differences could be detected in the growth rates of SHED isolated using the two methods.
Similarly to DPSCs, SHED express the two early MSCs surface markers STRO-1 and CD146. Cells positive to STRO-1 and CD146 were found to be located around blood vessels of the remnant pulp, implying that SHED may originate from a perivascular microenvironment [24]. SHED have also been identified as Immature Dental Pulp Stem Cells (IDPSCs) by Kerkis and co-workers [40]. Besides confirming the findings reported above, the authors established that IDPSCs express the ESCs markers Oct-4, Nanog, stage specific embryonic antigens (SSEA-3, SSEA-4), and tumorigenic recognition antigens (TRA-1-60, TRA-1-81). Further characterization revealed that SHED were uniformly positive for CD13, CD29, CD44, CD73, CD90, CD105, and CD166; and negative for CD14, CD34, CD45, and HLA-DR [41]. SHED were also found positive to several neural and glial markers, such as nestin, βIII-tubulin, glutamic acid decarboxylase (GAD), neuronal nuclei (NeuN), glial fibrillary acidic protein (GFAP), neurofilament M (NFM), and 2′,3′-cyclic nucleotide-3′-phosphodiesterase (CNPase), possibly due to the neural-crest cell origin of dental pulp [72].
Differentiation Potential of SHED
Under appropriate culture conditions, SHED have demonstrated the ability to undergo differentiation not only into odontogenic, osteogenic, neurogenic, and adipogenic [24], but also myogenic and chondrogenic cell lineages [40, 42].
Miura and colleagues observed that, when SHED were transplanted with HA/TCP particles into immunocompromised mice, they were able to differentiate into odontoblasts and were immunoreactive to DSPP. Nevertheless, these cells failed to generate a complete dentin/pulp-like complex, as do DPSCs in vivo [24]. Additionally, the same authors observed that SHED could not be differentiated directly into osteoblasts; rather, they were able to induce new bone formation by recruiting host murine cells [24]. This is not a property attributed to DPSCs following transplantation in vivo. The osteoinductive potential of SHED was demonstrated in a further study, in which these cells were found able of repairing critical-size calvarial defects in immunocompromised mice [43]. Together, the potential for odontogenic differentiation and the high osteoinductive potential in vivo suggest that SHED may represent a population of multipotent stem cells that are more immature than DPSCs. This is not surprising since deciduous teeth are significantly different from permanent teeth with regards to their developmental processes, tissue structure, and function [42].
As reported above, SHED readily express a variety of neural cell markers under non-neuronal inductive conditions [24]. When stimulated with neurogenic medium, Miura and colleagues observed that expression of βIII-tubulin, GAD, and NeuN increased, whereas expression of the other neural markers remained unchanged. Under these conditions, SHED lost their typical fibroblastic morphology and developed multicytoplasmic processes. Neural developmental potential was further studied in vivo by injecting SHED into the dentate gyrus of the hippocampus of immunocompromised mice. These studies showed that SHED survived for more than 10 days inside the mouse brain microenvironment and continued to express both neuronal and glial cell markers [24]. Koyama and co-workers reported the differentiation of SHED into the adipogenic and chondrogenic lineages in vitro [42]. The adipogenic differentiation of SHED was detected by the in vitro accumulation of lipid droplets, and by the expression of adipogenic markers such as Peroxisome proliferator-activated receptor-gamma (PPARG), and Lipoprotein lipase (LPL). The comparison between SHED and DPSCs revealed a higher adipogenic differentiation capacity of SHED than DPSCs. SHED were also shown to express the chondrogenic markers Sox-9, collagen type II (COL2), and collagen type X (COL10) when cultured for 14 days with bone morphogenic protein 2 (BMP-2), a chondrogenic signaling protein in the transforming growth factor β (TGFβ) family [42].
The strong differentiation plasticity of SHED was demonstrated in engraftment studies in vivo. In particular, Kerkis and co-workers observed that, when IDPSCs were transplanted into immunocompromised mice via intraperitoneal injection, they engrafted into the lungs, liver, spleen, brain and kidney, producing a tissue that was indistinguishable from the host tissue for liver, spleen, brain and kidney [40].
All these observations suggest that deciduous teeth could represent an ideal source of stem cells for repairing damaged tooth structures, inducing bone regeneration, and possibly treating neurodegenerative diseases.
Periodontal Ligament Stem Cells (PDLSCs)
PDLSCs Identification, Isolation, and Characterization
The periodontal ligament, one of the highly specialized and complex connective tissues of the human body, is embedded between the cementum and the inner wall of the alveolar bone socket. Periodontal ligament not only has an important role in supporting teeth, but also contributes to tooth nutrition, homoeostasis, and repair of damaged tissue [73, 74]. Periodontal ligament contains heterogeneous cell populations that can differentiate into either cementoblasts or osteoblasts. The presence of multiple cell types within periodontal ligament has led to speculation that this tissue might contain progenitor cells responsible for maintenance of periodontal ligament homoeostasis and regeneration. In effect, PDLSCs were identified in the periodontal ligament of extracted teeth by Seo and co-workers in 2004 [25]. These cells have been reported to form adherent clonogenic clusters of fibroblast-like cells, and had a proliferation rate similar to DPSCs after 24 h in culture.
PDLSCs can be obtained from periodontal ligament tissue after its separation from the root surface of extracted third molars [25]. Tran and co-workers compared the biological properties of PDLSCs isolated using the OG or ED methods [75]. Cells showed a similar morphology, mainly polygonal with expansion of the cytoplasm, regardless of the isolation protocol used. Additionally, the two isolation methods did not influence the proliferation rates, as well as the immunophenotypic profiles of PDLSCs. Regarding the osteogenic differentiation potential, PDLSCs obtained with the OG method produced a minor total area of mineralized nodules compared to that generated by the enzymatically digested cells.
In the work of Wang and colleagues it has been found that periodontal ligament possesses asymmetrically distributed stem cells, and that PDLSCs properties vary depending on the harvest location [76]. Cells isolated from the alveolar bone surface exhibited strong proliferation capability, and they were shown able to better regenerate the alveolar bone when compared to cells obtained from the root surface.
PDLSCs are similar to other MSCs with respect to the expression of STRO-1 and CD146 markers, implying that these cells might also be derived from a population of perivascular cells. In effect, McCulloch and co-workers have previously identified progenitor cells in the perivascular region of the periodontal ligament of mice [77]. Later, Chen and colleagues localized small clusters of PDLSCs also in the extravascular areas and regions near the cementum [78]. Differently from DPSCs and SHED, PDLSCs were found to express high levels of scleraxis, a tendon-specific transcription factor associated with tendon cells [25]. This is not surprising as the dense collagen fiber structure of the periodontal ligament makes it similar to the tendon. Further characterization revealed that PDLSCs express the following cell surface markers: CD9, CD10, CD13, CD26, CD29, CD44, CD59, CD73, CD90, CD105, CD106, and CD166. Like DPSCs and SHEDs, PDLSCs do not express CD14, CD34, CD45, and HLA-DR [33]. Different works demonstrated that PDLSCs also express ESCs markers, including Oct-4, Nanog, Sox-2, Rex-1, SSEA-1, SSEA-3, SSEA-4, TRA-1-60, and TRA-1-81 [44, 45], and a subset of neural crest markers, such as nestin, slug, p75, and Sox-10 [46].
Differentiation Potential of PDLSCs
PDLSCs were shown to be multipotent, having the ability to differentiate into osteoblasts, cementoblast-like cells, adipocytes, chondrocytes, and collagen-forming cells [25].
The osteogenic potential of PDLSCs was demonstrated through their ability to form a mineralized matrix containing calcium deposits after 14 days of exposure to osteogenic differentiation medium [47]. However, unlike BM-MSCs and DPSCs, these deposits were sparsely distributed in the culture. Under osteogenic induction medium, PDLSCs showed increased protein expression of the osteoblastic/cementoblastic markers ALP, BSP, OCN, and TGFβ receptor I. Despite the expression of these osteogenic/cementoblastic markers in vitro, PDLSCs were unable to form dentin or bone in vivo [25]. Nevertheless, PDLSCs loaded onto a HA/TCP-scaffold could give rise to a cementum/periodontal ligament structure when transplanted into immunocompromised mice and rats [25]. In particular, a thin layer of cementum-like tissue was formed along with condensed collagen fibers with sparse cells resembling periodontal ligament structure. In 2012, Song and co-workers compared stem cells derived from the periodontal ligament of permanent teeth (pPDLSCs) to those obtained from deciduous teeth (dPDLSCs) [79]. There were no significant differences in the proliferation rate, expression of stem cell markers, or in vitro differentiation between the two cell populations. Nevertheless, pPDLSCs generated more cementum/periodontal ligament-like structures in vivo than dPDLSCs , becoming better candidates for use in periodontium regeneration. In a recent work of Park and colleagues, PDLSCs from human healthy and inflamed periodontal ligament tissues were isolated and compared for their regenerative potential [22]. The proliferative potential did not differ between the two cell populations, and both of them were successfully able to differentiate under osteogenic/cementogenic and adipogenic culture conditions. Both cell types exhibited new cementum-like tissue and related periodontal ligament fiber regeneration after in vivo transplantation.
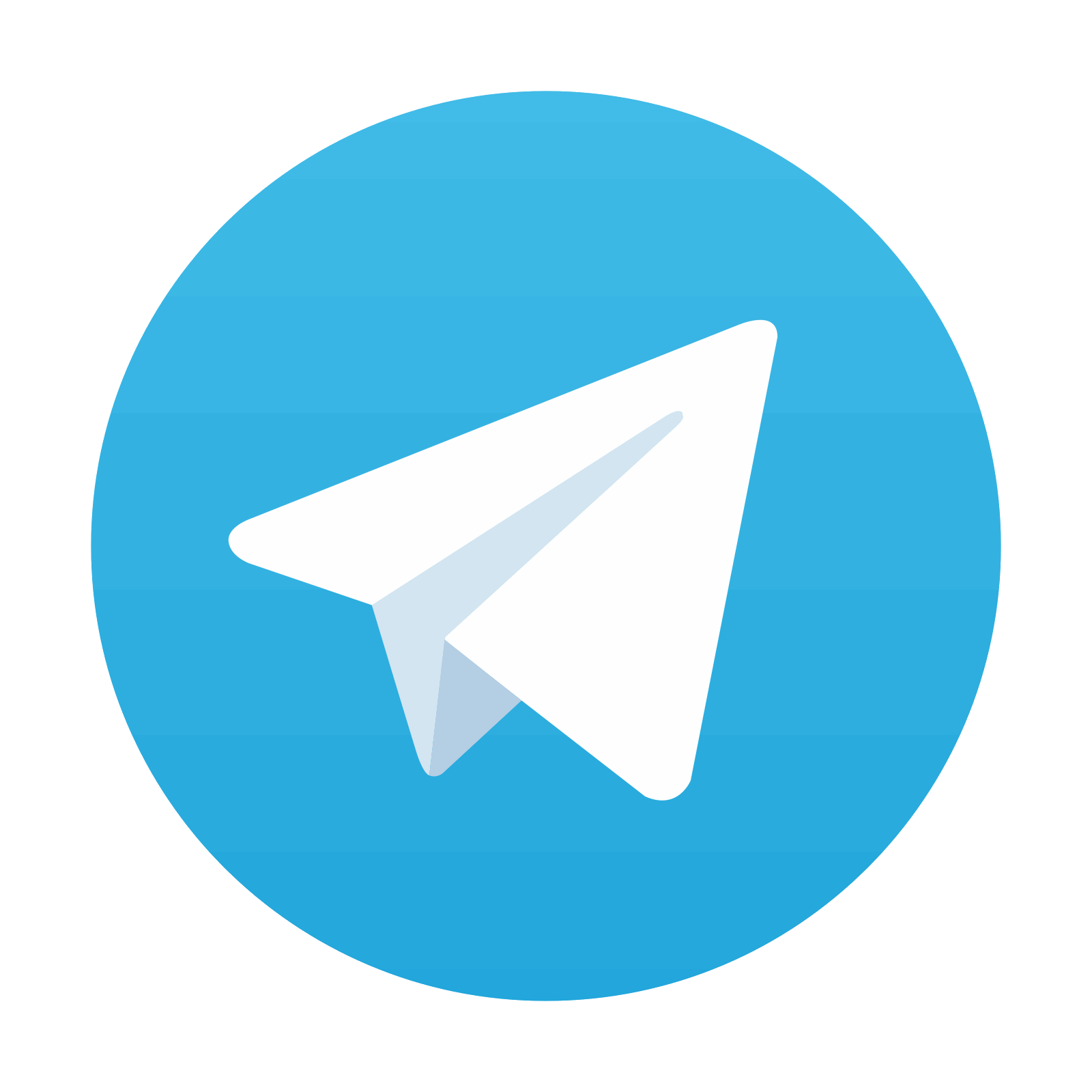
Stay updated, free dental videos. Join our Telegram channel

VIDEdental - Online dental courses
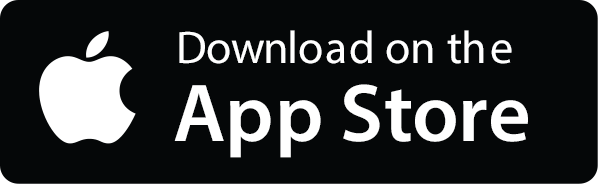
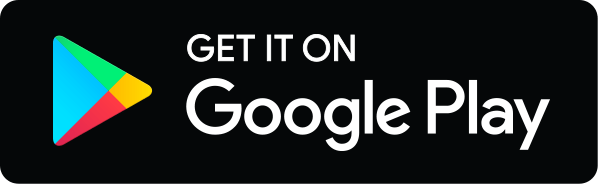