Introduction
Root resorption resulting from orthodontic treatment is an unpredictable adverse effect. Literature examining the potential protective influence of tooth cementum minerals against orthodontically induced inflammatory root resorption has been sparse. Fluorine could have a role in minimizing the extent and severity of resorptive lesions. The purpose of this study was to examine the elemental content of tooth cementum in orthodontically induced inflammatory root resorption lesions and the effect of systemic fluoride.
Methods
Twenty 7-week-old Wistar rats were divided into 2 groups of 10 and exposed to systemic fluoride (100 ppm) or nonfluoridated drinking water for 2 weeks. Orthodontic tooth movement was implemented with a nickel-titanitum closing coil with a force of 100 g. The molars were then extracted, dissected, and prepared for cross-sectioning through the largest mesial midroot crater. The samples were mounted and scanned by using the Commonwealth Scientific and Industrial Research Organisation and the Australian Research Council’s National Key for Geochemical Evolution and Metallogeny of Continents Nuclear Microprobe (Melbourne, Victoria, Australia). Analysis of variance (ANOVA) was used for statistical comparison of the elements and to determine the effect of fluoride, and unaffected tooth structure compared with root resorption craters. The Student t test was used to compare root crater lengths and depths of the fluoride vs no-fluoride groups.
Results
Root resorption lesions of the group exposed to fluoride were significantly reduced in length and depth ( P <0.01). The mineral content of the root resorption craters of the fluoride group had higher concentrations of fluorine and zinc ( P <0.01). There was less calcium in the craters of the no-fluoride group compared with the fluoride group ( P <0.05).
Conclusions
Cementum quality (influenced by systemic fluoride exposure) might impact the extent of orthodontically induced resorptive defects.
Root resorption resulting from orthodontic treatment is an unpredictable adverse effect involving transient inflammatory surface resorption. This process has been termed orthodontically induced inflammatory root resorption (OIIRR). The mechanism of OIIRR has not been fully elucidated. After orthodontic force application, tooth movement is associated with local compression of the periodontal ligament and the development of sterile necrosis. This morphometric phase is followed by biochemical changes. The sequence of events involves fiber coalescence, hyalinization, and degradation of connective tissue matrices (extracellular and intracellular pathways) followed by dissolution of Sharpey’s fibers and, finally, odontoclastic activity. The resorptive process initially occurs at the circumference of the necrotic hyalinized area. This is followed by resorption of the root surface in the central part of the necrotic zone. The later stages also demonstrate areas of peripheral repair.
Associated risk factors for increased incidence of root resorption include individual susceptibility, genetics, hormonal imbalance, medical history, nutritional balance, age, dental history, tooth type, duration of treatment, amount of tooth movement, appliance type, type of tooth movement, and magnitude of orthodontic force.
There is currently no consensus on how to, or whether it is possible to, prevent root resorption. However, strategies have been suggested on ways to minimize root resorption, such as limiting treatment duration, using light intermittent forces, assessment of medical history and familial tendency, control of habits, and, if required, cessation of treatment.
The literature is sparse in regard to examining fluoride’s potential protective influence and the effect of the mineral content of cementum against OIIRR. Foo et al used rats to induce orthodontic root resorption and exposed part of the sample to systemic fluoride, with an estimated intake of 1 mg per day. It was demonstrated that the average crater volumes of teeth exposed to fluoride were about half the size. However, because of large variations in results, these differences were not significant at the level of P <0.05.
Our study appears to be unique in examining the elemental content of OIIRR craters. Such information might increase our understanding of this process and the possible protective effects that elements, such as fluorine, impart to cementum. Although fluoride does not increase the physical hardness of cementum per se, there are a number of possible mechanisms whereby fluoride can impart a protective effect against orthodontically induced root resorption. These include direct effect on osteoclasts, alteration of shapes of resorption pits, and mineralization of cementum. Thus, fluoride might be extrapolated as a potential preventive agent against the severity of OIIRR.
There are various methods to study the elemental composition of teeth. The nuclear microprobe uses proton induced x-ray emissions (PIXE) and proton induced gamma-ray emissions (PIGE), allowing mapping of multiple elements at micrometer resolutions. Compared with other available techniques, the Commonwealth Scientific and Industrial Research Organisation and the Australian Research Council’s National Key for Geochemical Evolution and Metallogeny of Continents Nuclear Microprobe (CSIRO-GEMOC NMP) (Melbourne, Victoria, Australia) provides superior spatial resolution (1-2 μm) and quantitative data on elemental concentrations. As yet, this machine has not been used in the study of root resorption lesions in the cementum.
The aims of this study were (1) to develop a methodology to use the CSIRO-GEMOC NMP to analyze the elemental composition of root resorption craters and (2) to analyze the elemental composition of root resorption craters on rat molars after their intake of systemic fluoride.
Material and methods
In this study, we used 20 seven-week-old female Wistar rats (Westmead Hospital Animal Ethics Committee, project 134.12-03). The rats were allowed a week to acclimatize to their new laboratory environment. They were then randomly divided into 2 groups of 10 animals. Group 1 had orthodontic tooth movement and received deionized water that was not fluoridated. Group 2 had orthodontic tooth movement and received fluoridated deionized water (100 ppm). Sodium fluoride was dissolved in Milli-Q (deionized water, Millipore, Bedford, Mass) to meet this concentration level. Water was given ad libitum. Every 2 to 3 days, the water was monitored and replenished as necessary.
Orthodontic tooth movement was implemented with nickel-titanium 100-g closing coils (NiTi 10-000-06, GAC International, Bohemia, NY). The coil was ligated to the rat’s mandibular left first molar by means of a silk suture. The coil was activated via ligation to the mandibular incisors with steel ligatures. Attachment of the nickel-titanium coils was performed under anesthesia. The rats were administered a gaseous anesthetic agent (halothane), followed by the anesthetic agents xylazine (10 mg/kg) and ketamine (90 mg/kg).
The experimental period lasted 2 weeks. Then the rats were killed with carbon dioxide. Their mandibles were dissected and sectioned mesially and distally to the mandibular left first molar. The samples were stored in Milli-Q. This has been shown to have an insignificant effect on leaching of trace elements from cementum samples.
The teeth were progressively dissected from the mandible, with the aim of minimizing fracture of the fine rat molar roots and contact below the cementoenamel junction. This was achieved by severing any remaining marginal gingivae and then removing approximately a third of the alveolar bone surrounding the tooth followed by luxation with a blunt-ended periosteal elevator. This was carried out under a light microscope (WILD M3B, Heerbrugg, Switzerland). After dissection, the teeth were cleaned with sterile gauze soaked in Milli-Q, and gross periodontal ligament fibers were manually removed with tweezers. The teeth were then stored in Milli-Q at ambient room temperature (23 ± 10C°).
All root resorption craters were located on each tooth with a microscope (SZCTV, Olympus, Tokyo, Japan). The largest midroot crater on the mesial root of each tooth was identified.
A “wax marker system” was developed by an author (E.L.) to indicate the point at which the tooth required sectioning ( Fig 1 ). This achieved the goals of marking a microscopic point that could be seen macroscopically for hand sectioning and allowed minimal interference with the tooth minerals. The initial microscope photographs allowed relocation of the midpoint of the root resorption crater of interest.
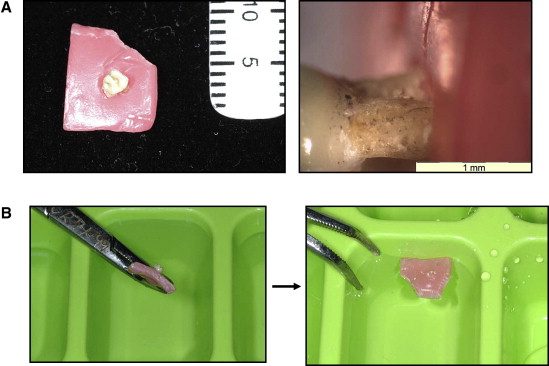
This technique involved softening the center point of an approximately 1 × 1 cm 2 sheet of dental wax (Moyco Union Broach, York, PA) with hot water delivered via a pipette. Under the microscopic view, the wax was pushed over the tooth root to the point of sectioning ( Fig 1 , A ).
The tooth was then floated in Milli-Q with its crown down in an ice cube tray ( Fig 1 , B ). The trays were then frozen for 24 hours. The wax was removed, leaving the exposed root portion to be cut and the exact point or region of interest embedded in the Milli-Q ice block. The specimens were then sectioned transversely, in layers from the apex, through to the identified midpoint of the root resorption crater. This was achieved by using fine and superfine Sof-Lex Discs (3M ESPE Monrovia, Calif) under Milli-Q irrigation to a surface polish of <0.2 μm.
The specimens were then mounted on glass slides with double-sided carbon adhesive tape. The slide and the specimens were carbon coated (evaporated) in a vacuum at 10 −4 mm of mercury with an approximately 20-nm layer. The glass slide was then encased in a metal stub designed specifically for the nuclear microprobe (NMP).
The spatial distribution of multiple elements based on x-ray and gamma ray emissions was measured in the resorption craters and the surrounding nonresorbed cementum of the tooth roots. In this study, we used the NMP developed by the Commonwealth Scientific and Industrial Research Organisation and the Australian Research Council’s National Key for Geochemical Evolution and Metallogeny of Continents.
The analysis was performed with 3.0 MeV protons, and the beam was focused at 1 × 1.8 μm 2 . Scanning was electrostatically driven in the y-axis between 800 and 1500 μm, with the stage stepped in the x-axis (step size, 2.5 μm) to generate a rectangular image. The beam current was maintained at 4 nA. The total accumulated charge for an average sized area was approximately 20 to 25 μC. An aluminum absorber of 100 μm was used between the target and germanium detector (Canberra Ultra-Ge Detector, Canberra, Autstralia). The software used to evaluate spectra was Geo-PIXE II (version 3.8, CSIRO Melbourne Australia). The elements detectable under gamma rays were sodium (Na), fluorine (F), manganese (Mn), aluminum (Al), and silicon (Si); under x-rays, calcium (Ca), iron (Fe), nickel (Ni), copper (Cu), zinc (Zn), bromine (Br), rubidium (Rb), strontium (Sr), tin (Sn), barium (Ba), and lead (Pb) were measurable.
The Geo-PIXE II computer program was used to extract the raw data generated by the scanning process. A manual analysis of regions, to provide quantitative data, was conducted by a function that allowed shapes to be drawn over the downloaded image. The regions analyzed were a “traverse” through the middle of the deepest point of the root resorption crater and adjacent cementum. Readings from dentin below the crater at its marginal cementum and corresponding areas of distant physically unaffected root structure of the same tooth surface were recorded ( Fig 2 , A ). This was repeated for each slice generated from both the x-ray and the gamma-ray scans. In addition to the quantitative data, the information was also used to process spectral (elemental) graphs and picture plots ( Fig 2 , B ). Spectral graphs are traverse concentration profiles of each element, showing the pattern of distribution through the tooth structure. Picture plots are qualitative representations (maps) of relative concentrations, distributed across the scanned region, for each element.
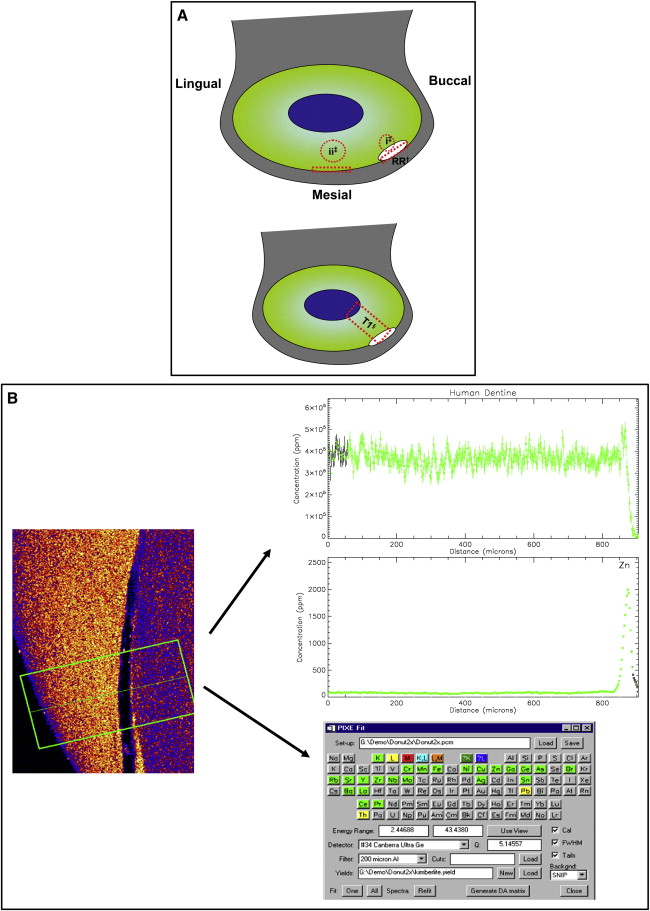
Statistical analysis
SPSS software (version 14, SPSS, Chicago, Ill) was used to compare the element concentrations between the fluoride and no-fluoride groups. Analysis of variance (ANOVA) was conducted to examine the concentrations of elements (F, Mg, Na, Al, Zn, Sr, and Ca), and the factors included were fluoride vs no-fluoride, root resorption crater vs normal tooth structure, and cementum vs dentin. Significant interactions between factors were also included in these models. A multivariate ANOVA was used to examine the effects of these factors on the complete collection of elements as a whole.
The error of the measurement was calculated for F, Mg, Na, Ca, and Zn and was based on triple measurements performed on randomly selected samples from the fluoride and no-fluoride groups. An ANOVA was performed, by using each group of repeated measurements as a unit. The root mean square error was used to give the standard error of measurement. The coefficient of variation was also calculated from the standard error measure as a percentage of the mean.
Because of the large number of dependent tests conducted, a P value of less than 0.01 was taken to indicate statistical significance, and P values between 0.01 and 0.05 were assigned “marginal” status.
Results
The fluoride and no-fluoride groups were analyzed for their elemental content. The error of the measurement was found to be minor, with the highest coefficient of variance 9.4% for Mg. The results for each tooth sample were divided for evaluation of root resorption crater cementum (representing the margin of the lesion), root resorption crater dentin (representing the surface of the crater floor and its immediate subsurface layer), and the cementum and dentin of the adjacent tooth physically unaffected by resorption. Multivariate ANOVA analysis, taking the group of minerals as a whole, demonstrated significant differences between fluoride and no fluoride ( P <0.01), and dentin and cementum ( P <0.01), with a marginally significant interaction ( P = 0.01). However, there were no significant differences between overall elemental concentrations in root resorption and normal tooth structure ( P = 0.27), although there was a marginal interaction between fluoride and no fluoride and root resorption and normal tooth ( P = 0.027). Essentially, the greatest differences were between fluoride and no fluoride, followed by dentin and cementum ( Table I ).
When individual elements were assessed, the fluoride group had significantly more fluorine concentration in the cementum compared with the no-fluoride group ( P <0.01) ( Table I , Fig 3 ). Overall, the fluoride group had a greater concentration of fluorine (mean concentration, 18661.04 ppm) compared with the no-fluoride group (mean concentration, 9791.25 ppm) ( Table II ). However, both groups had similar distribution patterns of fluorine in the dentin ( Fig 3 ).
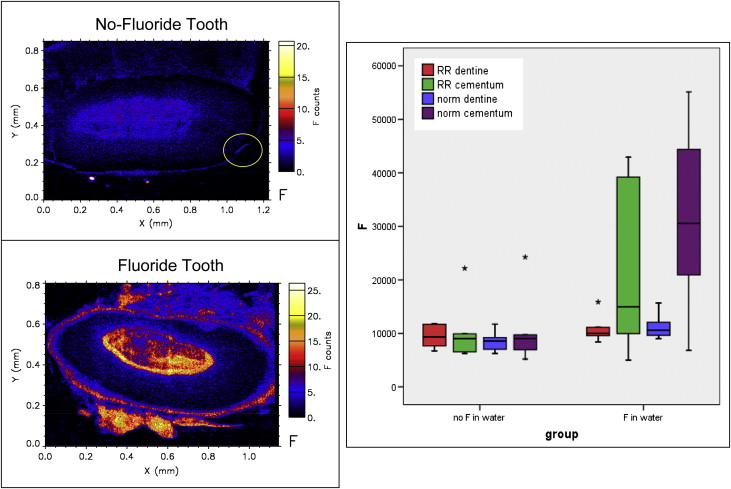
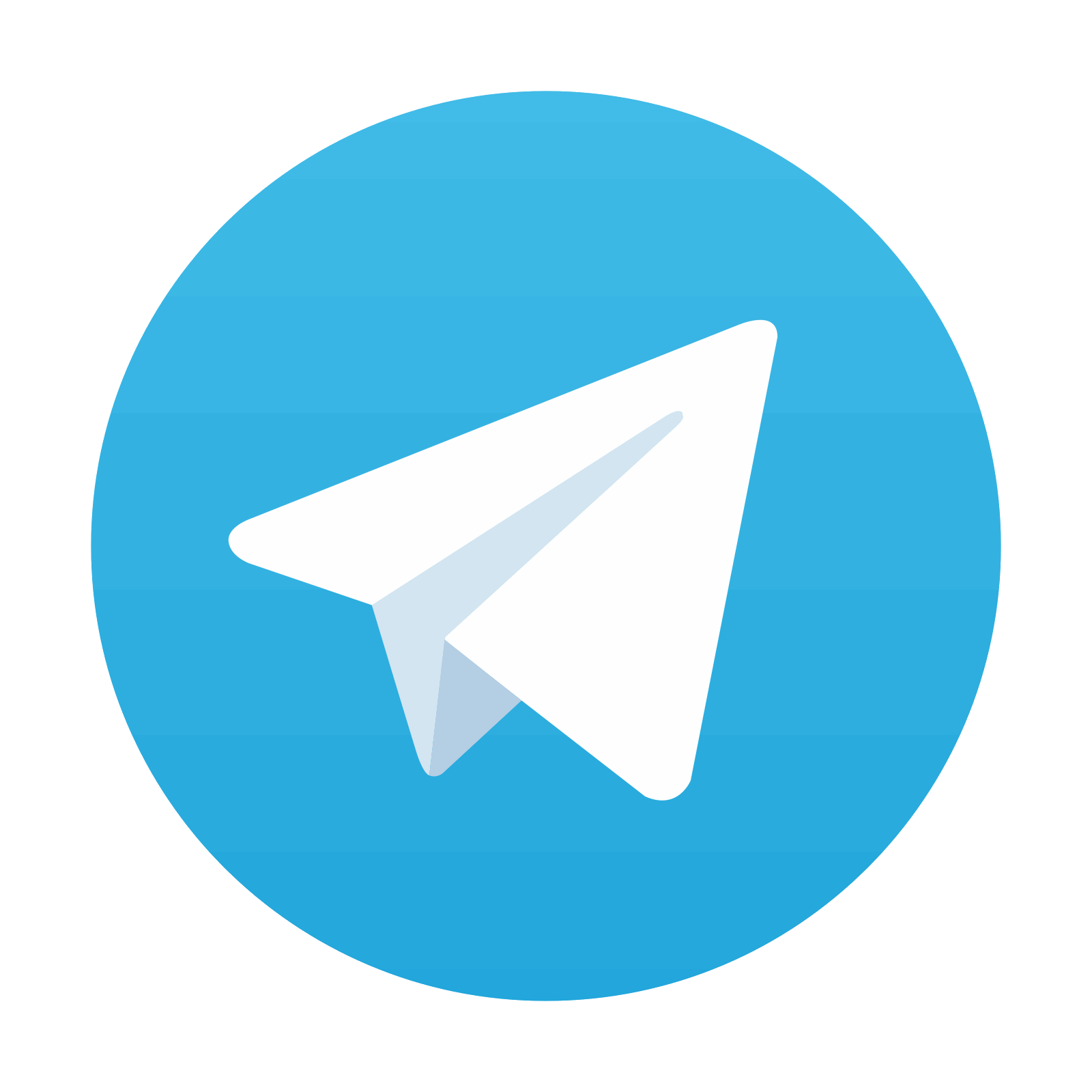
Stay updated, free dental videos. Join our Telegram channel

VIDEdental - Online dental courses
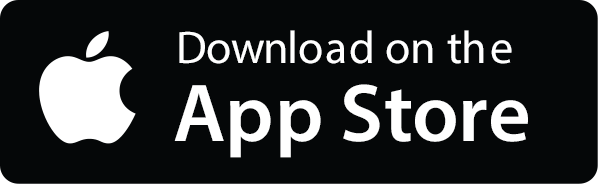
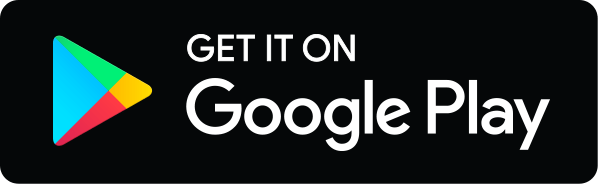
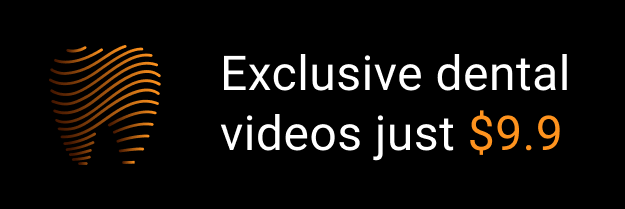