Abstract
Objective
Pit and fissure sealants with antibacterial and remineralization properties have broad application prospects in caries prevention. The objectives of this study were to: (1) develop a novel pit and fissure sealant containing CaF 2 nanoparticles (nCaF 2 ) and dimethylaminohexadecyl methacrylate (DMAHDM); and (2) investigate the effects of nCaF 2 and DMAHDM on biofilm response and fluoride (F) ion release for the first time.
Methods
Helioseal F was used as a control. Bioactive sealants were formulated with DMAHDM and nCaF 2 . Flow properties, enamel shear bond strength, hardness and F ion releases were measured. Streptococcus mutans ( S. mutans ) biofilms were grown on sealants. Biofilm metabolic activity, lactic acid production, colony-forming units (CFU), and pH of biofilm culture medium were measured.
Results
Adding 5% DMAHDM and 20% nCaF 2 did not reduce the paste flow and enamel bond strength, compared to control (p < 0.05). Hardness of sealants with 20% nCaF 2 and DMAHDM was higher than control (p < 0.05). The F ion release from 20% nCaF 2 was much higher than that of commercial control (p < 0.05). The sealant with DMAHDM reduced the S. mutans biofilm CFU by 4 logs. The pH in biofilm medium of the new bioactive sealant was much higher (pH 6.8) than that of commercial sealant (pH 4.66) (p < 0.05).
Significance
The new bioactive pit and fissure sealant with nCaF 2 and DMAHDM achieved high fluoride release and strong antibacterial performance. This novel fluoride-releasing and antibacterial sealant is promising to inhibit caries and promote the remineralizaton of enamel and dentin.
1
Introduction
Dental caries is a chronic oral disease that develops from biofilm accumulation and sugar exposure on the dental surface that affects most populations throughout the world and has been considered the most important global oral health burden. In spite of decades of significant decline, caries remains a global public health burden [ ]. It is estimated that around 44% of all people worldwide are suffering from untreated caries in primary and permanent teeth [ ]. Resin-based materials are increasingly used in dentistry due to their esthetics and photo-cure ability. Extensive efforts have been made to improve the resin compositions, curing conditions, and reduce polymerization shrinkage [ ]. Nonetheless, replacing the failed resin restorations accounts for 50%–70% of all restorations performed [ ]. The prevention of caries is important because caries may lead to speech, aesthetic and psychological problems, abnormal tongue habits and masticatory defects.
The occlusal surfaces of the permanent molars are the most susceptible to caries. Although the occlusal surfaces of children’s teeth constitute 12.5% of total dental surfaces, 85% of total caries incidence is seen in these areas [ ]. One major reason is that the pits and fissures of permanent molars are vulnerable sites for caries lesions due to morphology and plaque accumulation [ ]. Ninety percent of carious lesions originate in the pits and fissures of the occlusal surfaces of permanent molars [ ]. The use of caries-preventive approaches, such as community water fluoridation, topical fluoride therapy, plaque control, and dietary sugar control, has reduced the caries prevalence, with a greater effect on smooth surface caries reduction [ ]. However, the occlusal pit and fissures are generally too narrow (about 0.1 mm wide) for a standard toothbrush and saliva to reach for effective cleaning, thereby allowing bacteria and food to be mechanically retained [ ]. The complex morphology of pits and fissures make them much more susceptible to caries than smooth surfaces. The biofilms in pits and fissures can produce organic acids that can cause the loss of minerals.
The placement of pit and fissure sealants is an effective method for prevention of caries. For example, it was shown that the use of sealants on the first permanent molar reduced the need for further restorations and prevented caries [ ]. Sealants are classified into three groups: resin-based sealants; glass ionomer cement-based sealants; and polyacid-modified resin sealants [ ]. Nowadays, the most-used pit and fissure sealants are resin-based sealants based on urethane dimethacrylate (UDMA) or bisphenol A-glycidyl methacrylate (BisGMA) monomers that require either chemical or light activation for polymerization. The light-cured BisGMA-based resins are the most commonly used, owing to its reasonable flowability, good wettability and physical properties that are superior to those of other types of sealants [ ]. The sealant is applied to a tooth surface to provide a physical barrier that prevents growth of biofilm by blocking nutrition [ ]. The capacity of a sealant to prevent dental decay relies on the ability of the sealant material to thoroughly fill the pits, fissures, and/or morphological defects and remain completely intact and bonded to enamel surfaces for a lifetime [ ]. However, resin-based materials have a number of challenges [ ], such as accumulation of more biofilms/plaques [ ], and microleakage along the sealants margins which can provide pathways for new invading bacteria from the oral environment [ , ]. Eradication of dental biofilm is very difficult. Mechanical cleaning like brushing or flossing the teeth is not sufficient. Therefore, it would be highly beneficial for the pit and fissure sealant to have antibacterial properties.
The fourth generation of resin-based sealants have fluoride-releasing capabilities [ ]. Fluoride is well documented as an anticariogenic agent, as it could increase the dissolution-resistance of the tooth structure, enhance remineralization, and hinder demineralization [ ]. The enhancement of remineralization is the major mechanism that fluoride can inhibit caries, and the anticariogenic effect depends on the amount of fluoride that the material can release [ ]. It is believed that topical application of fluoride on the teeth leads to the formation of a calcium fluoride-like material which acts as a reservoir of fluoride ions. However, studies showed that the fluoride ion release from topical applications of conventional fluoride formulations was very limited [ ].
Nanotechnology has unique physical, chemical and biological properties that can be used to overcome the shortcomings of traditional dental treatments [ ]. The term ‘nanotechnology’ was first introduced by Richard Feynman and described materials having a structural range of 1–100 nm in at least one of the dimensions of the material [ ]. In dentistry, nanoparticles played an increasing role [ ]. Studies showed that adding titanium dioxide (TiO 2 ) nanoparticles improved the mechanical properties [ ], adding silver nanoparticles helped to inhibit biofilm growth [ ], and adding amorphous calcium phosphate nanoparticles inhibited dental caries [ ].
Recently, calcium fluoride nanoparticles (nCaF 2 ) were synthesized using a spray-drying system [ ]. The nCaF 2 particle size ranged from about 15 nm–335 nm, with a median (50th percentile) particle size of 56 nm. The small size of nCaF 2 provided a high surface area for the nanoparticles to enable more ion releases using a relatively lower filler level [ ]. In a previous study, the spray-dried nCaF 2 were incorporated into a dental resin composite, which showed relatively a high mechanical strength and the release of high levels of fluoride ions [ ]. A previous study demonstrated the ability of nCaF 2 to hinder Streptococcus mutans ( S. mutans ) biofilm growth [ ]. However, to date, there has been no report on the incorporation of the nCaF 2 into dental sealants.
Quaternary ammonium methacrylates (QAMs) were incorporated into dental resins to achieve antibacterial activities [ , ]. When the positively charged QAMs come in contact with the negatively charged bacterial membrane, lyses of the bacterial cell wall occurs due to the electrical imbalance [ ]. 12-methacryloyloxydodecylpyridinium bromide (MDPB) was incorporated into adhesives and showed significant reduction of bacterial growth [ ]. A QAS cavity-cleanser was reported to have the abilities of antimicrobial and anti-proteolytic activities [ ]. Methacryloxylethyl cetyl dimethyl ammonium chloride (DMAE-CB) was incorporated into a commercial pit and fissure sealant, which suppressed the growth of S. mutans [ ]. The alkyl chain length (CL) influenced the hydrophobicity of QAMs. QAMs with longer CL produced more potent antibacterial effects, and CL of 16 appeared to be the critical point for the alkyl chain to extend without burying the quaternary ammonium sites [ ]. Dimethylaminododecyl methacrylate (DMAHDM) with CL of 16 was incorporated into adhesive [ ] and composite [ ], and showed substantial reduction in biofilm growth and viability, without inducing bacterial drug-resistance [ ]. However, there has been no report on pit and fissure sealants containing nCaF 2 and DMAHDM.
Accordingly, the objectives of this study were to develop the first pit and fissure sealant containing nCaF 2 and DMAHDM, and to investigate the effects on biofilm response, mechanical properties and fluoride ion release ability. It was hypothesized that: (1) the new pit and fissure sealant containing nCaF 2 and DMAHDM would have good mechanical properties matching a commercial control sealant; (2) the new pit and fissure sealant would have greater and more sustained fluoride release than commercial sealant control; (3) the new sealant would possess a potent antibacterial ability to greatly reduce S. mutans biofilm viability and acid production, and increase the biofilm pH, compared to commercial sealant.
2
Materials and methods
2.1
Synthesis of nCaF 2
The nCaF 2 was synthesized via spray-drying as described previously [ , ]. Briefly, a two-liquid nozzle (Visco Mist, Lechler, St. Charles, IL) was employed to allow two solutions to be mixed at the time of atomization. A calcium solution (calcium hydroxide, Ca(OH) 2 at 2 mmol/L) and a fluoride solution (ammonium fluoride, NH 4 F at 4 mmol/L) were prepared using reagent grade chemicals and simultaneously fed to the nozzle. The feed rate was 10 mL/min and the solution was atomized into a heated air stream of approximately 70 ℃ in a glass column (VM770-48, VM Glass, Vineland, NJ). The reaction of Ca(OH) 2 and NH 4 F led to the formation of CaF 2 and NH 4 OH: Ca(OH) 2 + NH 4 F → CaF 2 + NH 3 ↑ + H 2 O↑. The nCaF 2 suspended in the flow were trapped in an electrostatic precipitator (Mist Buster, Air Quality Engineering, Minneapolis, MN). The NH 4 OH was removed as NH 3 and H 2 O vapors with the air flow. The collected nanoparticles were verified to be CaF 2 in a previous study, with particles sizes ranging from 15 nm to 335 nm and a median particle size of 56 nm [ ].
2.2
Synthesis of DMAHDM
DMAHDM was synthesized using a Menschutkin reaction where a tertiary amine group was react with an organohalide. The reaction products were generated at virtually quantitative amounts and required minimal purification [ ]. Briefly, 10 mmol of 2-(dimethylamino) ethyl methacrylate (DMAEMA, Sigma-Aldrich, MO, USA) and 10 mmol of 1-bromohexadecane (BHD, TCI America, Portland, OR, USA) were combined with 3 g of ethanol in a 20 mL scintillation vial. The vial was stirred at 70 ℃ for 24 h for the reaction to occur. The solvent was then evaporated, yielding DMAHDM as a clear, colorless, and viscous liquid [ ].
2.3
Preparation of pit and fissure sealant containing nCaF 2 and DMAHDM
BisGMA and triethylene glycol dimethacrylate (TEGDMA) (Esstech, Essington, PA) were mixed at a mass ratio = 1:1, and rendered light-curable with 0.2% camphorquinone (CQ) and 0.8% ethyl 4- N,N -dimethylaminobenzoate (4E) (all mass %) [ ]. For mechanical reinforcement, the resin was filled with barium boroaluminosilicate glass particles which were silanized with 4% 3-methacryloxypropyltrimethoxysilane and 2% n -propylamine (Caulk/Dentsply, Milford, DE) [ ]. The total mass fraction of nCaF 2 plus glass fillers was 50% to yield a flowable paste; the specific filler levels for nCaF 2 and glass were listed in the next section. DMAHDM was incorporated into the BisGMA-TEGDMA resin (referred to as BT) at DMAHDM/(BT + DMAHDM) mass fractions of 10%. Since the resin mass fraction in the final sealant was 50%, the DMAHDM mass fraction in the sealant was 5%. This DMAHDM mass fraction was selected following previous studies [ , , ].
2.4
Flow test
The following seven sealants were tested for the paste flow property.
- (1)
Helioseal F (Commercial sealant control);
- (2)
50% BT + 0% DMAHDM + 0% nCaF 2 + 50% glass (Experimental sealant control);
- (3)
45% BT + 5% DMAHDM + 0% nCaF 2 + 50% glass (referred to as 5%DMAHDM+0%nCaF 2 );
- (4)
45% BT + 5% DMAHDM + 10% nCaF 2 + 40% glass (referred to as 5%DMAHDM+10%nCaF 2 );
- (5)
45% BT + 5% DMAHDM + 15% nCaF 2 + 35% glass (referred to as 5%DMAHDM+15%nCaF 2 );
- (6)
45% BT + 5% DMAHDM + 20% nCaF 2 + 30% glass (referred to as 5%DMAHDM+20%nCaF 2 );
- (7)
45% BT + 5% DMAHDM + 25% nCaF 2 + 25% glass (referred to as 5%DMAHDM+25%nCaF 2 ).
A commercial pit and fissure sealant with fluoride release (Helioseal F, Ivoclar Vivadent, Liechtenstein) served as a control (referred to as “Commercial sealant control”). The fillers in Helioseal F consisted of fluorosilicate glass and silicon dioxide, with a filler lever of 40.5%. Helioseal F is photo-cured and indicated to seal pits, fissures, and foramina caeca.
The paste flow was measured following the International Organization for Standardization (ISO) Specification 6876/2012 and ANSI/ADA2000 [ ]. A volume of 0.05 mL of the sealant paste was placed on a glass plate of 40 mm × 40 mm. A second glass plate weighing approximately 20 g was then placed on the top of the sealant paste, followed by a weight of 100 g to make a total mass of 120 g. Ten minutes after the commencement of mixing the paste, the weight was removed and the maximum and minimum diameters of the compressed sealant paste disk were measured using a digital caliper, and the average diameter was calculated. The flow test was used to help choosing the optimal mass fractions of nCaF 2 and glass fillers.
2.5
Enamel shear bond strength testing
Twenty-five healthy, caries-free, extracted human third molars were collected with donor consent, under a protocol approved by the Institutional Review Board (IRB) of the University of Maryland. All teeth were cleaned to remove adherent tissue and debris, and the crowns were sectioned into four surfaces (mesial, distal, oral, buccal) with a diamond disk (REF 5000484U0, Brasseler, USA). This process resulted in 100 tooth surfaces, and 70 tooth surfaces with good quality were randomly assigned to seven groups (n = 10/group). All tooth surfaces were embedded in self-curing methyl methacrylate resin (REF 1845, Lang, IL, USA). Each enamel surface was polished with 600-grit SiC paper under running water for 10 s to create a standardized smear layer. All enamel surfaces were rinsed with a water spray and dried with water and oil-free air, then were etched with 37% phosphoric acid gel (Total Etch, Ivoclar Vivadent, Schaan, Liechtenstein) for 30 s [ ]. The tooth surfaces were rinsed with a water spray and dried with pressurized air for 5 s until a chalky-white enamel surface was visible. The teeth were then inserted into the sample jig (ISO 29022, Ultradent Products, South Jordan, UT, USA), and the cylindrical plastic mold (Button Mold Insert, ISO 29022, Ultradent Products, Inc., South Jordan, UT, USA) was lowered to achieve a gap-free fit on the etched enamel surface. The sealant material was applied in two steps. Each layer was light cured for 20 s with a light-curing unit, then the plastic mold was carefully removed. The procedure rendered a sealant cylinder with a 2.37 mm diameter perpendicular to the enamel surface, as required by ISO 29022 (2013). The specimens were stored in distilled water at 37 ℃ for 24 h and then tested for shear bond strength using a Universial Testing Machine (MTS, Eden Prairie, MN, USA). The notched-edge fixture with the shear blade was placed over the composite cylinder on the aligned specimen. After precise positioning of the shear blade over the cylinder, the load was applied at a constant crosshead speed of 1 mm/min until the bond failed. The enamel shear bond strength = 4 P/(πd 2 ), where P is the load at failure, and d is the diameter of the bonded area of the pit and fissure sealant on the tooth surface [ ].
2.6
Measurement of hardness
The sealant with 25% nCaF 2 was not included in hardness test due to a decrease in enamel shear bond strength. Three pit and fissure sealants were selected for hardness measurement to determine the influence of nCaF 2 and DMAHDM on hardness: Helioseal F control, 0%DMAHDM+20%nCaF 2 , and 5%DMAHDM+20%nCaF 2 . This was because the 20% nCaF 2 yielded a good enamel bond strength while having a high level of F ion release.
Disks were made using molds with a diameter of 10 mm and thickness of 0.8 mm. Sealant was light-cured for 1 min and stored in a NaCl solution (133 mmol/L) buffered with 50 mmol/L HEPES (pH = 7.4; 37 ℃) for 24 h. Vickers hardness was measured. A Vickers diamond indenter was used at a load of 20 g and a dwell time of 10 s.
2.7
Measurement of fluoride ion release
The aforementioned three sealants were tested for fluoride release. A NaCl solution (133 mmol/L) buffered with 50 mmol/L HEPES (pH = 7.4; 37 ℃) was used to immerse the specimens. As in previous studies, three specimens of approximately 2 mm × 2 mm × 12 mm were immersed in 50 mL of solution to yield a specimen volume/solution of 2.9 mm 3 /mL [ , ]. The concentrations of fluoride ion released from the specimens were measured at 1, 2, 4, 7, 14, 28, 35, 42, 49, 56, 63, 70, 77, 84, 91, and 98 days. At each time point, aliquots of 0.5 mL were removed and replaced by fresh solution. The amount of fluoride release was measured with a combination of a fluoride ion selective electrode and a reference electrode (Orion, Cambridge, MA). The collected solutions were combined with equal volume of total ionic strength adjustment buffer (TASAB) solution (Fisher, Fair lawn, NJ). Fluoride standard solutions ranging from 1 × 10 −6 to 1 × 10 −3 mol/L were measured to form a calibration curve, which was used to determine the fluoride ion concentration.
2.8
S. mutans biofilm formation
The following five groups were tested for biofilm experiments.
- (1)
Helioseal F (Commercial sealant control);
- (2)
50% BT + 0% DMAHDM + 0% nCaF 2 + 50% glass (Experimental sealant control);
- (3)
50% BT + 0% DMAHDM + 20% nCaF 2 + 30% glass (The purpose of including this group was to test the effect of F ion release on biofilm growth);
- (4)
45% BT + 5% DMAHDM + 0% nCaF 2 + 50% glass (The purpose of including this group was to test the effect of DMAHDM on biofilm growth);
- (5)
45% BT + 5% DMAHDM + 20% nCaF 2 + 30% glass (The purpose of including this group was to test the effect of DMAHDM + F ion release on biofilm growth).
The mold with a diameter of 10 mm and thickness of 1 mm was used to fabricate disks. The sealant was light-cured for 1 min between transparent Mylar strips. The cured disks were immersed in 200 mL of distilled water and magnetically-stirred with a bar at a speed of 100 rpm for 1 h to remove any uncured monomers. The disks were then sterilized with ethylene oxide (AnproleneAN 74i, Andersen, Haw River, NC) and de-gassed for 3 days, following the manufacturer’s instructions [ ].
The use of S. mutans (ATCC 700610, UA159) (American Type Culture, Manassas, VA) was approved by the University of Maryland IRB. S. mutans was chosen because it is a cariogenic, aero-tolerant anaerobic bacterium and it is the primary causative agent of dental caries. Brain heart infusion broth (BHI) (Becton, Sparks, MD) supplemented with 1% sucrose (pH = 7.4) was used as growth medium. 10 μL of stock bacteria was added into 10 mL of BHI broth (pH = 7.3) and incubated at 37 ℃ with 5% CO 2 for 16 h. Then, the S. mutans culture was diluted by 10-fold in the growth medium to form the inoculation medium for subsequent experiments. Each pit and fissure sealant disk was placed in a well of a 24-well plate, and 1.5 mL of the inoculation medium was added to each well. The samples were incubated at 5% CO 2 and 37 ℃ for 24 h. Then each disk with biofilm was transferred to a new 24-well plate containing 1.5 mL of fresh growth medium, and cultured for another 24 h. This totaled 48 h of incubation, which was shown to form relatively mature biofilms on resins, as shown in previous studies [ , ].
2.9
Live/dead assay of S. mutans biofilms on sealant resins
Pit and fissure sealant specimens with 2-day biofilms were washed with phosphate buffered saline (PBS). Then the biofilms on disks were stained using a live/dead kit (Molecular probes, Eugene, OR). Live bacteria were stained with Syto 9 to produce green fluorescence, and bacteria with compromised membranes were stained with propidium iodide to produce red fluorescence. The stained disks were imaged using an inverted fluorescence microscope (Eclipse TE2000-S, Nikon, Melville, NY) [ ]. Three disks were evaluated for each group. Five images were collected at random locations on each disk, yielding 15 images per group.
2.10
MTT metabolic activity of S. mutans biofilms on sealant resins
The 3-(4,5-dimethylthiazol-2-yl)-2,5-diphenyltetrazolium bromide (MTT) assay is a colorimetric assay that measures the enzymatic reduction of MTT, a yellow tetrazole to formazan [ ]. Pit and fissure sealant discs with 2-day biofilms were transferred to a new 24-well plate. 1 mL of 0.5 mg/mL MTT was added to each well and incubated at 37 ℃ in 5% CO 2 for 1 h. After 1 h, the biofilm specimens were transferred to a new 24-well plate. An aliquot of 1 mL of dimethyl sulfoxide (DMSO) was added to solubilize the formazan crystals. The discs were incubated for 20 min in the dark at room temperature. After 20 min, 200 μL of the DMSO solution in each well was transferred to a new 96-well plate, and the absorbance at 540 nm was measured via a microplate reader (SpectraMax M5, Molecular Devices, Sunnyvale, CA). A higher absorbance is related to a higher formazan concentration, which indicates a higher metabolic activity in the biofilm.
2.11
Lactic acid production of S. mutans biofilms on sealant resins
Pit and fissure sealant disc with 2-day biofilms were rinsed in cysteine peptone water (CPW) to remove loose bacteria. Then, the discs were transferred to a new 24-well plate with 1.5 mL of buffered peptone water (BPW) supplemented with 0.2% sucrose in each well. The sealant discs were incubated at 37 °C in 5% CO 2 for 3 h to allow the biofilms to produce acid. After 3 h, the BPW solutions were stored for lactate analysis. Lactate concentrations were determined using an enzymatic (lactate dehydrogenase) method [ ]. The microplate reader was used to measure the absorbance at 340 nm. Standard curves were prepared using a lactic acid standard (Supelco Analytical, Bellefonte, PA).
2.12
Colony-forming units (CFU) of S. mutans biofilms on sealant resins
Biofilms were cultured for 2-days as described above. The biofilms on the disks were harvested in PBS by scraping and sonication (3510R-MTH, Branson, Danbury, CT) for 3 min and vortexing (Fisher, Pittsburgh, PA) for 20 s. The bacterial suspensions were serially diluted and spread onto BHI ager plates for CFU analysis (n = 6). After 48 h incubation at 37 ℃ in 5% CO 2 , the colony number was counted and CFU counts were determined [ ].
2.13
The pH of S. mutans biofilm culture medium
Each sterilized sealant disk was placed in a well of a 24-well plate with 1.5 mL of S. mutans inoculation medium. The samples were incubated at 5% CO 2 and 37 °C for 24 h to form biofilms on the sealant disk. After 24 h, the disk with biofilm was transferred to a new 24-well plate containing 1.5 mL of fresh growth medium and incubated at 5% CO 2 and 37 ℃ for another 24 h. This totaled two days of culture, which could form relatively mature biofilms on resins. The pH of the first 24 h of culture was not measured, because the non-adherent planktonic bacteria in the medium could contribute to the pH changes, while the purpose of the experiment was to determine the pH caused by the biofilms. Therefore, the pH of the biofilm medium was measured at 48 h of culture using a pH meter (Accumet Excel XL25, Fisher, Pittsburgh, PA). In this way, the measured pH was solely related to the relatively mature 2-day biofilms on the resins [ ].
2.14
Statistical analyses
Statistical analysis was conducted with one-way analysis of variance to detect the significant effects of the variables. Post-hoc pair-wise comparisons were performed using the Tukey’s statistic. Parametric statistical methods were employed with the confirmation that the normality and equal variance assumptions of the data sets were not violated. Statistical analyses were performed by SPSS 18.0 software (SPSS, Chicago, IL) at p < 0.05.
3
Results
The sealant paste flow test results are plotted in Fig. 1 A (mean ± sd; n = 10). The nCaF 2 at a mass fraction of 25% reduced the flow of the sealant (p < 0.05). The experimental sealant with a mass fraction of 20% nCaF 2 had a flow that was not significantly different from the control (p > 0.05).
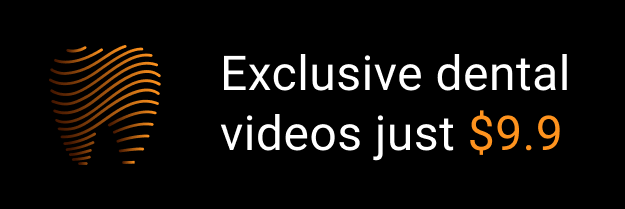