Stem cells permanently present in the adult tooth
Dental pulp stem cells (DPSCs) isolated from the pulp of permanent teeth
Exfoliated deciduous teeth stem cells (SHEDs) and immature dental pulp stem cells (IDPSC) from deciduous teeth
Apical papilla stem cells (SCAPs)
Stem cells present in periodontal tissues
Periodontal ligament stem cells (PDLSCs)
Dental follicle stem cells (DFSCs), differentiating in odontoblast-like cells or endothelial cells
SM taking origin from other tissues
Induced pluripotent stem cells (iPS)
Hematopoietic stem cells (HSCs)
Neuronal stem cells (NSCs)
16.3.1 Stem Cells Permanently Present in the Adult Tooth
Adult human dental pulp stem cells (DPSCs) are derived from the pulp of human permanent third molar. They take origin from the neural crest cells (NCCs), which are multipotential cells, migrate, and contribute to different tissues ranging from the peripheral nervous system to the craniofacial skeleton. Pre-migratory cells display plasticity. The cranial NCCs give rise to the majority of the bone and cartilage of the head and face [25].
The relative ease of access of the pulp to autologous use renders attractive the dental pulp stem cells and/or human exfoliated deciduous teeth stem cells (DPSC/SHED) as a valid option in regenerative dentistry [26].
In the dental pulp, DPSCs have been found both in adults and in children, in the superficial “cell-rich zone,” located beneath the Hoehl’s cell layer. They take origin from the neural crest and may differentiate into multiple cell lineages. Under specific stimuli, they differentiate into adipocytes, neurons, chondrocytes, and mesenchymal stem cells.
Adult DPSCs and SHEDs may regenerate a dentin-pulp-like complex. DPSCs form sporadic densely calcified nodules in vitro. When the stem cells are transplanted with hydroxyapatite/tricalcium phosphate into immunocompromised mice, a dentin-like structure is formed within 6 weeks, lined by odontoblast-like cells, with cellular processes into a mineralized matrix [27, 28]. Furthermore, SHEDs are able to induce differentiation into bone-forming cells. SHEDs have a higher proliferation rate compared with DPSCs of permanent teeth. They are easy to expand in vitro. They have high plasticity and may differentiate into neurons, adipocytes, osteoblasts, and odontoblasts [29].
Compared with human bone marrow stromal stem cells (BMSSCs), known as osteoblast precursors, DPSCs share similar phenotype in vitro, but they produced only sporadic densely calcified nodules. They did not form adipocytes, whereas BMSCs form adherent cell layer with clusters of lipid-loaded adipocytes. DPSCs transplanted into immunocompromised mice generated a dentin-like structure lined with human odontoblast-like cells surrounding a pulp-like structure. BMSCs formed lamellar bone-containing osteocytes and surface-lining osteoblasts. Therefore, DPSCs have the ability to form a dentin-pulp-like complex [30].
Other groups of cells issued from dental tissues may contribute as a source of odontoblasts; this is the case for the stem cells from the apical papilla (SCAP). According to Huang et al. [31], these cells seem to be responsible for the continuous formation of the root dentin. They are involved in “bioroot engineering.”
16.3.2 Stem Cells Taking Origin from Periodontal Tissues and from Other Tissues
In addition, mesenchymal cells originating from the dental surrounding tissues may differentiate and be used for tooth regeneration. Stem cells of the apical part of the papilla (SCAPs), stem cells from the dental follicle (DFSCs), periodontal ligament stem cells (PDLSCs), and bone marrow-derived mesenchymal stem cells (BMSCs) may be also a source of stem cells that may be implicated in tooth regeneration [32]. Perivascular multilineage progenitor cells and migrating mesenchymal stem cells may contribute to pulp regeneration. Adipose–derived stromal cells (ADSCs) contain a group of pluripotent mesenchymal stem cells. These cells manifest multilineage differentiation capacity. They exhibit stable growth and proliferation kinetics in vitro. They express bone marker proteins including alkaline phosphatase, type I collagen, osteopontin, and osteocalcin and produce mineralized matrix. Therefore, dental and non-dental stem cells share the following properties: high proliferation rate, multi-differentiation ability, and easy to be induced. They may be instrumental in tissue-engineered organ replacement [29, 30, 33–36].
16.4 Niches of Stem Cells
Stem cells are located in particular microenvironments known as niches. Schofield introduced the concept of a stem cell “niche” in 1978 [37]. The niche encompasses all of the elements immediately surrounding the stem cells, which are in their naïve state. This imaginary space includes the non-stem cells, which are in direct contact with the stem cells, as well as ECM and soluble molecules found in that location [38].
Stem cell behavior is regulated by a local microenvironment referred as “the stem cell niche” characterized by three essential properties: (1) A niche provides an anatomic space where the number of stem cell is regulated; (2) it is the place where stem cells are instructed to control the maintenance, quiescence, self-renewal, and recruitment toward differentiation, fate determination, and long-term regenerative capacity; (3) the niche will influence the cell motility [39]. Hallmarks of a niche include the stem cell itself, stromal supporting cells that interact directly with the stem cells via secreted factors, and cell surface molecules. The extracellular matrix (ECM) provides a structural support to the niche and allows the diffusion of mechanical and chemical signals. Systemic signals are implicated in the recruitment of inflammatory and circulating cells into the niche.
In the teeth, as in the adult blood system, multiple niches may exist; however, specific markers allowing the definitive identification of stem cells within the pulp are still lacking. Specific niches have been identified in the pulp. Niches provide unique microenvironments that regulate stem cells. Most investigations are related to the basal forming part of the rodent incisor, a situation that does not apply to the teeth of limited growth as they are found in humans.
In the rodent incisor, the production of progenitor cells is under the control of molecular signals such as Notch1, Lunatic fringe, and fibroblast growth factor-10 expressed in the apical bud, both in the surrounding mesenchyme and in the epithelium [40]. FGFR2 signaling axis maintains the stem cell niche required for incisor development and lifelong growth [41].
In the incisor, the term “apical bud” indicates a specialized epithelial structure, whereas in the guinea pig molars, which are continuously growing teeth, specific proliferative regions were identified, implicated in the formation of “apical bud” at the apical end [42].
The neuronal marker expressions of undifferentiated DPSCs are α-III-tubulin, S100 protein, and synaptophysin. A subset of the population showed a positive immunolabeling for galactocerebroside, neurofilament, and nerve growth factor receptor p75. Immunolabeling of young dental pulp tissue demonstrated the presence of a perivascular cell niche and a second stem cell niche at the cervical area. In adult dental pulp only the perivascular niche could be observed [43].
Lizier et al. [20] compared stem cells at early passages (2–5 passages) to a late population (6 months after several transfers). No morphological difference was noted between the two periods. It was the same for the expression of stem cell markers (nestin, vimentin, fibronectin, SH2, SH3, and Oct ¾ and chondrogenic, myogenic, and differentiation potential). Bromo-2′-deoxyuridine (BrdU)-positive cells were observed in the central part of the dissected pulp after 6 h, whereas after 72 h, BrdU-positive cells increased in number and were located at the pulp periphery. This suggested a migration of labeled cells from the central pulp toward the outer pulp border. Multiple niches were identified expressing nestin, vimentin, and Oct3/4, while STRO-1 protein localization was restricted to perivascular niche.
Perivascular niches were identified as expressing STRO-1-positive markers, identical to human bone marrow stromal stem cells (BMSSCs) and dental pulp stem cells (DPSCs). They were negative for von Willebrand factor and positive for α-smooth muscle actin and CD146. DPSCs expressed the pericyte marker 3G5 [44]. However, this localization is still controversial, and the lack of perivascular immunolabeling with an α-smooth actin antibody does not corroborate such assumptions [45].
In the primate molars, which are the teeth of limited growth, cervical class V cavities were prepared. After pulp exposure, pulp capping was carried out. The monkey received a single injection of 3H-thymidine. Six, 8, and 12 days after the treatment, the teeth were extracted and the number of cells labeled after radioautography was scored. Labeled cells were seen first in the deeper central pulp. Two cell replications were dependent on the timing of the injection of tritiated thymidine. Injected 84 and 96 h after capping, for the shorter period cells were radiolabeled in the central pulp, whereas the second injection occurring after a longer period of time was mostly located beneath the Hoehl’s cells and odontoblast layers. It was shown that odontoblasts are clearly postmitotic cells, never labeled after such procedure. Replacement cells differentiate into functioning odontoblast-like, and a continuous influx of differentiating cells was evidenced. They have initially replicated their DNA in the deep zone of the pulp. Then they migrate toward the terminal migration point, beneath the wounded odontoblasts, where the terminal duplication occurred. Hence, fibroblast-like cells, perivascular cell population, and progenitor cell are candidate to participate to the continuous influx of new candidates as stem cells [46].
Although this pioneer publication shed some light on the renewal and migration of cells within the dental pulp, it is noteworthy that (1) only two time points were taken into account, and therefore a more detailed kinetic study is still needed; (2) the cell migration phenomenon was partially elucidated in the crown, (3) but this was not the case for the root.
16.5 The A4 Clone Induces “In Vivo” the Formation of Reparative Dentin
Our aim was to explore for the first time the potential of pulp-derived “stem” cells to improve dental repair upon injury. In order to characterize the dental pulp progenitors and elucidate the molecular and cellular mechanisms governing their differentiation, we used clonal cell lines obtained in our laboratory from tooth germs of day 18 mouse embryos transgenic for an adenovirus-SV40 recombinant plasmid (pK4) [47]. Among the 50 clones obtained from the molar dental pulp, the clone named A4 represents a homogenous population of precursor cells which display in vitro properties of multipotent mesoblastic cells [3], which after differentiation were shown to mineralize.
Moreover, our in situ approach was essential since the definition of a “progenitor” implies an attempt to outline its in vivo potential into a repair/regeneration context.
16.5.1 In Vivo Experimental Cell Implantation
16.5.1.1 Implantation into the Mouse Incisor
In an in vivo pilot study, the A4 cells were implanted in the mouse mandibular incisor, and this led to the formation of a mineralized osteodentin within the dental pulp. Since a mouse incisor is very small and its diameter is about the same as the smaller dental burr, lateral perforation occurred establishing uncontrolled and undesirable contact between the dental pulp and the periodontal environment. The incisor is a continuously growing tooth. As a result from the continuous tooth eruption, the damaged tissue was moved forward. Serial sections allowed identifying a newly formed dental tissue in the basal part of the incisor, the lesion area itself and what was formed in the distal part of the tooth before the pulp exposure. As the results were inconclusive, it was necessary to choose another model closer to the human tooth with a limited growth. This is why another set of experiments was carried out on rat’s molar.
16.5.1.2 Implantation into the Rat Molar
The small size of the mouse molars makes such experimental procedures difficult, whereas the rat molar provides an easier experimental approach involving the preparation of a cavity on the mesial aspect of the teeth. The initial protocol was carried out on the first maxillary molar and used to study the successive events leading to the formation of reactionary dentin [48]. We modified slightly the protocol, and we too used the first maxillary molar of rat to explore the feasibility of a pulp capping therapy after a surgical exposure. Bioactive molecules or molecular fragments or pellets of stem cells were implanted within the dental pulp [49]. Agarose beads 75–150 μm in diameter were used as carrier.
16.5.1.3 Materials and Methods
Following institutionally approved protocols for animal experimentation research, the rats were anesthetized by intraperitoneal injection of 20 % ketamine (Imalgene; Alcyon) and 5 % xylazine (Rompun; Alcyon) solution. A cavity was drilled on the mesial aspect of the tooth using a tungsten dental bur (ISO 006; Dentsply) with a low-speed handpiece. Pulp perforation was accomplished by pressure with the tip of a steel probe. This method avoids rolling the pulp around the dental bur. The surgical approach was followed or not by agarose bead implantation. The beads were loaded or not by extracellular matrix molecules or solely acting as ECM or cell carriers. They were implanted into the pulp exposure of the first maxillary molar of Sprague-Dawley rats, 9 weeks old. About 105 A4 multipotent stem cells were collected in a tube and centrifuged to form a pellet, which was subsequently implanted into the pulp of rat’s molars (Figs. 16.1a–f and 16.2a–f).
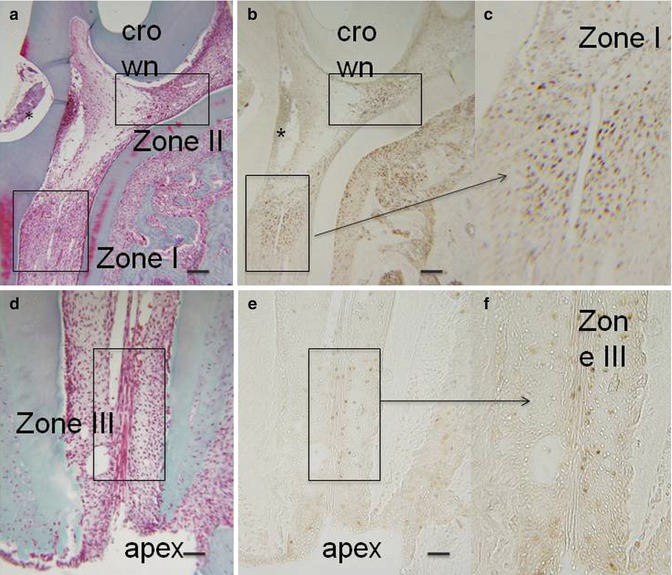
Fig. 16.1
(a, d) Hematoxylin/eosin. After the preparation of a cavity in the mesial aspect of the first mandibular molar, after proliferating cell nuclear antigen (PCNA), three zones were identified. Two zones in the coronal part, one near the cavity (zone I) and another in the isthmus between the mesial and central parts of the pulp (zone II). The zone III was located apically near the root ending. (b, c, e, f): PCNA staining. * is the place where the cavity was drilled
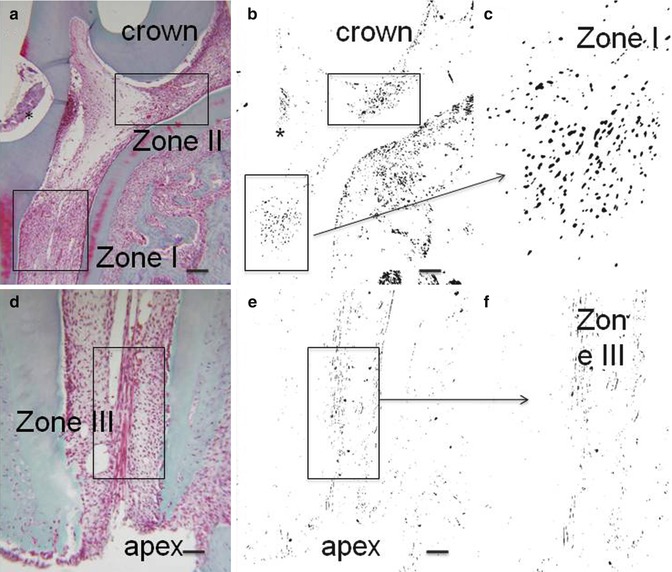
Fig. 16.2
(a, d) Hematoxylin/eosin. (b, c, e, f): negative staining of the same labeled sections. * is the place where the cavity was drilled
In the control group of rats, cavities were not prepared. At the end of tooth morphogenesis, odontoblasts and the subodontoblastic Hoehl’s cells are postmitotic cells. Accordingly, in sound teeth, after immunostaining with the proliferating cell nuclear antigen (PCNA) or with the silver-binding nucleolar organizer region (AgNOR), odontoblasts remain unlabeled. In physiological conditions, no proliferating cells are found in the human coronal pulp and only a low proliferative activity is confined to the radicular pulp [50]. Upon advanced dental caries, PCNA-stained cells become detectable in the coronal pulp [51]. After a dental lesion, the staining seen after 5-bromo-2′-deoxyuridine (BrdU) labeling disappears from the pulp-dentin border, and a wide range of proliferative cells is seen within the dental pulp. The pulp of a few animals was exposed but not implanted. The three other groups of rats are indicated in Table 16.2.
Table 16.2
The three groups of rats: sham group and two experimental groups
The sham group: A surgical pulp exposure was performed in rat molars. They were implanted with agarose beads, loaded or not with ECM molecules or A4 cells. One to 3 days after bead implantation, the pulp tissue appeared normal, without inflammatory process. In the absence of A4 cells, inflammatory cells were recruited near the implantation site. One month after the surgery, the mesial pulp chamber was fibrotic, but was never mineralized
|
Two experimental groups were prepared
|
1. The experimental A4 implantation group: After 1 month, the mesial part of the pulp chamber was massively filled by mineralized osteodentin, separated from the previously formed dentin by a calciotraumatic line
|
2. Implantation of agarose beads loaded with or without A4 cells: In order to improve the implantation protocol, i.e., precisely visualize the implantation site and control the number of cells implanted within the pulp, we used agarose beads as a carrier
|
The pulps were either exposed but not implanted (control groups) or implanted with unloaded agarose beads (sham groups) or with agarose beads loaded with bioactive ECM molecules (A+4, A-4, or LRAP amelogenin peptides) or with pellets of A4 cell lines. Agarose beads loaded by one amelogenin peptide A-4, A+4 (generous gifts from Prof. Art Veis, Northwestern University, Chicago) [52], or LRAP fragment (generous gift from Prof. Pamela Denbesten, University of California at San Francisco) were implanted in the exposed dental pulp (experimental group with bioactive molecule implantation).
To protect the pulp from bacterial contamination, after the surgery, the cavities were filled with glass ionomer cement (Fuji IX; GC). One, 3, 8, 15, or 30 days after the preparation of cavities alone, or after pulp implantation of unloaded or bioactive ECM molecule-loaded agarose beads, rats were anesthetized and killed by intracardiac perfusion of the fixative solution (4 % paraformaldehyde solution overnight at +4 °C). For each subgroups, three rats were killed for each period of time. Block sections including the three hemi-maxillary molars were dissected, fixed by immersion, demineralized in a 4.13 % EDTA, and embedded in paraffin (Paraplast Plus; Kendall).
Seven thick sections were collected on glass slides and observed after Masson’s trichrome staining (Fig. 16.3a, b). Other slides were immunostained with the proliferative cell nuclear antigen (PCNA), and all the mitotic cells were labeled. We used also antibodies raised against CD68 used to label monocytes/macrophages, MHCIIB identifying mainly dendritic cells, and α-smooth muscle actin (α-SMA) [53]. As control, we omit the primary antibody and employ only the secondary antibody. Western blots were used to ascertain the specificity of the antibodies. Using antibodies against actin to label microfilaments and alpha-acetyl tubulin revealed microtubules and cilia (Figs. 16.2a–f and 16.4a–l).
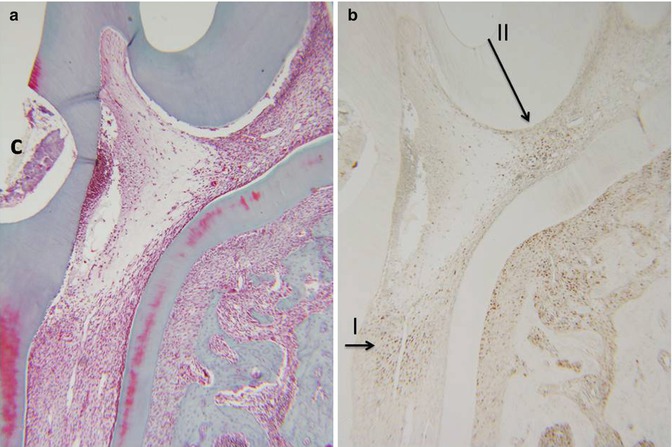
Fig. 16.3
(a) Hematoxylin/eosin staining. (b) PCNA labeling of zones I and II. C cavity
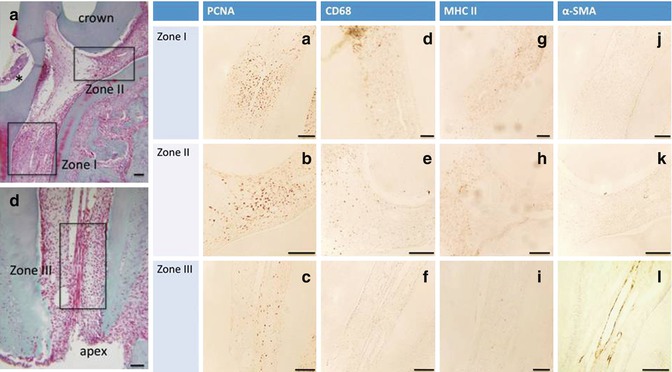
Fig. 16.4
(a–l) First column PCNA (the three zones are labeled), second column CD68, and third column MHC II (a few cells are labeled in zones I and II; in zone III MHC II alone label this zone): inflammatory cells; fourth column: alpha-smooth muscle actin (α-SMA) endothelial vascular cell (only zone III is labeled)
Ultrathin sections of rat’s molars were obtained after rapid freezing followed by freeze-substitution, and replicas were prepared after freeze fracture. Junctional complexes were identified by electron microscopy in the pulp of rat molars. In the extracellular matrix, thin collagen fibrils, glycosaminoglycans, and proteoglycans were visualized.
16.5.1.4 Results
When no cavity was prepared or prior to a pulp exposure, no PCNA positively labeled pulp cell was detectable, both in the crown and in the root. Consequently, cell divisions appeared to be associated with a repair process during the formation of reactionary dentin. The mitotic reaction was enhanced and produced reparative dentin mostly after a pulp exposure.
Forty-eight hours after a pulp exposure of the first maxillary molar, three different PCNA-labeled areas were observed in the dental pulp [45].
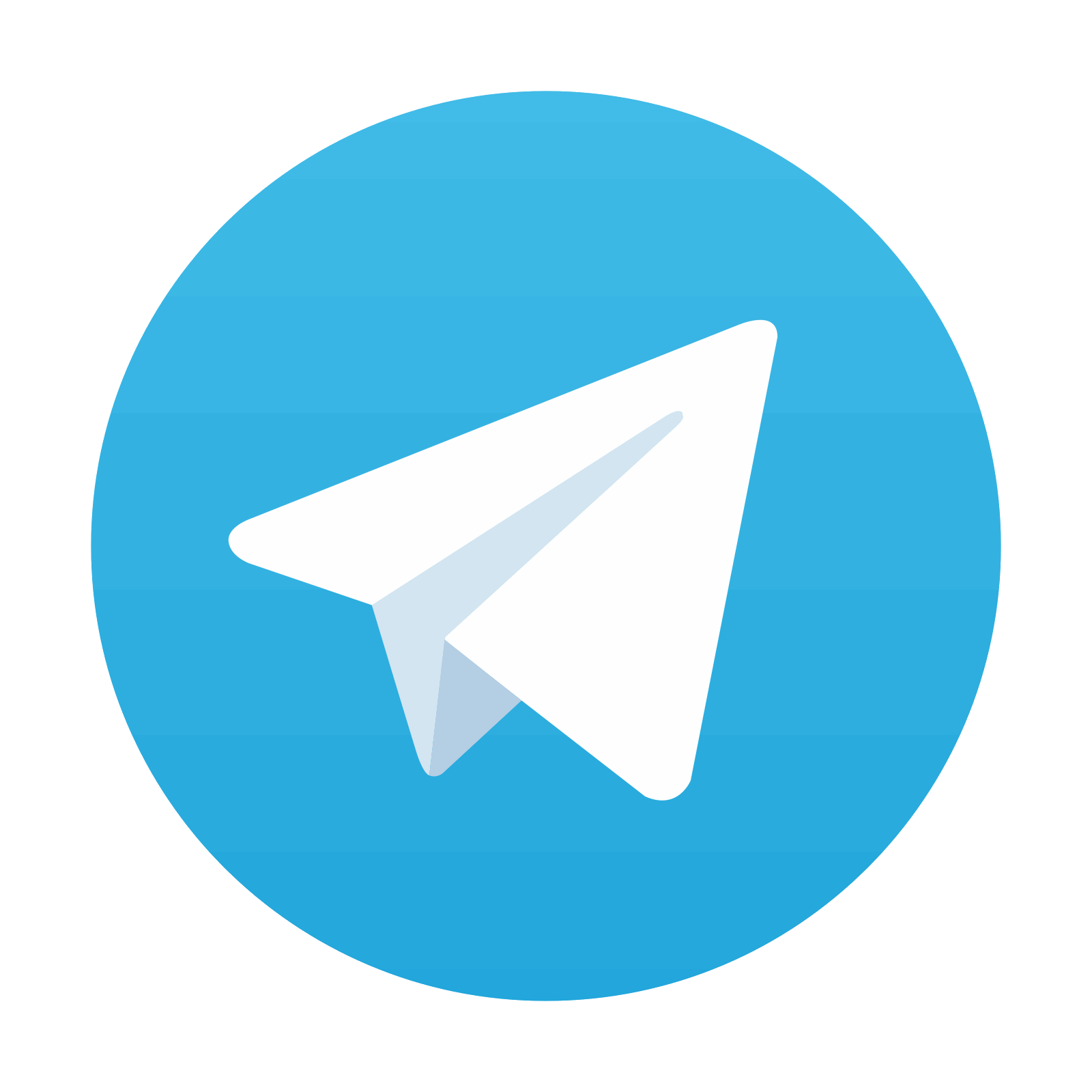
Stay updated, free dental videos. Join our Telegram channel

VIDEdental - Online dental courses
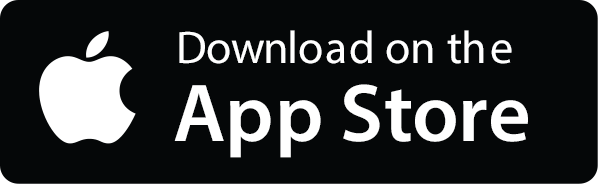
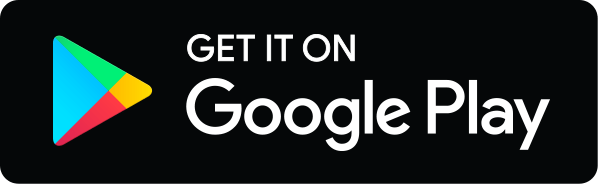