Fig. 1.1
(a, b) Normal trabecular bone. (a) Cut surface of a bone biopsy showing trabecular network and intertrabecular spaces. Note the honeycomb-like arrangement of the interconnected horizontal and vertical trabeculae and the nodes connecting them. The density of these “nodes” ensures mechanical strength. (b) Trabecula consisting of parallel layers of collagen fibrils. These lamellae ensure flexibility of bone (Reprinted with kind permission of Springer Business and Media from [1])
Modeling is continuous during skeletal development, then it is greatly reduced and ceases completely after skeletal maturity, while remodeling takes place throughout life. Modeling results in changes in the shape and size of the bone, while remodeling maintains but usually does not change size and shape. It is carried out by two major bone cells: the osteoclasts which resorb the bone and the osteoblasts which form the bone [1]. Characteristic of active osteoclasts are their “ruffled borders” which lie directly on the surface of the bone. Howship’s lacunae are formed as the osteoclasts carve out the bone [1]. Osteoblasts form an epithelial-like lining at the surface of the bone and are connected by gap junctions. They synthesize osteoid and the organic bone matrix and are responsible for its mineralization. When osteoblasts are embedded in the bone matrix, they become osteocytes. Osteocytes are essential for survival of the bone; if deficient osteocytes are not replaced, the involved bone cannot be maintained and a sequestrum is formed, rejected, and removed [1].
Hyperactive, abnormally activated osteoclasts are characterized by a higher resorptive capacity and therefore a high destructive potential, so that numerous osteoblasts need months to repair an osteolytic lesion accomplished by a few osteoclasts in a week [1]. Deregulation of osteoclasts is the main cause of nearly all osteopathies such as osteoporosis (systemic) or osteolysis (local), potentially accompanied by spontaneous fractures and hypercalcemia. Therefore, antiresorptive drugs such as bisphosphonates and denosumab which effectively reduce osteoclastic activity are of major clinical importance in the treatment of a variety of bone disorders especially osteoporosis and metastatic bone disease [1].
Bisphosphonates
Historical Review
The bisphosphonates constitute a group of pharmacological agents first synthesized in the 1880s but developed over the past 40 years for diagnosis and treatment of disorders of the bone and anomalies of calcium metabolism. The fundamental research carried out by H. Fleisch in the 1960s laid the ground work for the rapid development of the bisphosphonates in medicine [1–3].
The starting point was provided by the natural pyrophosphates which have a central P-O-P binding. Pyrophosphate was widely employed in industry due to its ability to dissolve calcium carbonate. Consequently, pyrophosphates were used in washing powders and other soapy solutions to inhibit scale formation. Interestingly, today they are also used worldwide in toothpaste to prevent and to reduce plaque formation. Due to its strong affinity for calcium phosphate and therefore for bone, pyrophosphate can be bound to 99mTc and utilized for scintigraphy of the skeleton (bone scans) [1].
Moreover, in vivo studies demonstrated an inhibitory effect of pyrophosphates on calcification. Various forms of ectopic calcification could be effectively avoided by parental, but not by oral, administration. However, there was no influence on osteoclastic resorption due to enzymatic splitting of pyrophosphate when taken orally (half-life of only 16 min) [1].
The bisphosphonates were then discovered during the search for analogues of pyrophosphate. They have similar physical and chemical effects but are resistant to enzymatic splitting and to metabolic breakdown. This is because, in contrast to the P-O-P binding of pyrophosphate, the P-C-P binding of the bisphosphonates is stable and above all cannot be broken down enzymatically so that their activity is retained. This switch of the binding from P-O-P to P-C-P represented a genuine breakthrough which enabled the development of the potent bisphosphonates which are now in use for therapy of disorders of bone all over the world [1].
The first medical application of a bisphosphonate was published in the Lancet in 1969. A 16-month-old baby, diagnosed as having progressive myositis ossificans, was successfully treated with oral etidronate to inhibit the extraosseous calcification [2].
Subsequently, H. Fleisch and co-workers demonstrated, by means of animal experiments, that bisphosphonates inhibit osteoclastic bone resorption and thereby achieve a positive calcium balance. The rapid advances in the diagnosis and therapy of the osteopathies is thus closely bound up with the history of the bisphosphonates [1, 3–5].
During the past 30 years, new, more potent bisphosphonates have been developed. These have now been extensively applied in medicine, particularly in the fields of osteology, orthopedics, surgery, as well as in hematology, and particularly in oncology. All osteopathies characterized by excess (absolute or relative) of osteoclastic activity are now treated with bisphosphonates and more recently denosumab, and it should be noted that this comprises about 90 % of all disorders of the bone [1]. Bisphosphonates are now the major drugs used in the treatment of postmenopausal osteoporosis and represent the first-line therapy in the majority of patients. The latest applications of bisphosphonates include their administration for prevention of osseous metastases (administered during adjuvant chemotherapy), for alleviation of bone pain, and for their modulation of the immune and stromal systems in the bone marrow and the bone [1].
Chemistry
Bisphosphonates are analogues of pyrophosphates which occur physiologically and in which the oxygen atom of the central P-O-P structure has been replaced by carbon, resulting in a P-C-P group (see Fig. 1.2), and this exchange has made them resistant to heat and enzymatic hydrolysis. These bisphosphonates exert strong effects on bone. Further substitutions have enabled synthesis of a series of biologically active bisphosphonates, each of which has its own characteristic potential activity and effect on bone. Therefore, every bisphosphonate has to be evaluated individually [1].
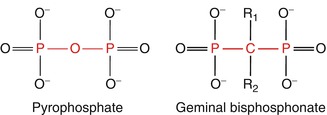
Fig. 1.2
Chemical structure of pyrophosphate and of bisphosphonates (Reprinted with kind permission of Springer Business and Media from [1])
For practical purposes, the bisphosphonates are subdivided into chemical groups according to the alphabetic order of the side chains:
-
Bisphosphonates without nitrogen substitution: etidronate and clodronate
-
Aminobisphosphonates: pamidronate and alendronate
-
Aminobisphosphonates with substitution of the nitrogen atom: ibandronate
-
Bisphosphonates with basic heterocycles containing nitrogen: risedronate, pyridine-ring, and zoledronate, imidazole-ring
The bisphosphonates used to be given in grams, now only milligrams, are given because of their greatly increased potency [1].
Pharmacodynamics
The bisphosphonates are poorly absorbed when taken orally, but this is compensated for by their greatly increased potency – even 1 % of a given dose is effective! They are distributed in the body via the blood stream, stored in the bones, and excreted unchanged by the kidneys. Interactions with other pharmaceutical agents have not been observed. Four compartments of bisphosphonate distribution are distinguished; these determine their pharmacodynamics (Fig. 1.3): the gastrointestinal tract, blood, bone, and kidneys [1].
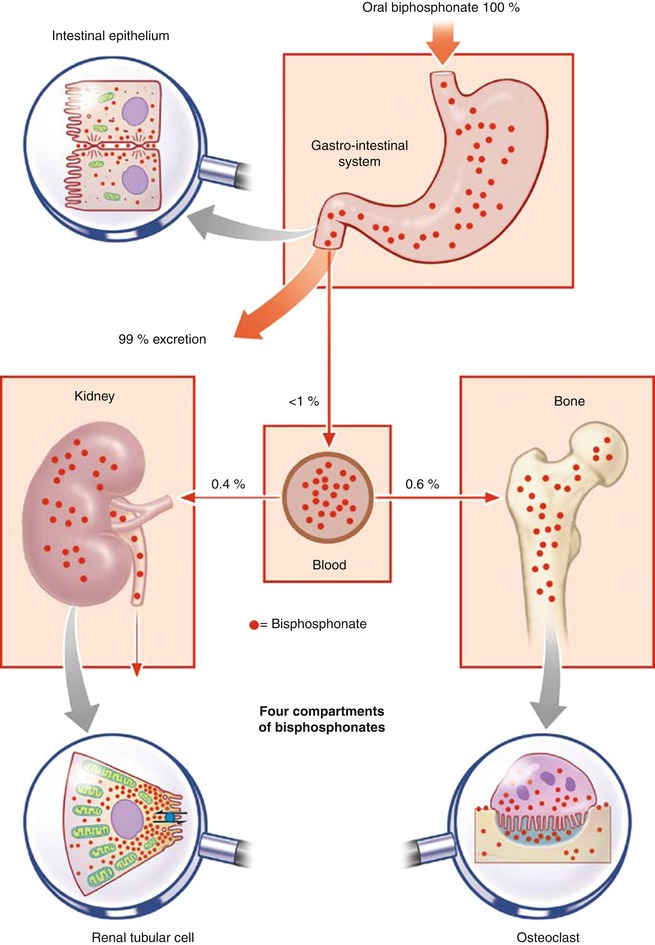
Fig. 1.3
Diagrammatic representation of the four compartments of bisphosphonate absorption and excretion: the gastrointestinal tract, blood, bone, and kidney (Reprinted with kind permission of Springer Business and Media from [1])
Administration and Absorption
Bisphosphonates may be taken orally as tablets or given intravenously as infusions (Fig. 1.3). The intestinal absorption of modern bisphosphonates is minimal to low. It varies from <1 to 3 %. However, as mentioned above, these doses are effective [1].
Two characteristics of bisphosphonates are responsible for their poor absorption: their low affinity for lipids, which hinders transport through membranes and into the cell, and their polarity, their negative charge, which prevents paracellular transport. Bisphosphonate absorption is further decreased when ingested together with food, especially food rich in calcium, such as milk and milk products because bisphosphonates form insoluble chelates with the calcium in these products [1].
Distribution Half-Life
Bisphosphonates are bound to albumin in the blood. There are big differences in the strength of the albumin bonds (from 22 % for zoledronate to 87 % for ibandronate) and therefore in the time it takes for the bisphosphonates to be eliminated from the plasma. The half-life of zoledronate in the plasma is only 1–2 h, while that of ibandronate is 10–16 h. But the half-life in the bone is much longer [1].
Bisphosphonates from the plasma are actively bound to the surface of the bones, especially in the resorption lacunae where they are attached to calcium (Fig. 1.4a, b). The amount of deposition depends on the extent of resorption surface of bone available [1].
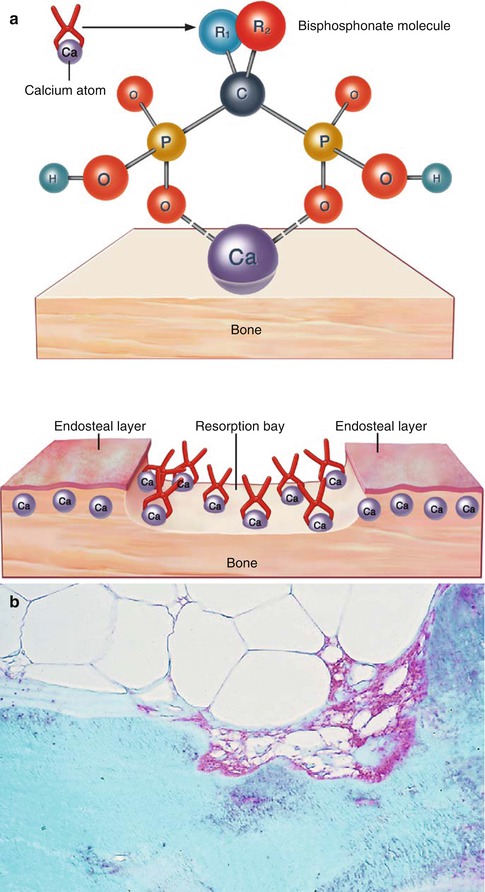
Fig. 1.4
(a) Molecular structure of bisphosphonates: they are stable analogs of pyrophosphate with a central P-C-P binding instead of the P-O-P. The various bisphosphonates are distinguished one from another by the ligands R1 and R2. The bisphosphonates depicted here as small tongs are deposited on the surface of the bone in the resorption lacunae. Here, they are taken up by osteoclasts or incorporated into the bone by osteoblasts. (b) Deposition of bisphosphonate (red) on bone in a resorption lacuna and in the cytoplasm of an osteoclast visualized by means of an antibody to ibandronate, in sections of a plastic embedded undecalcified iliac crest biopsy taken from a patient 2 days after 6 mg ibandronate IV (Reprinted with kind permission of Springer Business and Media from [1])
Affinity to Bone
By binding to hydroxyapatite, bisphosphonates accumulate at sites of bone resorption and are selectively internalized by actively resorbing osteoclasts. The different bisphosphonates have different affinities for hydroxyapatite crystals.
These differences in binding affinities and effects on mineral surface properties are likely to be reflected in the clinical differences among these bisphosphonates: uptake and retention on the skeleton, diffusion of the drug within the bone, release of absorbed drug from the bone, potential recycling of the desorbed drug back onto bone surface, effects on mineral dynamics, and effects on bone cellular function [1].
Uptake and Desorption of Bisphosphonates
Few studies have addressed the question of how bisphosphonates actually enter the cell. Since no specific transport mechanisms have yet been elucidated, the assumption has been made that bisphosphonates are taken up from the surrounding fluid by nonspecific pino- and endocytosis. Bisphosphonates have been demonstrated in the cytoplasm, in mitochondria, and in other organelles within the cytoplasm of the osteoclasts. Relatively speaking, macrophages, a cell line to which osteoclasts belong, are also active in their uptake. However, the concentration of bisphosphonates in extraosseous cells is very low, which explains the lack of toxicity and the paucity of side effects in these tissues [1].
Twenty to fifty percent of the bisphosphonate in the plasma is deposited on the bone and the rest is eliminated by the kidneys into the urine. There are considerable differences between the various bisphosphonates with respect to their elimination. Bisphosphonates exhibit a very strong affinity for hydroxyapatite crystals which are avid bisphosphonate grabbers, and this “binding” process is strictly pH-dependent, so that when, during active resorption, the interface between osteoclasts and bone becomes strongly acidic, the previously bound bisphosphonate is released from its binding to calcium. In contrast to the blood (half-life of 1–15 h), the half-life on the surface of the bone varies from 150 to 200 h; but once inside the bone, and after the resorption cavity has been filled by the osteoblasts, the bisphosphonates remain attached even for years [1, 6].
Skeletal retention varies with the different bisphosphonates and a major factor in retention is the rate of bone turnover and the amount of bone surface available [7]. This retention in bone is similar to that of substances such as tetracyclines, fluoride, and strontium. The prolonged surface attachment of bisphosphonates explains their extended duration of action. The earliest pharmacological effect is manifest 24 h after administration [1].
While some will enter the circulation and will appear in the urine, it is not known whether and to what extent the released bisphosphonate will be active for the suppression of bone resorption. In all studies with alendronate, risedronate, and pamidronate, cessation of bisphosphonate treatment given for 2–7 years was not associated with a rebound increase in bone turnover and rapid bone loss, as it occurs after stopping hormone therapy. These results support the hypothesis that some of the embedded bisphosphonate that is released later is active again at the bone surface [1, 8].
Elimination of Bisphosphonates
Bisphosphonates are eliminated without prior metabolism via the kidneys. This renal clearance of bisphosphonates is accomplished by glomerular filtration as well as active tubular excretion. Bisphosphonates are passively bourne by the blood stream to the kidneys; the quantity depends on the concentration gradient of the bisphosphonate in the blood. Bisphosphonates released from the surface of the bone (T½ 150–200 h) also reach the kidneys by way of the blood stream and are actively eliminated by the proximal tubules [1].
Consequently, excretion of bisphosphonates given by intravenous infusion is multiphasic – a fast biphasic elimination from the blood stream, followed by a lengthier phase with a final elimination half-life of several days. Even after administration of a number of doses, accumulation in the plasma does not occur [1].
About half of the amount of bisphosphonate given at any time is excreted unchanged by the kidneys within 24 h. The half-life time of the bisphosphonates in renal tissue is very variable. It is clear that these differences are responsible for differences in toxicity to the kidney, particularly if and when administration is repeated. Therefore, when dealing with patients with impaired renal function, precautionary measures have to be applied [1].
Actions of Bisphosphonates
Clinically, bisphosphonates act almost exclusively on bone as outlined above. The mechanisms of action of the bisphosphonates include the following:
The most important therapeutic action of bisphosphonates is inhibition of bone resorption, which commences within 1–2 days after administration, regardless of the route and frequency of administration. The total amount given determines the overall effect. The reduction in bone resorption is accompanied by a positive calcium balance (Fig. 1.5
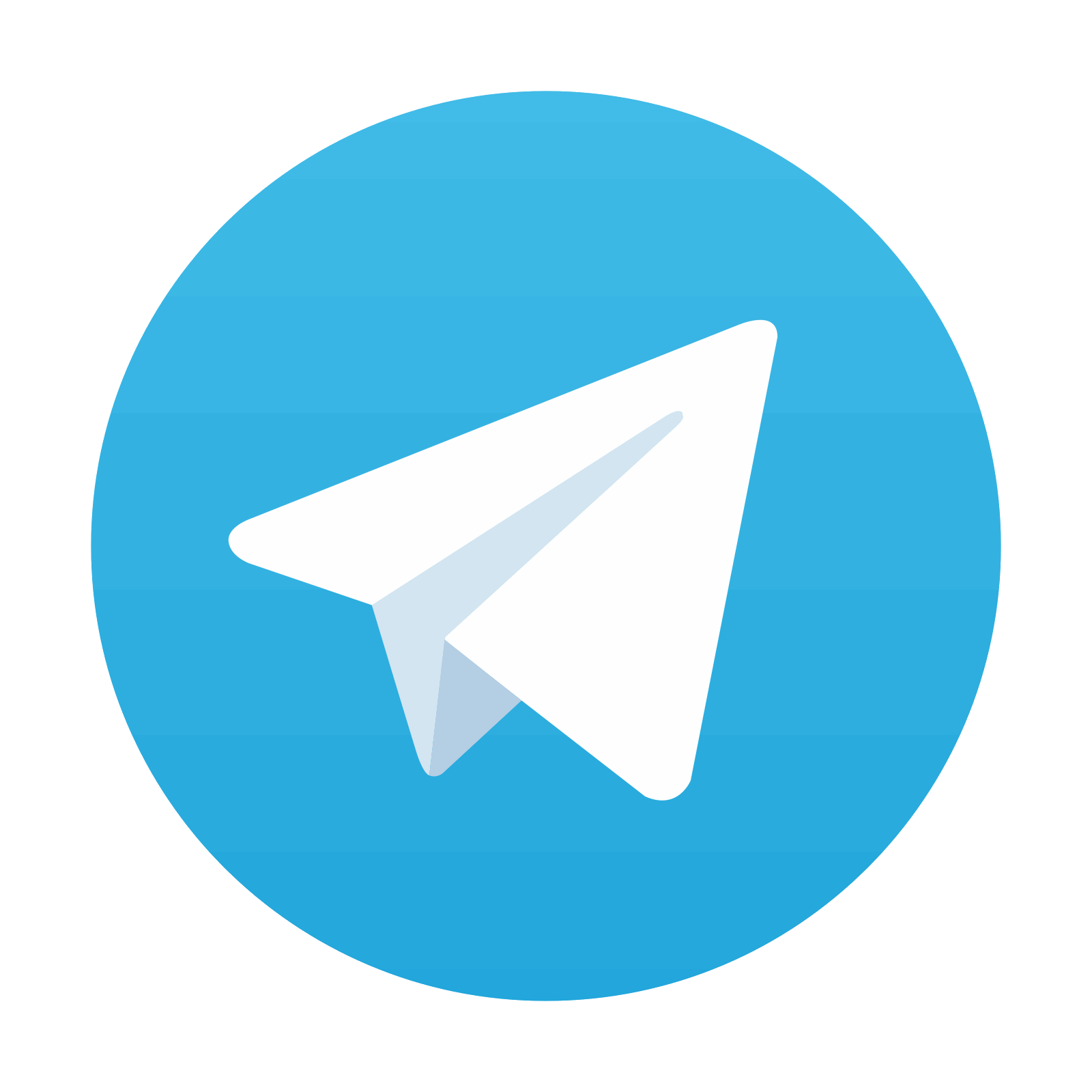
Stay updated, free dental videos. Join our Telegram channel

VIDEdental - Online dental courses
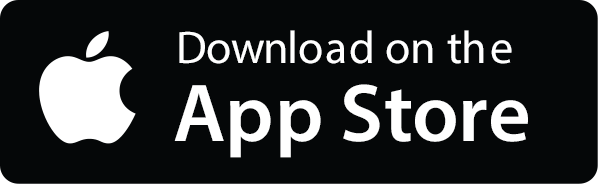
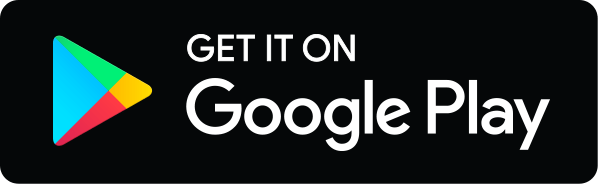