Dental pulp collagens
Type I collagen
56 %
Type III collagen
41–42.6 %, associated with fibronectin
Type V collagen
2 %
Type VI collagen
Thin fibrils -100 nm periodicity
Fibrillin-containing microfibrils
Phosphorylated non-collagenous ECM proteins
Small integrin-binding ligand N-linked glycoproteins (SIBLINGs)
Dentin sialophosphoprotein (DSPP)
Cleaved into dentin sialoprotein (DSP) and dentin phosphoprotein (DPP)
Dentin matrix protein 1 (DMP1)
Bone sialoprotein (BSP)
Osteopontin
Matrix extracellular phosphoglycoprotein (MEPE)
Non-phosphorylated ECM protein
Osteocalcin
Osteonectin (secreted protein, acidic and rich in cysteine –SPARC)
Tenascin
Fibronectin
Glycosaminoglycans and proteoglycans
Small leucine-rich proteoglycans (SLRPs)
Class I: CS/DS PGs – decorin and biglycan (collagen fibrillogenesis)
Class II: KSPGs – fibromodulin and lumican (collagen fibrillogenesis)
Keratocan and PRELP osteoadherin
Class III: CS/DS/KS. Epiphycan and mimecan/osteoglycin
Large GAGs and proteoglycans
Versican
GAG chains
Cellular and pericellular pulp molecules
Growth factors
Hepatocyte growth factor
Fibroblast growth factor 2
TGF beta 1
Tumor necrosis factor α
Lymphocyte enhancer-binding factor 1 (LEF1)
Bone morphogenetic protein 2 (BMP2)
Receptors of growth factors: Smad1, Smad7, BMPs
Enzymes
Alkaline phosphatase (nonspecific alkaline phosphatase, TNAP)
Acid phosphatases
Metalloproteinases
Collagenases: MMP-1, MMP-8, MMP-13
Collagenase inhibitors
Disintegrin and metalloproteinase 28 (ADAM28)
Cysteine cathepsin
Dipeptidyl peptidase II
PMN elastase
Cathepsin G
Matrilin-2, matrilin-4, CD44, interleulin-1 β
3.2 Isolation of the Dental Pulp
The dental pulp is comprised of fibroblast-like cells and mesenchymal stem cells (MSC) as well as more specialized cells and the regulatory factors they secrete. These reside in a more or less typical loose highly hydrated viscous extracellular connective tissue matrix rich in hyaluronan, glycosaminoglycans, and proteoglycans all held together in a network of thin collagen fibrils, reticular fibrils, and fibronectin. The pulp becomes highly vascularized [5, 6] and the vascular bed serves both as the pathway for entry of circulating factors produced elsewhere, and for the removal of metabolic degradation products. The coronal pulp cavity is also richly innervated [7]. The pulp fibrillar network gives the pulp a fixed shape and it can be removed intact if the confining mineralized dentin is carefully removed. Van Amerongen et al. [8] collected the pulp from freshly extracted human teeth, which were washed with 4 °C water, then immediately rinsed and frozen to −20° with no further treatment. A diamond disc saw, cooled with 4 °C water, was used to cut each tooth along the lingual, buccal, and both occlusal sides without entering the pulp. The teeth were then split with a chisel along each cut to expose the pulp. The intact pulp could then be plucked out with tweezers retaining its hydrated shape. Alternatively, an intact premolar could be cut into three portions perpendicular to the incisal edge-apical root direction, yielding a clear coronal portion cut at the cervical line, “limited by the cemento-enamel junction,” and two radicular sections of equal length representing the middle radicular pulp and the apical section (Fig. 3.1a, b).
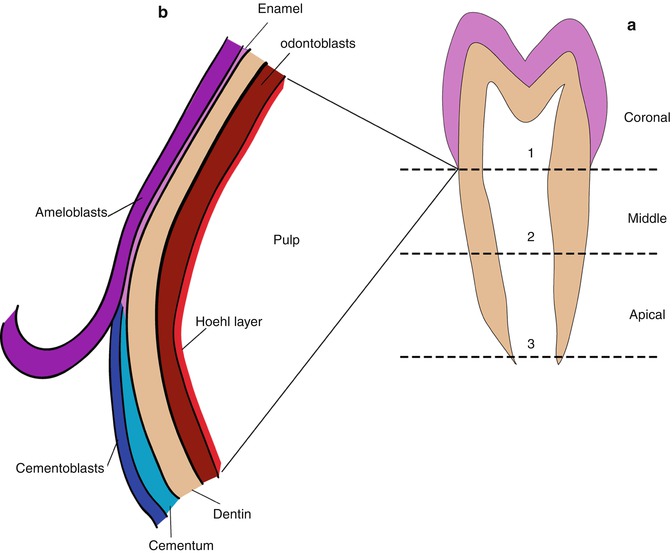
Fig. 3.1
(a) The plan for separating pulp zones in a human premolar tooth according to van Amerongen et al. [8]. Cuts are made along each of the dashed lines. Line 1 is at the cervical line, line 3 is at the tooth apex, line 2 is midway between 1 and 3. The pulp has sufficient mechanical strength so that the pulp segments can be plucked from the surrounding dentin with tweezers. Although there are no physical barriers, the three pulp segments have, on average, different structures and significantly different compositions. (b) Detail of the arrangement of the structures at the cervical line early in development. The ameloblasts in the IEE stop secreting enamel meet and form a tight junction with the cementoblasts and developing cementum layer, which covers the root dentin. The dentin–enamel junction (DEJ) is replaced by cementum–dentin junction (CEJ). However, the thin, approximately 4-cell thick layer of loosely arranged Hoehl’s cells remains interfaced between the dentin, odontoblasts, and the dental pulp cells. Hoehl’s cell region, depicted in red, is densely vascularized. The reciprocal enamel–dentin and cementum–dentin signaling interactions are probably also communicated locally and reciprocally through Hoehl’s cells to the pulp cells accounting for the differences seen in the compositions of the different pulp regions
The collection of the pulp via mechanical removal and the definition of the pulp as not including the dentin–odontoblast complex odontoblasts leave open the question of the sharpness of the mechanical separation. In the coronal region, the odontoblastic processes go deeply into the dentinal tubules, in some cases 2/3 of the total tubule length. Byers and Sugaya [9] used a fluorescent carbocyanine dye, Di-I (1,1′-dioctadecyl-3, 3,3′,3′ tetramethylindo-carbocyanine perchlorate), to stain the organelles and cytosol within the dentinal tubules. While there was no specificity to the staining, the packing of the individual tubules was readily seen, as was the high variability of the length of penetration of the odontoblast processes within the tubules. Control experiments were conducted to verify that the staining was in the processes and not in the surrounding dentin. The density, length, degree of convolution, and extent of branching of the tubules was different in the coronal, central, and apical radicular regions. In our experience mechanical removal of the pulp is not entirely clean, some odontoblasts or odontoblast precursors (the Hoehl’s cells) may adhere to the extracted pulp, or some of the contents of the torn and contracted odontoblast processes may bind to the pulp surface in spite of the macroscopically clean surface appearance. Thus, locally the composition of the pulp at the pulp-odontoblast interface may appear to be artifactually high in type I collagen and associated components [10].
3.3 Composition of the Dental Pulp: Type III Collagen, Type V Collagen
The composition of the intact premolar pulp was then compared to the compositions of the three sub-compartments of similar teeth. It was also possible to compare the compositions of equivalent premolar and more mature third molar pulps. There was a statistically significant larger content of collagen on a percentage dry weight basis in the third molar pulp than in the premolars. But the collagen content, based on hydroxyproline determinations, was not the same within the premolar pulp fractions. The radicular middle and apical sections had significantly higher contents of total collagen than the coronal pulp. DNA analysis of the coronal and apical pulp sections showed significantly higher DNA content (cellularity) than did the intervening middle radicular section. Very little of the collagen was soluble in neutral salts or 0.15 M acetic acid, but pepsin digestion did yield soluble collagen. SDS-gel electrophoresis of the pepsin soluble collagen showed a major peak at the 3-chain γ-component position, and that peak was converted to a mixture of α and β chains upon the addition of DTT. Differential denaturation and renaturation experiments [11] showed that the γ-component was essentially type III collagen, and this was confirmed by analysis of the compositions of the cyanogen bromide peptides [12]. By comparing the area under the well-resolved α1(III)CB3,6,8 peak to the total integrated areas of all peaks, it was determined that type III collagen comprised ~42.6 % of the total pulp collagen, a surprisingly high concentration compared to the <3 % found as type III in mature skin and bone collagens. Lechner and Kalnitsky [13] and Tsuzaki et al. [14] subsequently showed in detail that the same was true in bovine dental pulp obtained from more mature molars and in teeth with multiple roots. The more detailed isolation of the collagens from bovine pulps showed overall compositions of 56 % Type I, 41 %Type III, and 2 % Type V. The small amount of type V was heterogeneous: a mixture of two isoforms of Type V, [α1(V)2 α2(V)1], and [α1(V) α2(V) α3(V)]. The type III molecule was present as high molecular weight aggregates, which could be disassociated by treatment with 2-mercaptoethanol to yield α1(III) chains, indicating that the type III molecules were disulfide-linked homotrimers. Unfortunately, the more recent papers quantitating the relative contents of the pulp type I and III collagens all used whole homogenized pulps rather than the coronal and apical portions used by Amerongen et al. [8]. Thus, there is little fresh information concerning the differences in the type III contents in the apical and coronal pulp domains. Nevertheless, the analytical methodologies used by Amerongen et al. were essentially equivalent to those used later and there is no reason to dispute the regional composition differences reported in the direct and simultaneous comparison of pulps from different regions. In fact, perhaps, that should have been expected based on the fact discussed earlier that the pulp cells, including pulp-MSCs, are probably subjected to different ratios of factors stimulating MSC gene expression in different parts of the cervical and apical regions, and the recent findings that ectomesenchymal stem cells derived from the pulp (DP-MSC) are most effective and active [15–17]. In particular, DP-MSCs demonstrated in culture the highest osteogenic potential compared with that of bone marrow-derived, adipose tissue-derived and umbilical cord-derived MSC’s [17], and increased in activity with higher passage numbers, whereas the BM-MSC, AD-MSC and UBC-MSC cultures decreased in activity with increasing cell passage. Thus, DP-MSCs increase in activity with tissue aging, whereas the others decrease in activity with aging. Stanko et al. [17] noted that Snail, a zinc finger transcriptional repressor that downregulates ectodermal genes within the mesoderm, including E-cadherin, is expressed at lower levels in the DP-MSCs. The lower expression of Snail allows the E-cadherin level to remain high and favors the epithelial-mesenchymal transition so important for tooth development and osteogenic induction. Bone morphogenic protein BMP 4 is also higher in pulp than in bone and adipogenic tissues.
When type III was discovered to be present in small amounts in mature tissue and somewhat larger percentages were present in fetal tissues it was thought that type III, with its thin fibrils and different cross-linking chemistry when compared to type I collagen, was confined to a transient role with its expression decreasing with age. That is obviously not the case within the dental pulp, where type III collagen remains a major component in mature pulp ECM, suggesting that it may be important in maintaining the pulp structural integrity by stabilizing the highly hydrated network of thin reticular type III collagen fibrils in the ECM. Type VI collagen microfibrils with a characteristic periodicity of about 100 nm have also been detected, appearing as long thin and flexible filaments. They aggregate by lateral association. Microfibrils of 10–14 nm in diameter contained fibrillin. Fibrillin-containing microfibrils were abundant in the newly formed pulp [18]. The collagen network associates thin fibrils and fibers with a larger diameter. It has been estimated that about 14 % of the volume of the pulp is made up of blood vessels [19] but the rate of blood flow is different in different regions. The blood flow in the coronal half of a pulp is about twice that in the apical half.
3.4 Other Structural Proteins of the Dental Pulp
Dental pulp cells in culture can, depending on the culture conditions and the factors added to the culture, produce almost all of the ECM proteins found in other tissues, but only a few are present in normal pulp tissues. Of these fibronectin is a principal noncollagenous protein of the dental pulp, associated principally, but not exclusively, with type III collagen in argyrophillic fibrils. Fibronectin (FN) is abundant in the odontoblast layer around blood vessels and in the core of the pulp. A fucosylated glycoprotein, fibronectin is a disulfide linked dimer of two similar ~220,000 mass subunits. Other species of glycoproteins were also detected. Fibronectin is also associated with proteoglycans (PGs). The molecule is also detectable as the free dimer in the water extract of the pulp (0.030 %), in the collagenase extract (0.094 %), and in the hyaluronidase extract (0.109 %). The reappearance of collagen type III is parallel to the advanced vascularization of the dental papilla. The expression of fibronectin is dependent of the calcium-ions dose, but not on magnesium ions [20]. Twice as much fibronectin was extracted from the apical pulp as from the coronal and middle parts, in accord with the higher concentration of type III collagen also found in the apical region, suggesting a 1 to 1 collagen III to fibronectin complex formation.
3.5 Non-collagenous Proteins
3.5.1 The Phosphorylated Proteins, SIBLINGS
The small integrin-binding ligand N-linked glycoprotein family (SIBLING) is a family of phosphoproteins, present in dentin, in bone, and in many pathologic mineralized tissues (kidney, salivary glands, tumors etc.). Immunostaining of the SIBLING molecules shows much lower reactions in the pulp compared with the dentin staining, but they still remaining at a detectable level.
Among the phosphorylated NCP, dentin sialophosphoprotein (DSPP) is the most abundant and is a specific factor of odontoblast differentiation and dentin mineralization. Just after the molecule is released in the tissue, DSPP is proteolytically cleaved into dentin sialoprotein (DSP) and dentin phosphoprotein (DPP). BMP-1 cleaves DSPP to form DSP. Matrix metalloproteinases, MMP-2 and MMP-20, are implicated in cleavage of the COOH-terminal portion of DSPP yielding DPP. BMP-1/Tolloid-like metalloproteinases are also implicated in this cleavage. There is no evidence that DPP, the highly phosphorylated component created in the same molar concentration as DSP, has any bearing on the potential stimulation of pulp matrix mineralization.
The 53 kDa sialic acid-rich glycoprotein DSP, the NH2-terminal portion cleavage product of DSPP, is synthesized by dental pulp cells. It may be an effective stimulator for dental pulp stem/progenitor cell differentiation, dental tissue repair, and pulp regeneration [21], but it does not appear to be involved in pulp matrix mineralization as implicated in the dentin matrix.
Dentin matrix protein 1 (DMP1) is another SIBLING found in the dental pulp. It may be mutated, but in contrast with mild dentin defects, there is no apparent change in the pulp structure. However, deletion of the DMP-1 gene shows a postnatal failure of maturation of predentin into dentin, accompanied by an increased width of the predentin but reduced width of the dentin also leading to enlarged pulp chambers. This tooth phenotype is very similar to the human dentinogenesis imperfecta. Altogether this demonstrates that DMP-1 is essential for the late dentinogenesis development especially during the postnatal period [22].
Bone sialoprotein (BSP) is the sialylated bone analog of DSP. Some is expressed by pulp cells and has been detected by immunohistochemistry and in situ hybridization (ISH) in the pulp. Pulp capping with BSP leads to the formation of a robust homogeneous mineralized reparative dentinal bridge [23].
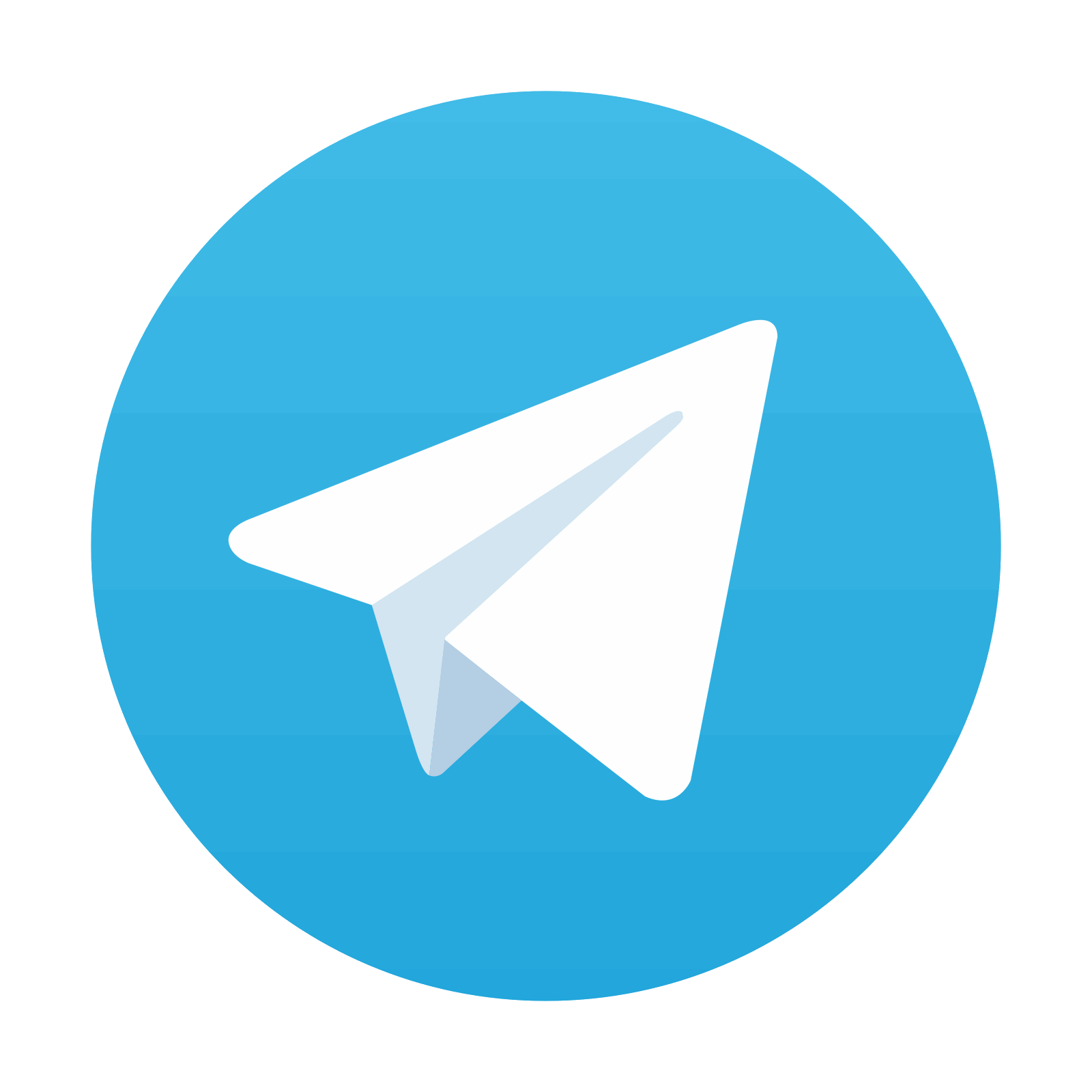
Stay updated, free dental videos. Join our Telegram channel

VIDEdental - Online dental courses
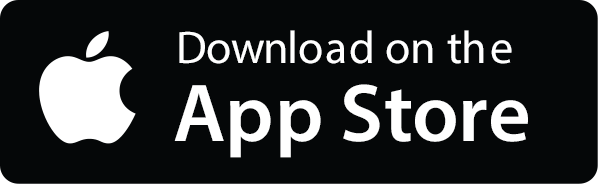
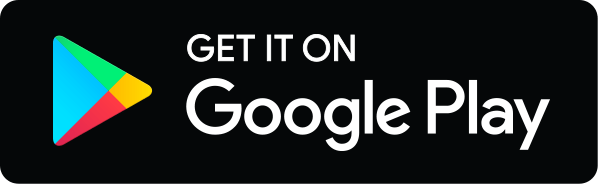