Introduction
The purpose of this study was to evaluate the correlations between bone characteristics, orthodontic miniscrew designs, and primary stability.
Methods
Four different miniscrews were placed in pig ribs. The miniscrews were first scanned with a scanning electron microscope to obtain measurable images of their threads. Subsequently, the maximum insertion torque of the screws and the maximum load value in the pullout force tests were measured; furthermore, bone specimen characteristics were analyzed by using cone-beam computed tomography. For each bone sample, the insertion site cortical thickness as well as both cortical and marrow bone density were evaluated. The nonparametric Kendall rank correlation (tau) was used to evaluate the strength of the associations among the characteristics measured. The nonparametric Kruskall-Wallis test was used to evaluate the differences among the groups, and post-hoc comparisons were assessed by using the Nemenyi-Damico-Wolfe-Dunn test.
Results
A significant dependence was found between pitch and maximum insertion torque (tau, −0.49). Positive correlations were also found between pullout force and maximum insertion torque (tau, 0.64), cortical thickness (tau, 0.36), and marrow bone density (tau, 0.35).
Conclusions
In this in-vitro experimental study, strong correlations were observed among miniscrew geometry, bone characteristics, and primary stability.
Because temporary skeletal anchorage devices do not need osseointegration to obtain clinical success, primary stability is an important factor for their use. Miniscrews are widely used in anchorage control during orthodontic treatment. Their various advantages permit applications in various clinical situations, optimizing efficiency and efficacy. However, the success rate of temporary skeletal anchorage devices has been reported to be relatively low compared with dental implants, with failure rates from 9% to 16.4%.
The primary stability of miniscrews has been associated with many factors, including insertion site characteristics, root proximity, geometric design of the screw, soft-tissue inflammation, operator technique, and magnitude and loading time of the orthodontic force. Cortical density, in particular, is thought to be the most important factor controlling the primary stability of temporary skeletal anchorage devices. Miniscrews obtain their maximum stability mechanically as a result of a tension-compression state generated at the bone-temporary skeletal anchorage device interface. Maximum insertion torque has been suggested as another parameter in the primary stability of screws. Evaluation of bone quality with placement torque measurements was first assessed by Friberg et al. Successive authors used this parameter in describing the primary stability in different experimental setups.
In a clinical study, a maximum insertion torque value within 5 to 10 Ncm showed the best result of resistance to movement, when compared with screws placed at less than 5 Ncm or more than10 Ncm. However, in standard controlled conditions, the torque insertion values were found to be significant when considering the mean resistance to the movement of miniscrews: the higher the maximum placement torque, the greater will be the resistance to movement. A recent patient-based study reported that self-drilling temporary skeletal anchorage devices show statistically significant higher maximum insertion torque values than do predrilling miniscrews in both maxillary and mandibular sites. On the other hand, a higher removal torque value was found for predrilling screws, suggesting that more favorable osseointegration could occur with lower maximum insertion torque values.
A previous experimental study with synthetic bone reported that the geometric properties of temporary skeletal anchorage devices, expressed as the thread shape factor, play a critical role in primary stability. Thread shape factor is calculated as the relationship between the mean thread depth and the pitch of the miniscrew; it was first introduced by Chapman et al, who described the influence of the thread shape factor in resistance to extraction of cancellous bone screws. By increasing this value, the resistance to pullout force becomes better under standard conditions.
A pullout test is generally used to obtain a mechanical evaluation of the retention property of a material or a device when it is embedded in something. In orthodontics, pullout force is basically used to describe the shear strength of miniscrews placed in bone specimens. Wei et al used pullout load to describe the stability of microscrews with different cortical thicknesses at different healing times, and Pickard et al used this test to verify the effect of miniscrew implant orientation on the resistance to failure.
However, to fully understand how screws generate the best stability, the static components of the system (bone quantity and quality as well as screw design) and the dynamic component (insertion phase) should be considered. The static components could be expressed in terms of cortical thickness and bone density, whereas a describing factor of insertion load is the maximum insertion torque value. The purpose of this in-vitro study was to examine how bone characteristics, temporary skeletal anchorage device designs, pullout force, and maximum insertion torque are correlated in describing primary stability.
Material and methods
Four devices were tested in this study: (1) Orthoeasy (Forestadent, Pforzheim, Germany), 1.7-mm diameter and 10-mm length ( Fig 1 , A ); (2) Orthoscrew (Leader Ortodonzia, Cinisello Balsamo, Italy), 1.65-mm diameter and 9-mm length ( Fig 1 , B ); (3) Tomas (Dentaurum, Ispringen, Germany), 1.6-mm diameter and 10-mm length ( Fig 1 , C ); and (4) ORTHOImplant (3M Unitek, Monrovia, Calif), 1.8-mm diameter and 10-mm length ( Fig 1 , D ).
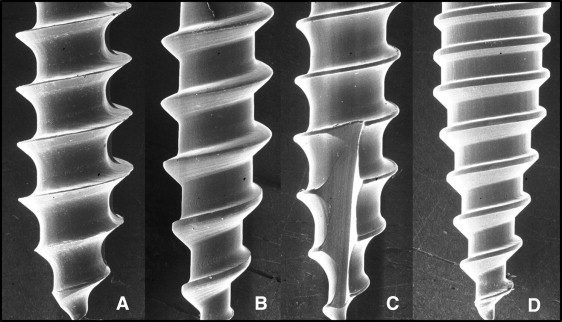
Each device was examined with a 20.00-kV scanning electron microscope (model S-2500; Hitachi, Tokyo, Japan), which provided 3-dimensional image processing on a micrometric scale. Images of the head and the shank of each screw were obtained at 20, 80, 100, and 500 times magnifications and were then converted by using Photoshop CS3 software (Adobe, San Jose, Calif), which permits linear measurement and manipulation of the images. Measurements were obtained in millimeters of the pitch of the screw and the depth of the thread; subsequently, the thread shape factor of each device was calculated. The method to obtain these measurements was similar to that used in a previous study. The mean values are reported in Table I .
Orthoeasy | Orthoscrew | Tomas | ORTHOimplant | P value | |
---|---|---|---|---|---|
D (mm) | 0.346 (0.023) | 0.278 (0.044) | 0.218 (0.013) | 0.116 (0.089) | <0.001 |
P (mm) | 0.828 (0.011) | 1.018 (0.025) | 0.896 (0.005) | 0.572 (0.011) | <0.001 |
TSF | 0.418 (0.030) | 0.273 (0.006) | 0.243 (0.014) | 0.203 (0.017) | <0.001 |
POF (kN) | 0.137 (0.058) | 0.169 (0.053) | 0.122 (0.055) | 0.222 (0.1) | NS |
MIT (Ncm) | 9 (5-11) | 5 (4-12) | 7.50 (6-8) | 11 (11-22) | NS |
CT (mm) | 1.09 (0.13) | 1.19 (0.19) | 0.80 (0.26) | 1.12 (0.58) | NS |
CD (HU) | 1514 (1395-1695) | 1868 (1688-1979) | 2135 (1902-2175) | 2123 (2064-2291) | 0.02 |
MBD (HU) | 971.4 (767.9-1029.7) | 952.8 (718.8-981.5) | 482.1 (434.2-545) | 1103 (1008-1218.5) | 0.02 |
Fresh pig ribs were collected from the same animal on the day of the tests. The samples were sliced into 30-mm sections to obtain 20 specimens. Pig ribs have weaker bone characteristics than the human mandible, and they show heterogeneity in terms of bone density, but the homogeneity of their cortical thickness is even more significant for this kind of test. The pig or cow mandible was also considered as a substrate for the test, but pig ribs showed better homogeneity of cortical thickness. This consideration was further demonstrated in this study. The room temperature was 27°C, and the temperature of the bone was maintained at 37°C ± 1°C in saline solution until the test was carried out. Before the pullout tests, the bone specimen characteristics were analyzed with cone-beam computed tomography (Promax 3D Max; Planmeca Oy, Helsinki, Finland) with a mean exposure time of 12.38 seconds, 66 kV, and a voxel size of 100 μm (isotropic) at Gazzerro Radiological Centre (Genoa, Italy). For each bone sample, an expert operator (H.T.) measured the insertion-site cortical thickness in millimeters as well as both cortical and marrow bone density, expressed in Hounsfield units, using dedicated imaging software (Romexis, Planmeca Oy). Hounsfield units were used as an indication for differences in gray value and hence as an indication of relative density of the bone when compared with other parts of the same specimen. Software allowed precise measurements of cortical density at the miniscrew insertion site, in the middle of the cortical layer, whereas marrow bone density was measured 3 mm under the apical limit of the cortical layer; the set of the points where Hounsfield units doubled was assessed as the border from cortical bone to marrow bone. To obtain these measurements, an implant-like model was created (for each group respecting the device geometry) and virtually placed at the planned insertion site ( Fig 2 ). Successively, all temporary skeletal anchorage devices were implanted to an intraosseous thread of 7 mm depth by using a dedicated thread locker; no predrilling pilot hole was used. Twenty bone specimens were used, 5 for each type of temporary skeletal anchorage device. Maximum insertion torque was measured for each temporary skeletal anchorage device in centi Newton meters with a modified torque wrench (model TT50 SD, MHH Engineering, Bramley, United Kingdom).
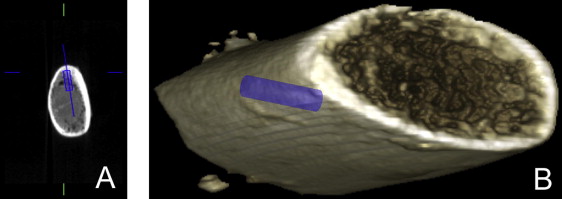
An apposite device was created to guarantee homogeneity and perpendicularity of insertion and for a perfect alignment of screw, bone sample, and load cell. The component parts of this device were an aluminum frame to house the bone samples, the thread locker to prevent screw penetration more than 7 mm, a hollow steel cylinder to encompass each temporary skeletal anchorage device driver, and a cylindrical steel frame to connect these components to the load cell.
Subsequently, the pullout tests were performed. A universal testing machine (model 8501 plus; Instron, Canton, Mass) with a 10-kN load cell was used for the pullout tests; sensibility of the load cell was 0.0001 kN. The software Plus Windows 98 (series IX, version 8; Microsoft Corporation, Redmond, Wash) was used for data acquisition and processing.
A crosshead speed of 2 mm per minute was applied in a controlled environment at 27°C and 70% humidity. The maximum load and screw displacement at peak load were measured. Digital photographs were taken at regular intervals to illustrate the tests ( Fig 3 ).
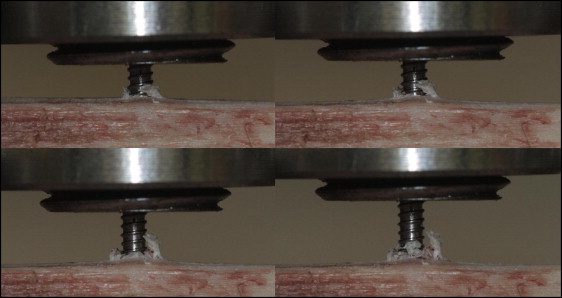
Statistical analysis
Data were analyzed blindly by a statistician (A.S.) with regard to the miniscrews. The nonparametric Kendall rank correlation (tau) was used to evaluate the strength of dependence among the characteristics measured. The nonparametric Kruskall-Wallis test was used to evaluate the differences among the groups, and post-hoc comparisons were carried out with the Nemenyi-Damico-Wolfe-Dunn test. If the data were not skewed, means and standard deviations were calculated for each continuous feature; otherwise, the median and interquartile ranges were obtained. A P value of 0.05 was considered to be statistically significant. Software R (version 2.13.1) was used for computation.
The repeatability of measures of depth and pitch was evaluated with the intraclass correlation coefficient; the intraclass correlation coefficient value for the geometric measurements of the screws was greater than 0.95 for all temporary skeletal anchorage devices; that for bone quantity and density was greater than 0.81.
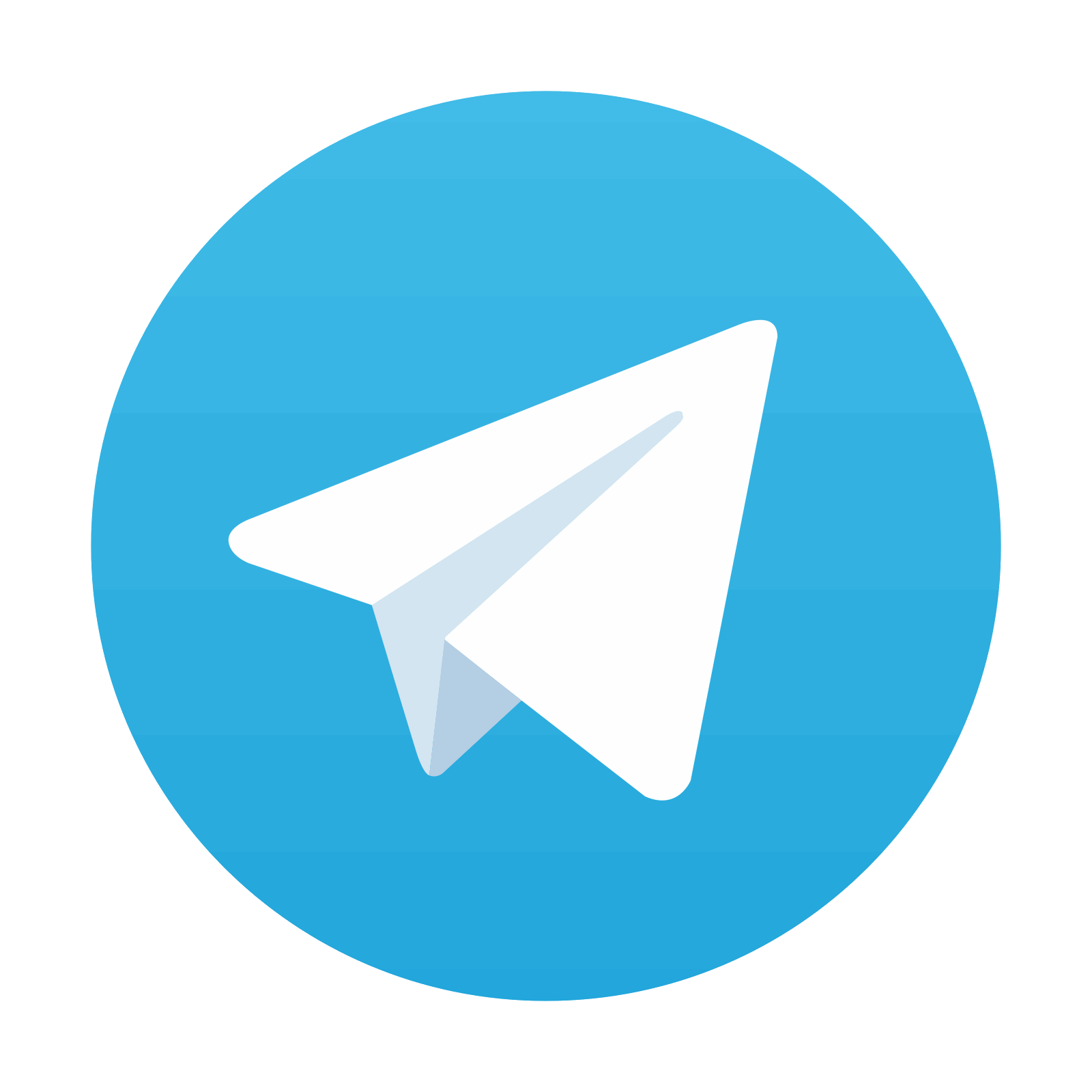
Stay updated, free dental videos. Join our Telegram channel

VIDEdental - Online dental courses
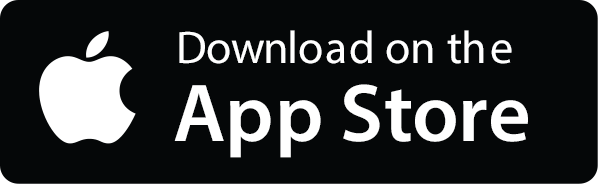
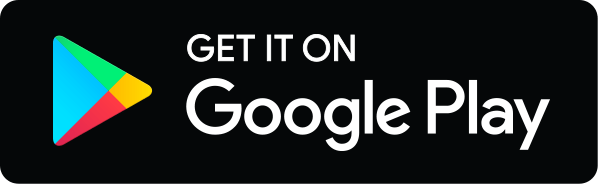