© Springer-Verlag Berlin Heidelberg 2009
Margaritis Z. PimenidisThe Neurobiology of Orthodontics10.1007/978-3-642-00396-7_10
10. Orthodontic Avenues to Neuroplasticity
(1)
Marathonos Street 22, 152 33 Halandri, Athens, Greece
10.1 Introduction
The sensory and motor areas of the cerebral cortex are not rigidly hard-wired genetically. Neurogenesis and neurophysiology are dynamic. The allocation of a given region is a dynamic consequence of a series of interactive processes. The overall organization is not static, but derived from the dynamics itself. In visual development, for example, the retina and then the geniculate body and finally the cortex become organized, each deriving an organizing stimulus from the chaotic excitations established at the previous level. This cortical dynamic plasticity is preserved into later life, where injury, compensation, or a major new learned skill can result in development of new functional areas or in significant rearrangement of existing areas. For example, in a person studied before and after becoming a real-time translator at the United Nations, live positron emission tomography (PET) showed the development of a whole new language area [194].
Neurogenesis begins with embryogenesis, where neurons migrate up the glial cellular scaffold to make specific synapses. Neurogenesis is accompanied by growth and migration, and also by programmed cell death. Selective patterned cell death during development generates the architecture of the brain and its organization in maps that represent the external and internal worlds. This is readily appreciated by considering the sensory maps. For example, regions of the cerebral somatosensory cortex contain maps of the mouth plan, where the oral sensory modalities are anatomically represented in the precentral and postcentral gyri [2, 36].
Memory mechanisms have been built into the rules governing the architecture of the mouth in the gyri, so that activation of regions of the oral map by sensory experiences, such as for instance touch or pain, results in generation of action potentials in the neurons devoted to these modalities, resulting in sensory perception of the tactile or pain stimulus. The activation of the tactile or pain regions of the mouth in the somatosensory cortex is then relayed in the motor regions that plan and execute the movement of the mouth in response to oral sensory stimulation by experience. Thus, map structure leads to map function, which forms our reality. This implies that since the oral sensation and perception phenomena have a memory–learning component build into the brain through the experiences, the brain has a plasticity which is brought into play with changing degrees and types of oral sensory stimulation or sensory deprivation [2].
This interrelationship between the cerebral cortical structure and function in response to changes in oral experiences is not considered in the classical theory and practice of orthodontics, of how the optimum of health, function, and esthetics, of the stomatognathic system may be obtained. Orthodontists may assume that only the dental alveolar structures can be altered by conventional orthodontic therapy, and may not suspect that the orthodontic changes, at least in the occlusion of the teeth, can modify the function and the development of the brain or structures within it.
10.2 Principal Components of Brain Function: The Neuron
The most rudimentary functional unit in the brain is the neuron, which typically has a cell body, a dendritic tree, and an axon as its output limb. The dendritic tree is the portion of the neuron where synaptic inputs are received. Some neurons receive tens of thousands of synaptic inputs distributed over their dendrites. These inputs may be either excitatory or inhibitory in nature; that is, they may result in a postsynaptic electrical potential that makes the dendritic cytoplasm either relatively less or more electronegative with respect to the extracellular space. Action potentials are properties of axons, while excitatory and inhibitory postsynaptic potentials occur at synapses. The excitatory and inhibitory postsynaptic potentials probably account for most electroencephalographic and magnetoencephalographic signals [151].
10.3 Bioenergetics
All the described activity requires energy, and it has been estimated that about 70% of the energy consumed by the brain is used for maintenance of the ionic gradients required for the initiation and propagation of postsynaptic potentials and action potentials. The remainder of the energy presumably is used for maintenance of the structural integrity of the cell and for various synaptic functions, including the synthesis and packaging of neurotransmitters [151].
The energy supplied to the brain is derived almost exclusively from glucose metabolism. The primary sources of brain glucose are glycogen stored in astrocytes and blood glucose. Some evidence suggests that glycogen within astrocytes is broken down to glucose and used within the astrocytes themselves, leaving neurons dependent on blood glucose for their energy needs. Blood glucose is transported across the blood–brain barrier by means of a saturable transport mechanism, the capacity of which can be modified to meet changes in local neural activity. Once within the cell, the glucose molecule is phosphorylated to glucose-6-phosphate by the enzyme hexokinase; this reaction is the critical point in the regulation of the rate of glycolysis. Glycolysis results in the net production of two adenosine triphosphate (ATP) molecules and a small amount of lactate. Usually, this bioenergetically inefficient glycolysis (anaerobic metabolism) is coupled with more efficient oxidative phosphorylation (aerobic metabolism). The final result is the production of between 30 and 35 ATP molecules per molecule of glucose, as well as the generation of substrates for the neurotransmitters acetylcholine, γ-aminobutyric acid, aspartate, and glutamate [151].
Interestingly and importantly, it has been suggested that with cortical activation, regional cerebral blood flow and glucose consumption increase out of proportion to blood oxygen extraction. In other words, regional cerebral blood flow and glycolysis are increased, but oxidative phosphorylation is not. Such decoupling of oxidative phosphorylation results in a relatively lower concentration of deoxyhemoglobin in the venous blood draining activated brain tissue [153].
10.4 Functional Brain Imaging in Orthodontics
Until very recently, scientists could only speculate about the brain’s role in our personality and decision-making skills. We did not have advanced tools to look at the functioning of the brain and thus made many false assumptions about its impact on our lives. With the advent of sophisticated brain-imaging techniques, we are now answering questions about the brain’s role in behavior that have practical applicability to our lives.
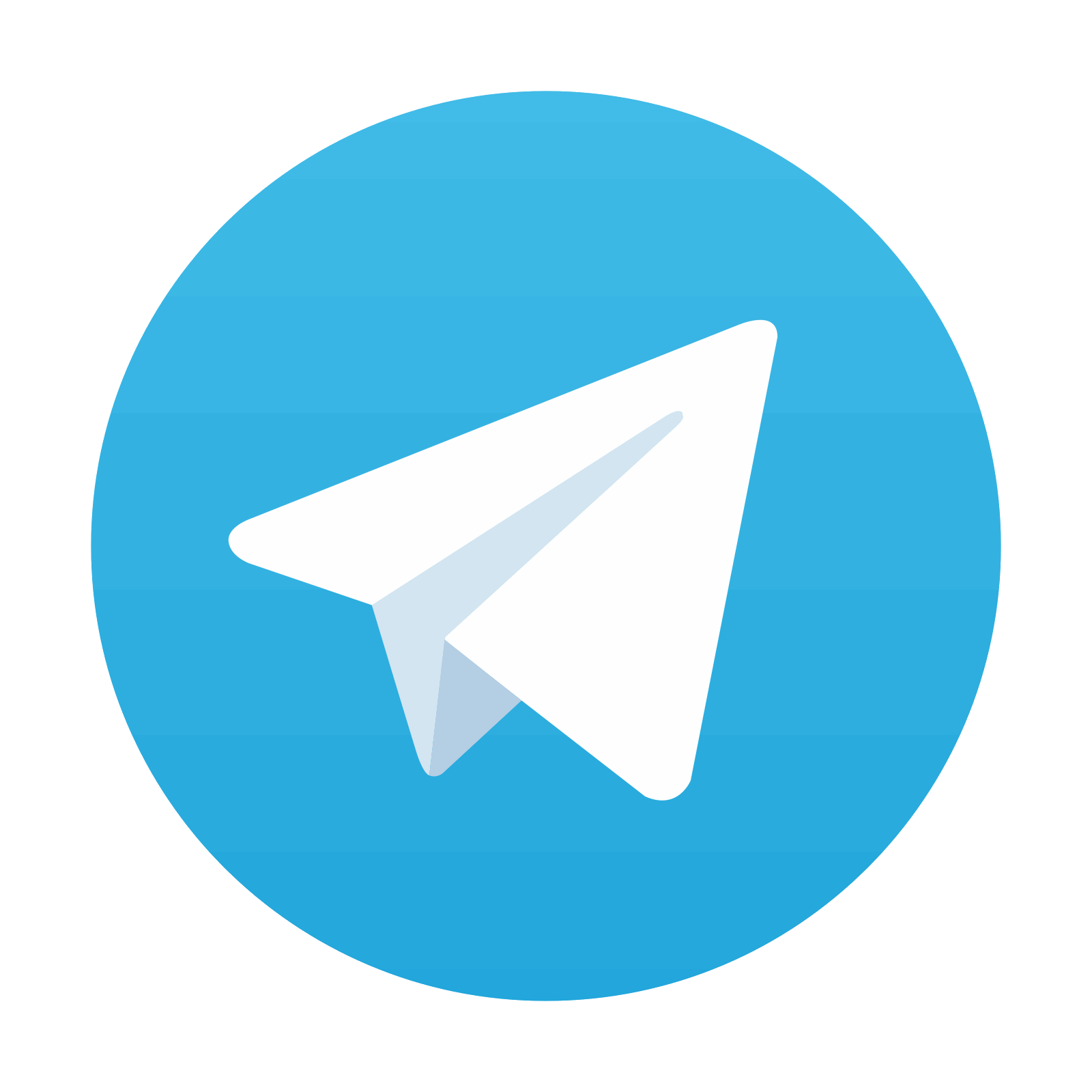
Stay updated, free dental videos. Join our Telegram channel

VIDEdental - Online dental courses
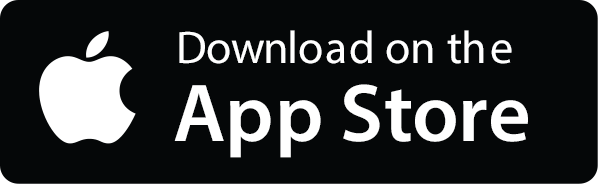
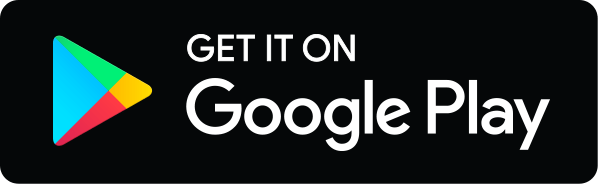