Graphical abstract

Highlights
- •
ML-doped polymeric nanoparticles facilitate functional remineralization of dentin.
- •
ML-loaded polymeric nanoparticles remineralize and seal root dentin.
- •
ML-doped NPs promote amorphous and stoichiometric apatites formation at dentin.
Abstract
Objectives
To investigate the effectiveness of novel polymeric nanoparticles (NPs) doped with melatonin (ML) in reducing dentin permeability and facilitating dentin remineralization after endodontic treatment.
Methods
The effect of undoped NPs and ML-doped NPs (ML-NPs) was tested in radicular dentin, at 24 h and 6 m. A control group without NPs was included. ML liberation was measured. Radicular dentin was assessed for fluid filtration. Dentin remineralization was analyzed by scanning electron microscopy, AFM, Young’s modulus ( E i), Nano DMA-tan delta, and Raman analysis.
Results
ML release ranged from 1.85 mg/mL at 24 h to 0.033 mg/mL at 28 d. Both undoped NPs and ML-NPs treated dentin exhibited the lowest microleakage, but samples treated with ML-NPs exhibited hermetically sealed dentinal tubules and extended mineral deposits onto dentin. ML-NPs promoted higher and durable Ei , and functional remineralization at root dentin, generating differences between the values of tan delta among groups and creating zones of stress concentration. Undoped-NPs produced closure of some tubules and porosities at the expense of a relative mineral amorphization. Chemical remineralization based on mineral and organic assessments was higher in samples treated with ML-NPs. When using undoped NPs, precipitation of minerals occurred; however, radicular dentin was not mechanically reinforced but weakened over time.
Significance
Application of ML-NPs in endodontically treated teeth, previous to the canal filling step, is encouraged due to occlusion of dentinal tubules and the reinforcement of the radicular dentin structure.
1
Introduction
The concept of apical periodontitis involves an inflammatory disease that affects the peri-radicular tissues and bacterial presence in dental pulp. It is the most common sequel of untreated tooth decay and usually origins tooth loss. Nevertheless, apical periodontitis poses an important host defense reaction destined to confine root canal bacteria and avoid them from spreading into adjacent oral tissues [ ]. The interest for strengthening the radicular dentin after periapical periodontitis, abscesses and pulpitis that may indicate endodontic treatment, has increased in the last years [ ]. The structure and treatment of radicular dentin gather several peculiarities [ ]. An endodontically-treated tooth is prone to fracture because the tooth structure damage caused by carious infection and pulpitis, preparation of cavity and canal instrumentation [ ]. Besides, the composition of dentin is not the same across its anatomy, but it changes from the cervical down to the apical zones in root length [ ].
Even when eliminating microorganisms from the root canal system before canal filling is the principal aim of endodontic treatment; sealing and remineralization should also be considered [ , ]. Indicated materials as endodontic sealers have ranged from the employ of silicate aluminum based cements [ ], glass-ionomer [ ], to epoxy resins for radicular canal sealers such as AH-Plus [ ], but most of them have presented different clinical limitations [ ]. Non-resorbable polymeric NPs doped with antimicrobials and reinforcing agents have successfully been tested in advance [ ], but combined anti-inflammatory therapy has not been considered yet. The role of melatonin (ML) in hard tissues has attracted attention [ ]. ML ( N -acetyl-5-metoxy-tryptamine) is an indoleamine that is synthesized and secreted by the pineal gland in a circandian pattern [ ]. Melatonin is also formed in perhaps all organs in quantities by orders of magnitude higher than in the pineal gland and in the circulation [ ]. ML may be involved in the development of hard tissues, as bone and teeth [ ]. ML increases alkaline phosphatase activity, dentin sialoprotein expression and mineralized matrix formation, all of them involved in dentin remineralization. ML may also regulate dentin formation and tooth development [ ]. The generation of reactive oxygen species is always associated with inflammation. It has been demonstrated that, in several endodontic pathologies such as periapical abscess and pulpitis, oxidative stress is an important pathogenetic mechanism [ ]. The free radicals that generate reactive oxygen or nitrogen species are considered to be highly destructive, but directly neutralized by ML [ ].
As stated, ML has been used because of its anti-inflammatory, antioxidant and free-radical-scavenger properties [ , ] and cytoprotective actions [ , ]. A decline in the production of inflammatory mediators, by regulating NF-kB activity, occurs when there is high concentration of ML and contributes to signaling pathway. Although the beneficial effects of ML on periodontal regeneration has been demonstrated in gingival fibroblasts and in vivo and in vitro animal models [ ], its effects in radicular dentin regeneration have not been reported yet. The circulating half-life of ML is approximately 23 min [ ]. Hence, few authors have recommended the use of carriers in ML in order to release it gradually and increase the half-life in the tissues. Constant release of ML by the use of poly-lactic-co-glycolic acid microspheres has been demonstrated to differentiate human mesenchymal stem cells into osteoblasts. Novel ML delivery systems such as ML microspheres and bone-regenerating scaffolds have shown great promise for use in regenerative medicine and dentistry, specifically bone-grafting procedures, to inspire new bone formation [ ], but the release kinenics in all these studies is unknown. Controlled delivery of drugs is determinant for the radicular dentin treatment trying to reinforce the tissue [ ]. Currently, this can be attained as a result of the increased use of nanoparticles (NPs) and the rapid advances of nanotechnology [ , ]. Novel polymeric NPs with anionic carboxylate ( i.e ., COO − ) groups placed along the backbone of the polymer, that may be loaded with calcium (Ca-NPs), zinc (Zn-NPs) or doxycycline (D-NPs) have been previously synthesized to favor dentin remineralization of endodontically treated teeth [ ]. However, in some cases in which apical periodontitis exists it may be valuable to consider the anti-inflammatory, antioxidant and possible remineralizing effects exerted by ML.
Histomorphometric, physical, chemical and mechanical analysis of dentin performance under the administration of exogenous melatonin would be very helpful to tentatively elucidate and confirm the therapeutic role of melatonin in dentin strengthening. Thereby, the present study was aimed to examine the possibility that melatonin might exert its influence on root dentin remineralization. Hence, the purpose of this investigation was to evaluate remineralization and dentin permeability after dentin infiltration with experimental ML-loaded NPs before the endodontic sealer placement. The tested null hypothesis was that melatonin-doped NPs application did not affect, at the short term or overtime, dentin micropermeability, neither remineralization of the endodontically treated radicular dentin.
2
Materials and methods
2.1
Nanoparticles fabrication
PolymP- n Active nanoparticles (NPs) (NanoMyP, Granada, Spain) were obtained through polymerization precipitation. In order to control the process of precipitation, a thermodynamic approach has been used: the Flory-Huggins model based on the Hansen solubility parameters. The model was developed based on the growth of polymeric chains and solvent molecules interactions by hydrogen-bonding, polar and dispersion forces [ ]. The NPs are designed with 2-hydroxyethyl methacrylate as the backbone monomer, the methacrylic acid as the functional monomer and ethylene glycol dimethacrylate as the cross-linker. NPs were functionalized with melatonin. The NPs ML loading process was conducted via immersion of 150 mg of NPs in aqueous solutions of 15 mL of melatonin solutions (containing 50 mg of melatonin) for 2 h at room temperature under constant shaking. The NPs were then left at room temperature until de solvent was completely evaporated, ensuring that all the melatonin remains adhered to the NPs. NPs were resuspended in distilled water at a concentration of 10 mg/mL. To ascertain melatonin liberation profile, HPLC was used. To do this, melatonin was extracted with trichloromethane, and evaporated the organic phase to dryness using a SPD 2010 Speed Vac System (Fisher Scientific, Madrid, Spain). The samples were then analysed by HPLC (Shimadzu Europe GmbH, Duisburg, Germany) using a Waters Sunfire C18 column (150 × 4.5 mm, 5 μm). Melatonin fluorescence was measured using a Shimadzu RF-10A XL fluorescence detector (Shimadzu Europe GmbH, Duisburg, Germany) with 285-nm excitation and 345-nm emission wavelengths [ ]. Three different Eppendorf tubes containing 1 mL of the Melatonin-NPs suspension (at a concentration of 10 mg/mL) were stored at 37 °C. After 24 h, suspensions were centrifuged and the particles were separated from the supernatant. An aliquot of each supernatant was analyzed for melatonin concentration. NPs were washed and 1 mL of fresh distilled water was used to resuspend the NPs at 10 mg/mL until the next supernatant collection. Six different time-points were tested: 24 h, 48 h, 3 d, 7 d, 14 d, and 28 d. The experiment was conducted in triplicate. Two NPs-based groups were established, undoped-NPs and ML-doped NPs (ML-NPs).
2.2
Teeth specimen preparation and nanoparticles application
Forty-eight human mandibular premolars were obtained from donors (18–25 yr of age) with single roots and vital pulp, extracted for periodontal or orthodontic reasons and without caries lesions. The informed consent for inclusion was obtained from all subjects before their participation in the study. The procedure was undertaken following the Declaration of Helsinki, and the approval of the protocol was carried out by the Institution Review Board (#405/CEIH/2017). A random selection procedure was realized to select the teeth samples and they were conserved at 4 °C in 0.5% chloramine T bacteriostatic/bactericidal solution for up to 1 month. The decoronation of the teeth was realized with a low-speed diamond saw (Accutom-50 Struers, Copenhagen, Denmark), and the length of the root was standardized to approximately 12 mm and radiographed at two angulations to check the presence of a single canal. The treatment of the root canal began with the use of Gates Glidden drills (Dentsply Maillefer, Ballaigues, Switzerland), size 2 and 3, to shape the roots coronal third part. Subsequently, a size 15 Flex-o-file (Dentsply Maillefer, Ballaigues, Switzerland) was used to achieve a canal patency. The working length was recorded, and it was established 0.5 mm shorter than apical foramen. ProTaper nickel-titanium rotary instruments (Dentsply Maillefer, Ballaigues, Switzerland) up to size F4 were used for the final instrumentation. At each instrument change, the root canal was irrigated by means of a 27-gauge needle with 0.5 mL of 5% sodium hypochlorite (NaOCl, Panreac, ref. n. 212297), while at the end of canal instrumentation 0.5 mL of a 17% EDTA solution were used for 3 min (MD-Cleanser, Meta Biomed, Chungbuk, Republic of Korea) to remove the smear layer [ ].
Each specimen was finally irrigated with 0.5 mL of 5% NaOCl for 1 min, followed with distilled water for one more minute, and dried with paper points (Dentsply Maillefer). The same operator conducted all procedures. The specimens were randomly separated in 3 groups (n = 10): (i) Control group, (ii) Undoped NPs group, and (iii) ML-NPs doped group. 100 μL of the two different NPs suspensions in ethanol at 10 mg/mL were introduced into the teeth roots using a sterile micropipette. It was left undisturbed for 10 min. Then, the solvent excess was removed by gently blowing with the air syringe until evaporation, without desiccate the dentin. In one of groups, NPs were not applied (control group). Afterwards, in all groups, AH-Plus sealant cement (Dentsply Maillefer, Ballaigues, Switzerland) were introduced into the root using a lentulo spiral and it was compacted into the radicular canal with an endodontic plugger. Immediately, one size 30 guttapercha (guttacore –GC-) cone (Dentsply Maillefer, Ballaigues, Switzerland) was placed and compacted into the canal to working length, in all groups. The excess of gutta-percha and/or cement was removed from the coronal portion of the root canal, and a 3 mm space was created to insert the fluid filtration device. The space was filled with Cavit (ESPE, 3 M, St. Paul, MN, USA) as a provisional restoration [ ]. The adequate obturation of the material along the root canal and the voids absence was evaluated with two angulations radiographs [ ].
2.3
Sealing ability trough the fluid filtration system
After storing the filled root canals in simulated body fluid solution [SBFS: NaCl, NaHCO 3 , KCl, K 2 HPO 4 , MgCl 2 , CaCl 2 , and (CH 2 OH) 3 CNH 2 /HCl (6 N) to buffer the pH (7.4)], 10 teeth from each group (n = 30) were coated with two layers of varnish [ ] up to 2 mm from the root apex. After the removal of the provisional restoration, the coronal part was bound to a plexiglass support with cyanoacrylate adhesive (Rocket, Corona, CA, USA). A 18-gauge needle penetrated 2 mm into the root coronal piece through the Plexiglas support. The other end of the 18-gauge needle was inserted into an 18-gauge polyethylene tubing (R-3603, Tygon, Paris, France) to assess the filled roots microfiltration. A liquid flow sensor (ASL 1600, Sensirion, Staefa, Switzerland) connected between the root specimen and the source of hydraulic pressure was employed to quantify the filled root canals fluid flow. A syringe suspended 70 cm above the sensor and filled with 60 mL of deionized water generated a constant hydraulic pressure of 6.86 kPa. The specimens were stored in SBFS and the medium was refreshed every 2 weeks. The rest of the fluid flow method of the filled root canals was assessed as in Toledano et al. [ ] at different storage times (24 h, 1 week, 3 months and 6 months) (Fig. S1).
2.4
Nanoindentation and AFM analysis
Eighteen teeth were employed for this part of the study. Half of the specimens were studied at 24 h of storage, while the other half was analyzed after 6 months. To isolate regions in the cervical and apical root dentin, two radicular disks were obtained from each root (13.5 and 4.5 mm respectively, above apex), by splitting perpendicularly to their long axis into 1 mm ( + 0.1 mm) thick slices (Fig. S1). The crowns were discarded at the cement-enamel junction, using a water-cooled diamond saw (Accutom-50 Struers, Copenhagen, Denmark). Two samples slabs with a 1 mm thickness were obtained sectioning bucco-lingually the root canal to examine cervical and apical areas (Fig. S1b). 800 up to 4000 grit SiC abrasive papers were used to polish the surfaces. The final polishing steps were done by using diamond pastes through 1 μm down to 0.25 μm (Struers LaboPol-4; Struers GmbH, Hannover, Germany) (Fig. S1). After each polishing step, samples were treated with deionized water for 5 min in ultrasonic bath (Model QS3, Ultrawave Ltd, Cardiff, UK).
2.4.1
Young’s modulus and nano-DMA analysis
A Hysitron Ti-750D TriboIndenter indentation system (Hysitron Inc., Minneapolis, MN) equipped with a commercial nano-DMA package was employed. A Berkovich (three sides pyramidal) diamond tip (tip radius ∼20 nm) was used as the nanoindenter. The calibration of the nanoindenter tip was performed over a fused quartz sample. A quasistatic force setpoint of 5 μN was used. The calibration-reduced modulus value of 1.1400E + 03 N/mm 2 was employed to obtain the best-fit spherical radius (150 nm). Ten intertubular dentin indentations were performed on each specimen at 20 μm next to radicular canal (inner zone) (Fig. S1b). A load of 4000 nN and a time function of 10 s were used to execute the indentations. The tip pressed progressively over the sample (at a constant rate) up to a peak load of 4000 μN. The indentations were performed in an hydrated condition. A drop of ethylene glycol was put over the dentin surfaces to avoid water evaporation during a 25-to-30-min scanning period. The rest of the process was performed as in Toledano et al. [ ] and Toledano-Osorio et al. [ ].
Dentin disks were also subjected to nano-DMA analyses. A dynamic (oscillatory) force of 2 μN was superimposed on the quasistatic signal at a frequency of 200 Hz. Based on a calibration-reduced modulus value of 69.6 GPa for the fused quartz, the best-fit spherical radius approximation for tip was found to be 150 nm, for the selected nano-DMA scanning parameters. Modulus mapping was conducted by imposing a quasistatic force setpoint, Fq = 2 μN, to which it was superimposed a sinusoidal force of amplitude FA = 0.10 μN and frequency f = 100 Hz. The resulting displacement (deformation) at the site of indentation was monitored as a function of time. Data from regions, approximately 20 × 20 μm in size, were collected using a scanning frequency of 0.2 Hz. Specimens were scanned under a hydrated condition. In order to accomplish for this purpose, samples were stored in PBS after polishing to maintain the hydration. Both Young’s modulus ( Ei ) and tan delta (δ) were calculated.
2.4.2
Atomic force microscopy (AFM) and nanoroughness assessments
Topography mappings were obtained with an atomic force microscope (AFM Nanoscope V, Digital Instruments, Veeco Metrology group, Santa Barbara, CA, USA). The images were obtained, in a fully hydrated state, inside a fluids cell of the AFM using the tapping mode with a calibrated vertical-engaged piezo-scanner (Digital Instrument, Santa Barbara, CA, USA). An oscillating cantilever with a 10 nm radius silicon nitride tip (Veeco) contacted intermittently with the dentin surface at the lowest point of the oscillation. Changes in vertical position of the AFM tip at resonance frequencies near 330 kHz provided the height of the images registered as bright and dark regions. 5 × 5 and 2 × 2 μm digital images were recorded with a slow scan rate (0.1 Hz). For each image, 5 randomized boxes (1 × 1 μm) were created to examine the nanoroughness at 24 h and 6 m of storage. Nanoroughness (SRa, in nanometers) was measured with proprietary software (Nanoscope Software, version V7). The rest of the procedure was as in Toledano et al. [ ].
2.5
Statistical analysis
Microleakage, Young’s modulus, tan delta, and nanoroughness were statistically analyzed. As the normality and homoscedasticity conventions of the data were valid, ANOVA and Student-Newman-Keuls multiple comparison tests were employed, with statistical significance preset at p < 0.05. Microleakage, Young’s modulus, tan delta, and nanoroughness were considered dependent variables in each ANOVA model. NPs, storage time, and dentin zone (cervical/apical) were independent influencing factors. Interactions between factors were included in the analysis.
2.6
Raman spectroscopy
The same AFM-analyzed dentin surfaces were subsequently studied with Raman spectroscopy. For this analysis, it was employed a dispersive Raman spectrometer/microscope (Horiba Scientific Xplora, Villeneuve d’Ascq, France) with a 785-nm diode laser through a X100/0.90 NA air objective and with 500 μm pinhole (which means a spatial resolution of 785 nm, approximately). The spectrometer was equipped with a CCD detector (DR-324B-FI-327, Andor Technology LTD, UK). The 785 nm laser was used with 100X objective, and the spectral resolution of the system was 2 cm –1 . Two surface areas of 12 × 12 μm were mapped at dissimilar sites for each sample at X and Y axis with a 0.5 μm spacing (625 points per map) and each spectrum was measured by using 2 s acquisition time with 2 accumulations. Chemical mapping was submitted to K-means cluster (KMC) analysis using the multivariate analysis tool (ISys® Horiba), which includes statistical pattern to derive the independent clusters. The K-means clustering is a method of analysis based on a centroid model which aims to partition “n” observations into “k” clusters in which each observation belongs to the cluster with the nearest mean. The natural groups of components (or data) based on some similarity and the centroids of a group of data sets were found by the clustering algorithm once calculated by the software and the Hierarchical Cluster Analysis (HCA). The observed spectra were described at 400–1700–cm −1 with 10 complete overlapping Gaussian lines, suggesting homogeneous data for further calculations [ ]. Each cluster biochemical content was examined though the average cluster spectra. Using the Hierarchical Cluster Analysis (HCA) and the algorithm calculated by the software, the natural groups of components (or data) based on some similarity and the centroids of a group of data sets was found. At this point, the dentin mineral component was assessed studying the relative presence of mineral, i.e. , the phosphate (960 cm −1 ) peak and area and the relative mineral concentration of phosphate (PO 4 3− ) referred to phenyl (RMC p ), the stoichiometric HAp (963 cm −1 ), the carbonate peak (1070 cm −1 ), and the crystallinity of biological apatites by the value of the 1020/1030 ratio intensity (1020 cm −1 , nonstoichiometric apatites containing HPO 4 2− , CO 3 2− , and vacancies; 1030 cm −1 , stoichiometric apatites) [ , ]. The dentin organic component was analyzed examining the crosslinking at 1032 cm −1 (pyridinium ring vibration), the nature and secondary structure of collagen (C C stretch of hydroxyproline, C C Hydroxilated proline, collagen and lipids and proteins) and the proteoglycans [ , ].
2.7
Field emission scanning electron microscopy (FESEM)
Dentin surfaces were then fixed for 24 h in a 2.5% glutaraldehyde solution in 0.1 mol/L sodium cacodylate buffer. To observe the samples with a field emission scanning electron microscope (FESEM Gemini, Carl Zeiss, Oberkochen, Germany), they were exposed to critical point drying (Leica EM CPD 300, Wien, Austria) and sputter-coated with carbon by means of a sputter-coating Nanotech Polaron-SEMPREP2 (Polaron Equipment Ltd., Watford, UK). An X-ray detector system (EDX Inca 300, Oxford Instruments, Oxford, UK) attached to the FESEM realized an energy-dispersive analysis at designated points.
3
Results
3.1
Melatonin liberation profile
The melatonin liberation profile curve is shown in Fig. S2. It corresponded with the following amount of melatonin expressed in mg/mL at each time point: 24 h, 1.85; 48 h, 0.65; 3 d, 0.37; 7 d, 0.13; 14 d, 0.13; 28 d, 0.033.
3.2
Sealing ability trough the fluid filtration system
The fluid filtration rate (μL min −1 ) at distinct storage time for all groups is shown in Fig. 1 . Significant differences (P < 0.05) were found between groups. All groups showed the same microleakage values after 24 h and 1 w of storage. Control samples promoted the highest microleakage values among groups, at 6 m time point; specimens treated with undoped NPs and ML-NPs performed similar after 6 m storage.

3.3
Nanoindentation
Mean and SD of the modulus of Young ( Ei ) at the two different dentin disks (cervical and apical) are represented in Fig. 2 a. All specimens, regardless the group, performed similar when cervical root dentin was analyzed at 24 h of storage. Samples treated with undoped NPs attained higher Ei than the rest of the groups at apical root dentin. After 6 m of storage all NPs-treated groups attained higher Ei than the control group. Control samples showed an Ei decrease over time, irrespective of the dentin location. Cervical dentin treated with undoped NPs maintained its Ei after 6 m of storage, but Ei decreased at apical dentin. When ML-NPs were used, Ei was similar over time, regardless the dentin location. At 6 m of storage tan delta assessed at cervical dentin in control specimens and those treated ML-NPs obtained higher values than samples treated with undoped NPs. At apical dentin, specimens treated with ML-NPs attained the highest tan delta values ( Fig. 2 b).

3.4
Atomic force microscopy (AFM) and nanoroughness assessments
Control specimens and ML-NPs treated ones attained lower SRa values than those treated with undoped NPs, at both cervical and apical root dentin, after 6 m of storage ( Fig. 2 c). At the apical half of radicular dentin, samples treated with ML-NPs decreased their roughness values overt time. Samples treated with undoped NPs did not modify their SRa values over time ( Fig. 2 c). AFM analysis, in Fig. 3 , permitted to observe dentin control surfaces showing open dentinal tubules with numerous microporosities ( Fig. 3 a,d). Undoped NPs on dentin provoked filled or open dentinal tubule entrances and sights of stress concentration affecting intertubular and peritubular dentin structures with microcraking ( Fig. 3 b,e). Smoother dentin surfaces, strongly mineralized, were observed when ML-NPs were applied on radicular dentin ( Fig. 3 c,f). At 24 h storage, control samples showed both peritubular and intertubular dentin clearly identified (Fig. S3a,d). Tubules appeared completely, partially occluded or empty when undoped NPs were applied on dentin (Fig. S3b,e). Peritubular, intertubular and intratubular mineralization characterized the application of ML-NPs on radicular dentin (Fig. S3c,f).

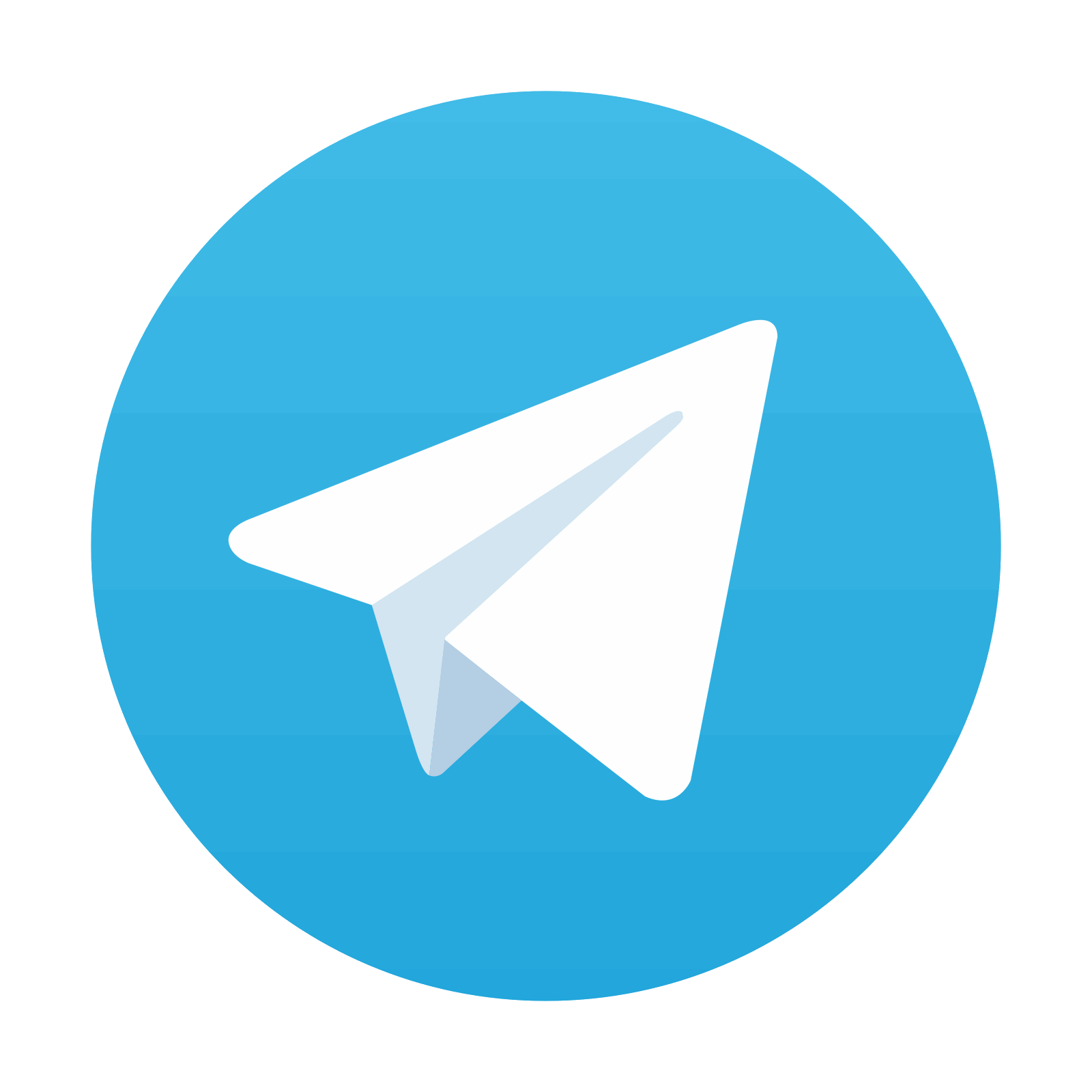
Stay updated, free dental videos. Join our Telegram channel

VIDEdental - Online dental courses
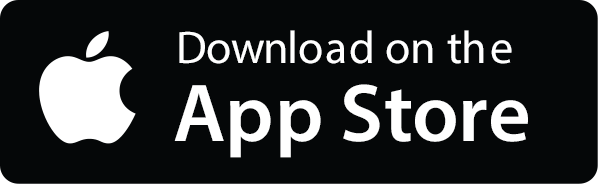
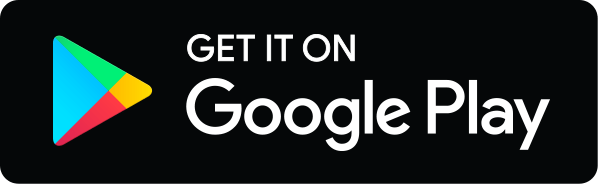
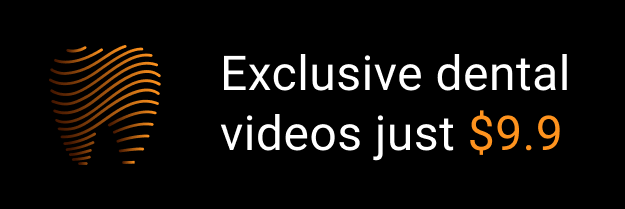