Fig. 5.1
Dental pulp vascularization. The dental pulp is highly vascularized. This vascularization is re-established after pulp healing and dentin regeneration. (a) Inflammation induces dilatation of blood vessels. These can be easily distinguished under a carious injury at low (b) and high magnifications (c). Arrows blood vessels, P pulp, D dentin, O odontoblasts, star tertiary dentin scale bar 100 μm
Recent advancements revealed some interesting aspects demonstrating a local regulation of pulp angiogenesis that may be valuable in future tissue engineering investigations for regenerating a vascularized pulp.
For a better understanding of the pulp vasculature, it is important to know how it is formed during tooth development and in mature teeth. It is also important to understand how it can be re-established after pulp injury and during tissue engineering.
5.2 Establishment of the First Capillary Network (Vasculogenesis)
The main cell-forming unit of the vascular system is the endothelial cell which forms the internal lining of blood vessels. These cells are derived from mesoderm stem cells which give rise to haemangioblast precursor cells which in turn give rise to the haematopoietic stem cells and angioblasts: the progenitors of endothelial cells [2]. Thus, blood cells and endothelial cells share a common origin and remain tightly linked to each other during adult life (Fig. 5.2).
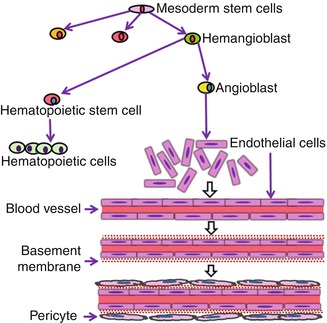
Fig. 5.2
Origin of endothelial cells and vasculogenesis. Endothelial cells derive from mesoderm stem cells and share a common origin with haematopoietic cells. During the embryonic development, angioblasts differentiate into endothelial cells which establish well-organized networks of blood vessels through the vasculogenesis process
During the tooth development and in the absence of blood vessels, oxygen and nutrients may reach the embryonic tissues by simple diffusion [3]. The first evidence of mesodermally derived cell migration was observed within the dental papilla at the early bell stage [4]. This migration was demonstrated on tooth slice cultures of E14.5 mouse mandibles comprising the first molar tooth germ at the cap stage. CD31-positive endothelial cells were present in the dental follicle surrounding the forming dental papilla. After 4 days in culture, they entered the dental papilla, and the first capillary network of blood vessels rises de novo at the late bell stage [5]. At this stage, the metabolic activity and oxygen requirements of both epithelial and mesenchymal cells are high. Endothelial cells connect to each other and organize into hollow and interconnecting structures forming the blood vessels (Fig. 5.2) of the tooth by a process called vasculogenesis [6]. This is followed by the recruitment of smooth muscle-like pericytes to cover capillaries [7, 8]. During angiogenesis which occurs at later developmental stages, this primary network is remodelled, adjusted and specialized into arterial and vein capillary beds [9]. Expression analysis of vascular endothelial growth factor (VEGF) and its receptors (VEGFR) demonstrated that VEGF signalling pathway plays a central role in the migration of angioblasts during vasculogenesis [10]. The importance of VEGF signalling was further supported by the fact that heterozygote deletion of VEGF-A, VEGFR1 and VEGFR2 in knockout mice led either to a strong alteration or to a nearly complete lack of blood vessels in mouse embryos [2, 11, 12].
Although vasculogenesis is believed to be the main process involved in blood vessel formation until the late bell stage of tooth development, investigations using CD34 as marker of endothelial cells revealed that vasculogenesis can still be functional at later stages in mature teeth. The presence of CD34+ endothelial cells in permanent teeth suggests that vasculogenesis persists into adult life, where it contributes to continuous adjustment of vessel and network structures in response to functional needs and dental tissue homeostasis [13].
5.3 Angiogenesis
At later developmental stages and in the mature pulp, endothelial cells form blood vessels by another process called angiogenesis. This process implies the formation of new blood vessels by “sprouting” from pre-existing blood vessels. This occurs via the extension or remodelling from existing capillaries (Fig. 5.3a–c). This process is encountered during chronic inflammation, menstrual cycle and tumour growth. It is a critical part of the wound healing process in all tissues, and the local pulpal angiogenesis is a prerequisite for successful repair in the tooth [14]. It is also a key process in tissue engineering procedures. If blood supply is not established rapidly into the transplanted cells/tissues, a necrosis occurs due to a lack of oxygen and nutrient supply [15].
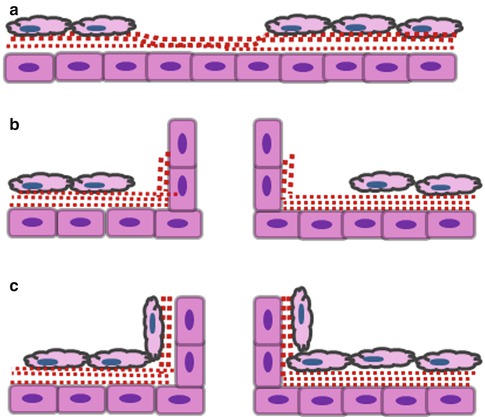
Fig. 5.3
Angiogenesis by sprouting from pre-existing vessels. This is a multistep process which implies the following: (a) pre-existing vessel destabilization (angiopoietin 2, VEGF), extracellular matrix degradation (MMP, chymases, heparanases) and release of matrix-sequestered molecules (VEGF, FGF-2, IGF1); (b) proliferation (VEGF, FGF-2, EGF, HGF, PLGF, TGF-β, TNF-α) and anastomosis (VEGF, angiopoietin 1, VE cadherin, ephrin B2/B4); and (c) pericyte recruitment (angiopoietin 1, PDGF, TGF-β, VE cadherin)
Angiogenesis is a complex process. It implies extracellular matrix secretion and remodelling, secretion of proteolytic enzymes to degrade the vessel wall extracellular matrix, endothelial cell migration and proliferation, capillary differentiation and anastomosis [16]. This process is regulated by many inductive and inhibitory signals [17, 18]. Among all the pro-angiogenic factors, VEGF is considered the most essential for the differentiation of the vascular system. It induces endothelial cell proliferation, migration and survival [12]. Basic fibroblast growth factor (FGF-2) stimulates angiogenesis in vivo and plays a significant role in vascularization of damaged or traumatized tissue [19]. Platelet-derived growth factor (PDGF) is a smooth muscle cell mitogen [20] and plays an essential role in the formation of new vessels and in maintaining their stability [21]. Angiopoietins (Ang1, Ang2) and their receptor (Tie-2) play a role in destabilizing the blood vessel and establishment of contacts between endothelial cells. Transforming growth factor β1 (TGF-β1) is involved in endothelial cell proliferation [22]. On the other hand, anti-angiogenic signals regulate the vasculature and inhibit overgrowth of blood vessels. Among these, endostatin inhibits endothelial proliferation and angiogenesis [23]. Angiostatin is considered as a potent inhibitor of angiogenesis by selectively inhibiting endothelial cell proliferation [24]. Thus, angiogenesis appears as a balance between pro- and anti-angiogenic signals, and a “switch” occurs under pathological conditions.
The endothelial cells in the blood vessels are closely related to the vascular basement membrane extracellular matrix. This membrane inhibits endothelial cell proliferation, but upon degradation by proteolytic enzymes, it allows endothelial cell proliferation and angiogenesis to proceed. The basement membrane also plays critical roles in most of the processes of blood vessel formation and stabilization. This role is best demonstrated by extracellular matrix molecule null mutations. Indeed, in α-1(I) collagen knockouts, mutants die at E12–E14 due to blood vessel rupture. This suggests that fibrillar collagen type I may play an important role in the blood vessel stability [25]. In fibronectin knockout mice, a range of phenotypes including deformed heart and embryonic vessels was observed. This may be due to mesenchymal defects mainly in cell proliferation, adhesion and migration [26, 27]. Perlecan is a proteoglycan synthesized by both vascular endothelial and smooth muscle cells and deposited in the extracellular matrix. It has been shown that half of perlecan knockout mice die at E10 or E12 due to pericardial haemorrhage and to death from respiratory failure at birth. This may be due to rupture of basement membranes [28]. These data clearly demonstrate that the composition of the basement membrane of blood vessels and its structure is a key element in blood vessel stabilization and in maintaining its physiological function.
5.3.1 Intussusceptive or Non-sprouting Angiogenesis
Intussusceptive angiogenesis does not require cell proliferation but rather endothelial cell reorganization to form new blood vessels from pre-existing ones. During this reorganization, an individual blood vessel splits into two separate compartments leading to the formation of two individual blood vessels [29]. There is no evidence of the existence of this process within the mature dental pulp where angiogenesis occurs mainly by sprouting angiogenesis.
5.4 The Dental Pulp Is a Highly Vascularized Tissue
The development and establishment of pulp vascularization by these processes in embryonic and mature teeth lead to the formation of a densely vascularized pulp tissue. In mature teeth, the maxillary artery brings blood supply to the pulp through the dental artery which feeds each pulp via arterioles. These arterioles enter the apical foramina and ascend the central region of the coronal pulps and branches off to form a rich capillary network at the periphery of the pulp [30, 31]. During primary dentinogenesis, this vasculature develops in the odontogenic zone of the dentine-pulp complex, showing increased vessel fenestration for odontoblast nutrition. With the completion of dentine formation, a decrease in fenestration and a withdrawal of vessels from the odontoblast layer can be seen so that in the mature tooth of limited growth, the capillary network is confined to the subodontoblastic region [32]. A parallel network drains blood into the central pulp venules and leaves out the pulp via the apical foramina [30].
Thus, the dental pulp becomes a highly vascularized tissue both due to vasculogenesis and angiogenesis. It has been shown that the dental pulp has an average capillary density higher than in most other tissues [33]. The pulp blood flow is also high (around 50 ml/min/100 g of pulp tissue) [34] as compared to that of other tissues [35].
5.5 The Pulp Vascularization Has Unique Properties
1.
The dental pulp is surrounded by inextensible calcified dentin walls. Because of this low-compliance encasement, intrapulpal tissue pressure is of prime importance in pulpal physiology. During acute inflammation, capillary dilatation may lead to a significant swelling of the dental pulp which, in turn, increases the local blood pressure that stimulates pulp nerves leading to pain sensation. The measurements of the local pressure indicate that its increase due to inflammation is a localized event which is not transmitted to the rest of the pulp. This was demonstrated by measuring interstitial fluid pressure in cat dental pulp 7 days after inducing pulpitis in vivo. Under pulpitis, the pressure was significantly high (16 mmHg) as compared with controls (5.5 mmHg). However, the pressure measurements at a site 1–2 mm distant to the induced inflammation site averaged 7.0 mm indicating that the increase in pulp blood pressure is a very localized event [36].
2.
The combination of both the pulp resilient ground substance properties and the localized pulpal pressure does not transmit the deleterious effects of increased pressure to the pulp and limits its transmission throughout the pulp [37]. Thus, the thin-walled veins and venules collapse only in the area of the affected pulp tissue, leading to a local vascular stasis and cell death.
3.
The dental pulp has arteriovenous anastomoses providing a direct communication between arterioles and venules. Although the function of these anastomoses is not well elucidated [31], these shunt vessels may open up to counterbalance any significant rise in pulp blood pressure. When the intrapulpal pressure rises during inflammation, these shunt vessels that are abundant at the apical half of the pulp may communicate to reduce the intrapulpal pressure and maintain normal blood flow [38]. This suggests a local vascular regulation in the pulp [39]. Indeed, recent works allow a better understanding of this regulation which seems to occur at different levels:
5.6 Neural Control of Blood Flow
The sensory nerves predominate in the dental pulp which is innervated by large numbers of unmyelinated and small myelinated axons of autonomic and sensory origin [40]. However, there is no evidence for pulpal blood flow control by sympathetic or parasympathetic nerve activity to meet specific requirements of the pulp tissue, and neural control seems to be operative on a local scale. It has been shown that large terminal arterioles in the rat incisors receive a dense nerve supply, suggesting an important role of neuronal regulation on the vessels [41]. Perivascular sympathetic nerve fibres liberate noradrenaline and neuropeptide Y leading to a reduction of pulp blood flow [42], whereas intradental sensory nerves liberate neuropeptides which increase pulp blood flow [43]. The perivascular nerve endings are either adrenergic containing noradrenaline or somatosensory nerve fibres containing substance P or calcitonin gene-related peptides [41]. These nerve fibres participate in the pulp blood flow regulation by affecting vascular smooth muscle tone and vessel diameter. Thus pulp blood flow is under the influence of local nerve impulses. This is why pulp blood flow is considered to be predominantly under neural local control [44].
5.7 Neo-angiogenesis Is a Requirement for Regeneration and Healing
While an increased vasodilatation brings more blood supply to the inflamed tissue, sprouting of capillaries leads to an increase of their density which increases blood perfusion of hypoxic tissue to restore local oxygen and nutrition supply. Thus, neo-angiogenesis appears as a basic requirement under hypoxia.
5.8 Hypoxia Triggers Pulp Angiogenesis
After the initial vasodilatation of acute inflammatory reaction which increases blood supply to the pulp, an adaptive formation of capillaries by neo-angiogenesis is initiated as a reaction to hypoxia in ischemic tissues during the regeneration process [45, 46]. The regulation of angiogenesis by hypoxia ensures an adequate oxygen and nutrient supply required to meet the needs of the hypoxic tissue. Hypoxia can be frequently encountered in the dental pulp under different clinical situations such as carious or traumatic pulp injuries, orthodontic movements or replanted avulsed teeth. Maintenance of oxygen homeostasis is essential to all living tissues. It is regulated through the activity of hypoxia-inducible transcription factor-1 (Hif-1) [47]. This transcription factor is a hetero-dimeric protein of a constitutive subunit (Hif-1β) and an oxygen-sensitive subunit (Hif-1α). Under hypoxic conditions, Hif-1α expression increases rapidly in the cells and binds to specific enhancer elements in the promoter region of pro-angiogenic genes [48, 49]. Hif-1α plays a key role in angiogenesis by activating the transcription of genes encoding pro-angiogenic growth factors including VEGF, FGF-2, angiopoietin 1 and 2 (Ang1, Ang2), placental growth factor (PGF), PDGF and angiogenic receptors [50–53]. These factors induce different steps of the neo-angiogenesis process (Fig. 5.4). The strong mitogen VEGF-A, for example, has induced not only proliferation of endothelial cells but also permeabilization in the initiation of the neo-angiogenesis process.
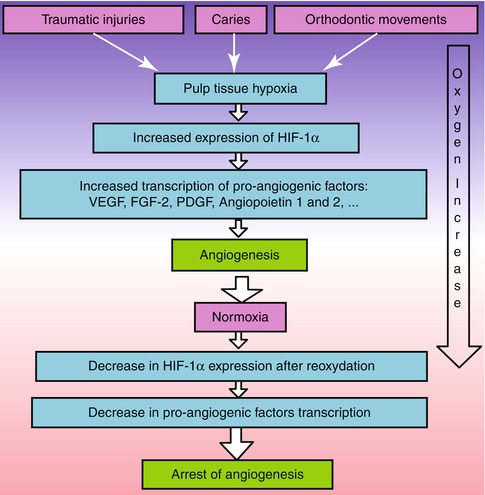
Fig. 5.4
Pulp hypoxia induces neo-angiogenesis. Hypoxia up-regulates hypoxia-inducible transcription factor-1α (Hif-1α) which induces pro-angiogenic factor transcription and neo-angiogenesis. This increases oxygen supply to the hypoxic tissue. After reoxidation, Hif-1α expression decreases and neo-angiogenesis is arrested. Arrow indicates the increase in oxygen supply
Given the fact that TGF-β, FGF-2 and PDGF growth factors have been reported to up-regulate VEGF expression [54], the capillary network formation may be triggered by a combined action of both hypoxia and locally secreted factors [49].
In the dental pulp, induction of Hif-1α was demonstrated after plating pulp cells under anaerobic conditions for 6 h [55]. Immunocytochemistry revealed that Hif-1α was localized primarily in the cell nuclei under hypoxic conditions [53]. This induction was also reported, in the dental pulp, in an orthodontic tooth movement model.
Hif-1α induction was also demonstrated both in dental pulp stem cells and pulp fibroblasts cultured in a hypoxia chamber at 37 °C containing 1 % O2. In both cell types, Hif-1α expression peaked at 4 h of hypoxia and then decreased gradually through 24 h under normoxia. This indicates that this protein subunit is degraded after re-oxygenation. This degradation was also associated with a decrease in pro-angiogenic factor transcription, and this led to an arrest of neo-angiogenesis (Fig. 5.4). Interestingly, the fact that Hif-1α expression increases in dental pulp stem cells implies that these cells may be activated under hypoxia not only to regenerate dentin but also to regenerate the pulp vasculature.
5.9 Pulp Fibroblasts Induce Endothelial Network Formation
In an attempt to understand the effect of both hypoxia and locally secreted pro-angiogenic factors within the pulp, a coculture system was used. Both endothelial and pulp fibroblasts were transduced with fluorescent proteins and plated as a mix in an 80–20 % ratio on the surface of a Matrigel extracellular matrix to simulate the pulp extracellular matrix. The coculture of endothelial cells with pulp fibroblasts induced time-independent morphological changes in the endothelial cells. At 3–5 h, both cell types had a spherical or a fibroblastic morphology. The endothelial cells spread and started to organize after 24 h, while fibroblasts had the typical fibroblastic appearance. At 48 h, most endothelial cells formed tubular and closed structures. After 6 days, most endothelial cells became elongated, forming thin cords of interconnecting cells, and exhibited elongations and branching to form a 2D network of capillary-like structures corresponding to neo-angiogenesis in vivo [56, 57]. Blood vessels are clearly visible after 14 days (Fig. 5.5a–d). This indicates that, in addition to hypoxia, pulp fibroblasts are essential in maintaining pulp vasculature homeostasis.
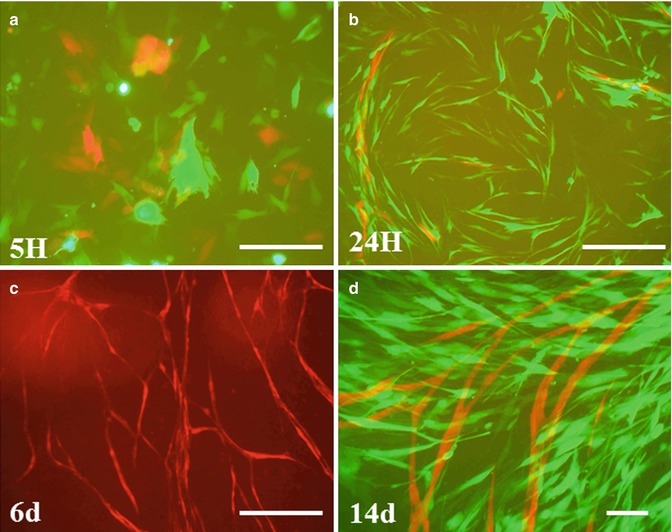
Fig. 5.5
Pulp fibroblasts induce neo-angiogenesis. Pulp fibroblasts and endothelial cells were transduced with fluorescent proteins and cocultured on Matrigel. Pulp fibroblasts induced formation of vascular network by endothelial cells. The cells are separated from each other after 5 h of culture (a). After 24 h, endothelial cells elongate and form anastomosis (b). After 6 days, a 2D network is formed (c). Blood vessels are seen after 14 days (d). Scale bar = 200 μm
In order to understand the consequences of hypoxia on the secretion of angiogenic factors, pulp fibroblasts were cultured in vitro, and injuries were performed mechanically to disrupt the fibroblast monolayer, simulating the hypoxia under traumatic pulp injury. The culture medium obtained after 5 h of contact with intact or injured pulp fibroblasts was used as a culture medium to feed a separate culture of endothelial cells on Matrigel. These media induced marked changes in endothelial cell morphology, with structural rearrangements leading to the organization of endothelial cells into capillary-like networks in 24 h. The dimensions of the newly formed tubes were larger in the medium obtained from injured cells when compared to the dimensions obtained with the medium from intact cells. This organization is due to the secretion of higher level of angiogenic factors from injured pulp fibroblasts as demonstrated by quantitative ELISA measurements of FGF-2, VEGF and PDGF [50]. This increased secretion of pro-angiogenic factors and higher stimulation of endothelial organization by injured pulp fibroblasts highlights the direct involvement of pulp fibroblasts in angiogenesis under hypoxia.
5.10 Pulp Angiogenesis Is Tightly Controlled by the Microenvironment
Several lines of evidence suggest that pulp vascularization is under a strong local control [57, 58] via pro- and anti-angiogenic factors and neuropeptides that can be liberated from the microenvironment [59]. The sources of these signals include the dentin and the different cell types within the dental pulp including pulp fibroblasts, endothelial cells and pulp nerves (Fig. 5.6).
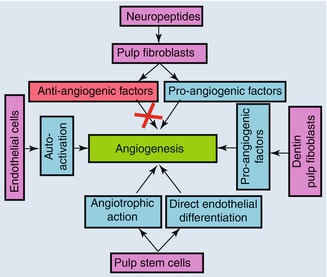
Fig. 5.6
Regulation of pulp angiogenesis by the microenvironment
5.10.1 Dentin and Pulp Fibroblasts
The dentin acts as a reservoir of signalling molecules. Pro-angiogenic factors such as VEGF and FGF-2 were identified in the dentin and may play a role in angiogenesis when released under carious injuries [60].
Pulp fibroblasts express several pro-angiogenic growth factors such as angiogenin, angiopoietin 2, epidermal growth factor (EGF), heparin-binding epidermal growth factor, hepatocyte growth factor, leptin and placental growth factor [59]. They also secrete FGF-2, VEGF and PDGF under normal culture conditions, and this secretion in the culture medium increases when injuries were performed to the cell layer. When the culture medium obtained from injured pulp fibroblasts was pre-incubated with neutralizing antibodies to both VEGF and FGF-2 and used for the culture of endothelial cells on Matrigel extracellular matrix, a significant decrease of capillary formation capacity was obtained [56]. This result demonstrated that both factors were involved in pulp neo-angiogenesis. These data highlight the fact that the locally secreted VEGF and FGF-2 under hypoxia play a major role in neovascularization [61]. Several works strongly suggest the involvement of these pro-angiogenic factors under pathological pulp conditions. VEGF expression has been reported in inflamed pulp tissues [62], and its secretion has recently been reported in MDPC-23 cells in response to adhesive resins [63] and resinous monomers [50] or after lipoteichoic acid application suggesting the implication of this growth factor in angiogenesis under pathological conditions [64]. The dental pulp fibroblasts express and secrete the pro-angiogenic factor TGF-ß1. The secretion of this factor is modulated by the restorative materials. Its secretion increases when pulp cells are placed in contact with bioactive tricalcium-based restorative materials such as the mineral trioxide aggregate or Biodentine. On the opposite, this secretion decreases when the cells were placed in contact with a resin-based adhesive [65].
5.10.2 Endothelial Cells
Endothelial cells also produce pro-angiogenic growth factors for the regulation of their own activity. It has been well established that injured endothelial cells release signalling molecules that initiate the inflammatory reaction and the healing process [66]. Cultured endothelial cells, which are known to express VEGF receptors, secrete VEGF and FGF-2. When physical injuries were performed on these cells, endothelial cells increased VEGF and FGF-2 secretion. The secretion level of VEGF significantly peaked 5 h after the injury and returned to baseline values 24 h later. Similarly, a significant increase in FGF-2 secretion was observed after 24 h and returned to baseline values after 48 h [58].
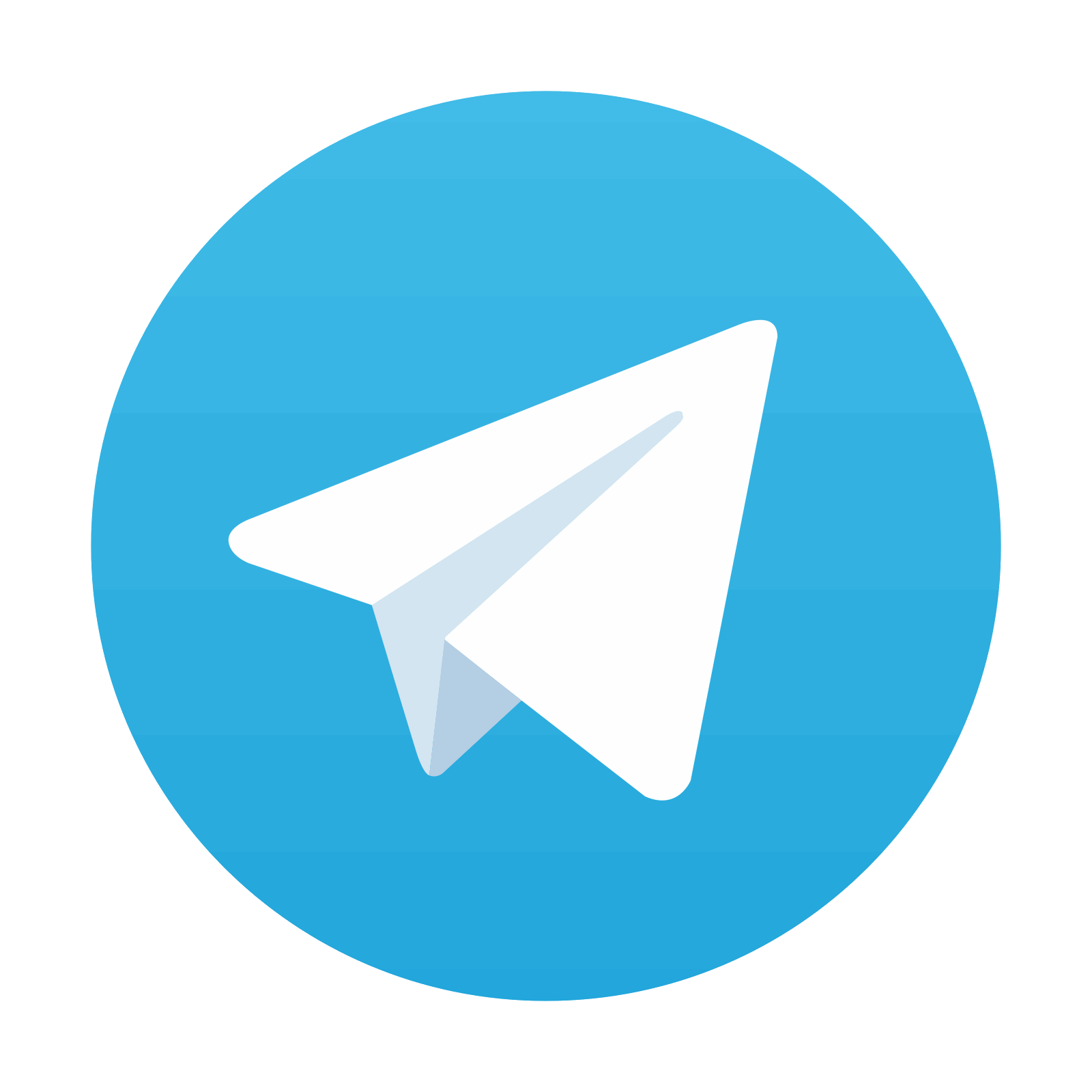
Stay updated, free dental videos. Join our Telegram channel

VIDEdental - Online dental courses
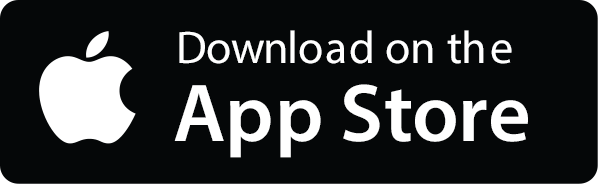
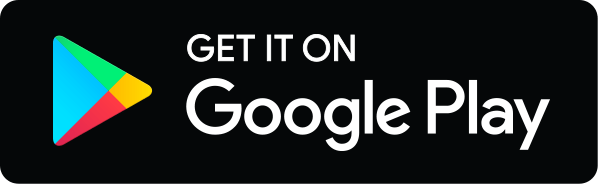