Fig. 3.1
Peripheral and central targets of medications inhibiting nerve signalling in the salivary reflex. The illustration of the salivary reflex shows afferent stimuli arriving via cranial nerves VII and IX at the nucleus of the solitary tract (NST) in the brainstem. Central neural tracts connect to the salivary nuclei, and parasympathetic nerves from the superior salivary nucleus innervate the submandibular (SMG)/sublingual (SLG) glands via the submandibular ganglion, whilst parasympathetic nerves from the inferior salivary nucleus innervated the parotid (P) gland. Not shown is central integration of afferent (chewing, chemical) stimuli from cranial nerve V. Descending tracts from the cortex via the lateral hypothalamus modulate transmission of stimuli in the salivary nuclei and alpha-2 adrenoceptor agonists (e.g. antihypertensives) produce an inhibitory effect on transmission and cause MISGD. Inhibitors of SERT and NET, serotonin and noradrenaline transporters responsible for reuptake, appear to cause MISGD through activation of alpha-2 adrenoceptors by elevating endogenous levels of noradrenaline. The main peripheral target of drugs causing MISGD is cholinergic muscarinic receptors (M3 an M1) on salivary gland acinar cells. Central connections and sympathetic outflow are also shown. Antihypertensive (and maybe also nasal decongestant) drugs may alter saliva protein content by acting on peripheral beta-1 adrenoceptors, but there is little evidence that substantial MISGD is caused through receptors in this pathway
The ages of study subjects have been found to influence salivary flow rates with older age groups showing reduced flow. However, the extent of this reduction appears to be mostly influenced by the increased consumption of medications in the older age groups. The effects of age per se, in a non-medicated population, have been less frequently studied with some researchers finding an effect of age [39, 41] and others suggesting that such an effect is not significant [10] since there is little evidence of age-related (per se) xerostomia. The observed differences between the sexes referred to above need also to be considered in the light of medication. For example [12], a study of older (over 65 years) subjects found that UWMS flow rate in female subjects was 63 % of males. However, this difference was not seen when non-medicated subjects were considered as a separate group.
How is it that subjects might experience MIX in the absence of reduced saliva production? One possibility is that altered salivary composition and properties may affect salivary function including coating and retention on soft tissue surfaces. Osailan et al. studied a group of dry mouth patients with mixed diagnoses including drug-induced dryness, and a substantial proportion (20 %) had signs (scored using a clinical scale) and symptoms (scored using relevant questions and a VAS) of dryness even though their unstimulated salivary flow rate was greater than 0.2 ml/min, well above the accepted figure for hypofunction of 0.1 (or 0.15) ml/min [33]. Analysis of the composition of saliva from patients did not reveal decreased levels of high-molecular-weight mucin (MUC5B), an important glycoprotein in determining the viscoelastic properties of saliva [42]. More recent studies suggest reduced extensional rheology in these ‘higher salivary flow’ dry mouth patients which may be linked with a reduced function on mucosal surfaces (Chaudhury et al. 2014, unpublished findings).
What Are the Mechanisms of MISGD?
Interruption of Reflex Signalling at the Periphery: Afferent Signalling
Secretion of fluid from salivary glands is a nerve-mediated reflex (Fig. 3.1). Secretion from the major salivary glands is evoked by tastants with citric acid and other strong sour tastes evoking high flows of saliva, whilst salt, bitter and sweet evoke less saliva. High salivary flow rates are also produced following activation of mechanoreceptors in the periodontal ligament and mucosae [43]. Minor salivary glands may also increase secretion in response to taste stimulation [44], but perhaps mechanoreceptors responding to movement and tactile stimulation of the mucosa play a more important role in evoking secretion from submucosal labial and palatine minor salivary glands [45, 46]. Interference with afferent signalling does not appear to play a primary role in MISGD although it might be speculated that dietary consumption of softer foods, as a result of insufficient saliva to moisten/process harder foods, may exacerbate a dry mouth condition due to reduced chewing reflex stimulation of salivary glands. Reduced reflex stimulation can result in salivary gland atrophy [47].
Interruption of Reflex Signalling at the Periphery: Efferent Signalling
Salivary secretion is dependent upon autonomic nerve-mediated signals. Under anaesthesia, most salivary glands do not secrete fluid, and there are only a few examples of spontaneously secreting salivary glands, and even in these glands secretion ceases when the autonomic nerve supply to salivary glands is severed [48]. When the parasympathetic nerve supply is stimulated electrically under anaesthesia, large volumes of saliva are evoked, whilst similar stimulation of sympathetic nerves evoked only a small volume of fluid. Dual parasympathetic and sympathetic nerve stimulation experiments under anaesthesia have demonstrated that the individual actions of the nerves, particularly protein secretion evoked by the sympathetic nerve, are augmented, whilst the volume of secretion is maintained in rat parotid [47]. Such dual stimulation experiments are thought to better reflect the events leading to reflex secretion of saliva, since it is expected that both parasympathetic and sympathetic impulses are acting on secretory cells simultaneously. The concept of peripheral sympathetic inhibition of salivary secretion, which later became widely accepted, was appreciated as an experimental artefact over a century ago. Experimental electrical stimulation of the sympathetic nerve supply to salivary glands or use of alpha-1 adrenoceptor agonists in anaesthetized animals leads to a vasoconstriction of glandular blood vessels in addition to activation of parenchymal cells. In contrast under reflex conditions, only sympathetic secretomotor nerve fibres and not vasoactive nerve fibres to salivary glands are activated. Thus vasoconstriction is not part of the salivary reflex.
Denervation studies in the rat have demonstrated that sympathetic impulses make a contribution to the amount of protein secreted under reflex taste stimulation [49]. The importance of an intact parasympathetic innervation is clear when one considers the dryness caused by blockade of the effects of acetylcholine by atropine and its analogues. Blockade of the effects of sympathetically mediated stimuli by the beta-blocker propranolol and its analogues causes reductions in protein concentration of saliva.
Although adrenergic signalling from sympathetic nerves leads to an augmentation of protein secretion by parotid and submandibular glands, mucin secretion from mucous glands such as the rat sublingual gland and human minor glands is dependent upon parasympathetic stimulation and peptidergic stimulation [50]. Thus, although parasympathetic nerve-mediated stimuli appear to universally cause fluid secretion from salivary glands, the role of the sympathetic innervation in evoking protein secretion is variable.
Efferent Signalling and Muscarinic Receptor Antagonists
Salivary secretion is largely dependent upon the activation of muscarinic acetylcholine receptors (mAchRs) on salivary acinar cells by acetylcholine released from parasympathetic nerves [47]. Pharmacological studies suggest that secretion is almost entirely dependent upon signalling through m3AchRs in the rat parotid gland whilst submandibular gland secretion in rat and rabbit is dependent on both m3AchRs and m1AchRs. Selective knockout of muscarinic receptor subtypes in mice indicates that m1AchRs play a role in whole mouth saliva secretion in response to stimulation with cholinergic agonists, including pilocarpine [51, 52]. The m1AchRs are not expressed on all submandibular cells, and activation appears to require higher doses of agonist compared to activation of m3AchR, and studies of calcium signalling in submandibular acinar cells in vitro indicate, unlike m3 receptors, that m1 receptors are not ubiquitously expressed [51].
Given the paramount importance of cholinergic signalling in salivary secretion, it is clear that xerostomia associated with anticholinergic medications (see earlier) is most likely due to medication-induced salivary gland hypofunction (Table 3.1; Fig. 3.1). Oxybutynin, tolterodine, trospium, solifenacin, darifenacin and fesoterodine are muscarinic receptor antagonists commonly used in the treatment of overactive bladder, and each of these drugs blocks M3 and/or M2 receptors, which are the intended targets since the latter mediate smooth muscle relaxation in the bladder. Dry mouth is an ADR of varying severity depending on the drug; for example, tolterodine appears to produces less dry mouth than fesoterodine although each drug interacts with a number of muscarinic receptors including M3 ([53], [54], [55], [56]). Studies on the mouse have compared some prescribed anticholinergics used in the treatment of overactive bladders – oxybutynin, solifenacin and tolterodine. Submandibular gland secretion was less affected by solifenacin and tolterodine compared to oxybutynin, and solifenacin dissociated from muscarinic receptors more easily than oxybutynin [57].
Table 3.1
Pharmacological mechanisms of peripherally acting xerogenic drugs
Drug type
|
Intended action
|
Decreased salivation
|
Mechanism of MISGD
|
---|---|---|---|
Overactive bladder
|
Anticholinergic (M3)
|
Y
|
Anticholinergic (M3)
|
Anti-emetics
|
Anticholinergic
|
Y
|
Anticholinergic (M3)
|
Tricyclic antidepressants
|
SNRI
|
Y
|
Anticholinergic (M3)
|
Antihistamines
|
Anti-H1 receptor
|
Y
|
Anticholinergic (M3)
|
Antihypertensives
|
β-Adr antagonist
|
N (reduced protein secretion)
|
β-Adr antagonist
|
Dry mouth may be offset to a degree through controlled drug-release formulations compared to immediate release [58] as described above. Tiotropium is an inhaled bronchodilator used in the treatment of chronic obstructive pulmonary disease which interacts with muscarinic receptors including M3 and was found to cause dry mouth as an ADR in 9.3–16 % of the subjects compared to 1.6–2.7 % of the subjects in a placebo group [59, 60]. Tiotropium results in more dry mouth symptoms than new LAMA (glycopyrronium bromide). The efficacy of this drug in controlling COPD still needs confirmation by a phase III study [61]. Orphenadrine, procyclidine and trihexyphenidyl are anticholinergics that antagonize muscarinic receptors and can cause dry mouth as a side effect, presumably due to blockade of M3 and M1 receptors.
Medications with an Unintended Antimuscarinic Effect
Tricyclic antidepressants (TCAs) used in the treatment of depression not only inhibit reuptake of serotonin and noradrenaline by transporters in the CNS but interact with muscarinic receptors as demonstrated by competitive radioligand binding assays [62]. TCAs cause dry mouth in a substantial (27 %) percentage of treated subjects [63], and the TCAs amitriptyline and nortriptyline have been demonstrated to reduce stimulated salivary secretion [64–66] whilst amitriptyline and the TCA dothiepin also significantly reduced (58 %) stimulated parotid salivary flow rate.
Early formulated antihistamines such as clemastine, diphenhydramine and brompheniramine used in the treatment of allergy, nausea and vomiting cause varying degrees of dry mouth in subjects, and this appears to be due to an interaction with muscarinic receptors. More specific histamine H1 receptor blockers are less associated with dry mouth as an ADR [1, 22]. Some anti-emetic medications such as scopolamine used in the control of motion sickness have an anticholinergic action and cause hyposalivation and dry mouth through this mechanism.
Central Interruption of Reflex Signalling
Taste, mechanical or pungency signals generate afferent signals in fibres of the facial (CN VII), glossopharyngeal (CN IX) and trigeminal (CN V) nerves. The nucleus of the solitary tract is innervated by the CN VII and CN IX and sends interneurons to the salivary centres, respectively, the superior and inferior salivary nuclei in the medulla oblongata. Interneurons presumably supply the primary sympathetic salivary centres which are located in the upper thoracic segments of the spinal cord although it remains unclear precisely where in this region [67]. Efferent nerve fibres from the salivary nuclei conduct efferent signals via the chorda lingual nerve to the submandibular ganglion and thence to the submandibular and sublingual glands. The parotid gland is supplied by efferent fibres in the glossopharyngeal (tympanic branch) nerve to the otic ganglion and postganglionic fibres in the auriculotemporal nerve (see Fig. 3.1).
The salivary reflex is profoundly influenced by central nerves from other nuclei in the brain supplying the salivary nuclei in the medulla oblongata. The salivary nuclei have various inputs from the frontal cortical areas as demonstrated by nerve-tracing experiments [68]. This central neural activity appears to contribute towards the resting rate of salivary secretion into the mouth since salivary flow rates are lower during sleep and virtually absent during anaesthesia. Retrograde labelling of neurons has demonstrated that the primary parasympathetic salivary centres form connections with the lateral hypothalamus where the regulation of feeding, drinking and body temperature occurs. Both excitatory (gamma-aminobutyric acid containing) and inhibitory (glycine containing) nerves synapse with the salivary centres [67].
Suppression of impulse traffic from the salivary nuclei to salivary glands leading to reduced salivation and dry mouth is most obviously demonstrated during fear and anxiety and, like other autonomic regulations, involves a complex interaction with higher (limbic and cortical) centres in the brain. Different sensory modalities, including auditory, visual and somatosensory, are associated with fear and may potentially impact on salivary secretion through pathways in the amygdala, the hypothalamus and the brainstem.
Previous neuroanatomical studies have shown that there are also cholinergic inputs to the salivary centres from other nuclei including the substantia innominata, pedunculopontine nucleus (PPN) and lateral dorsal tegmental nucleus (LDT). It has recently been demonstrated that neurons in the SSN express M3 and other muscarinic acetylcholine receptors [69].
Since cholinergic neurons from the PPN and LDT are associated with the maintenance of wakefulness and show increasing impulses during wakefulness, it may be that these inputs enhance activity of superior salivary nucleus neurons and increase salivation during wakefulness, whilst reduced impulse input from the PPN and LDT suppresses salivation during sleep and may account for the circadian pattern of resting or unstimulated salivation observed in man [38]. The presence of muscarinic receptors on neurons of the salivary nuclei may also partly explain the observed effects of enhanced salivary secretion evoked by intracerebroventricular injection of pilocarpine or atropine which was found to, respectively, stimulate and inhibit salivation [70, 71].
Central Signalling and Alpha-2 Adrenoceptor Agonists
Clonidine, guanabenz, guanfacine, methyldopa and moxonidine are α2Ad agonists used in the treatment of hypertension and have been found to cause dry mouth in high percentages of treated subjects (see Chap. 3 in [1]). Alpha-2 adrenoceptor (α2Ad) agonists (e.g. clonidine) have been demonstrated to reduce salivary secretion in human subjects (Table 3.2; Fig. 3.1). Studies in animal models indicate that the mechanism of hyposalivation involves central α2Ads which are distributed throughout the central nervous system, and high levels of specific α2Ad binding sites are found in the nuclei of the hypothalamus and amygdala. The lateral hypothalamus projects to the parasympathetic salivary nuclei in the brainstem, and lesions of the lateral hypothalamus produce a typical pattern of degeneration secretion with immediate, transient hypersalivation due to neurotransmitter release being superceded by prolonged impairment of basal salivation and reduction of pilocarpine-induced salivation [72]. Alpha-2 adrenoceptor blockade can increase salivary secretion, whilst α2Ad agonists inhibit secretion [73, 74]. Injection of moxonidine (an α2Ad and imidazoline agonist) reduced salivation induced by pilocarpine (i.p.) in rats, and prior injection of the RX 821002, an α2Ad antagonist, abolished the inhibitory effect of moxonidine [72]. It appears that under physiological conditions, α2Ad signalling is part of a central inhibitory circuit related to the control of salivary gland secretion involving projections running from the rat hypothalamus to the midbrain and hindbrain autonomic centres, including the salivary nuclei. In addition, direct efferent connections exist between the forebrain and the salivary glands. The α2Ad mechanisms on salivary secretion probably involve descending pathways passing through the LH before reaching the brainstem or more caudal autonomic centres related to the control of salivary gland function. Binding studies have shown the existence of high levels of α2Ad in the LH, and moxonidine acting on α2Ad may produce inhibitory effects as a consequence of presynaptic inhibition of noradrenaline or glutamate release or postsynaptic hyperpolarization, both mechanisms consistent with the pre- and postsynaptic location of the α2Ad in the brain. Therefore, moxonidine acting on α2Ad probably located postsynaptically in the LH may inhibit the excitatory mechanisms of the LH activated by pilocarpine to induce salivation [72, 75].
Table 3.2
Pharmacological mechanisms of centrally acting xerogenic drugs
Drug type
|
Intended action
|
Hyposalivation
|
Mechanism of MISGD
|
---|---|---|---|
Antihypertensives
|
α2-Adr agonist
|
Y
|
α2-Adr agonist
|
Tricyclic antidepressants
|
SNRI
|
Y
|
Uncertain – α2-Adr agonist?
|
Antidepressants (non-TCA)
|
SNRI
|
Y
|
Uncertain – α2-Adr agonist?
|
SSRI
|
N
|
N
|
|
Appetite suppressant
|
SNRI
|
Y
|
Uncertain – α2-Adr agonist?
|
SSRI
|
N
|
N
|
|
Opioid analgesics
|
Mu-opioid receptor
|
N
|
Uncertain – α2-Adr agonist?
|
SNRI
|
Y
|
N
|
|
Decongestant (pseudoephedrine)
|
α1-Adr agonist
|
Uncertain
|
Uncertain – α2-Adr agonist?
|
β-Adr agonist
|
N (increased protein secretion?)
|
β-Adr agonist
|
Medications with an Unintended Activation of α2 Adrenoceptors
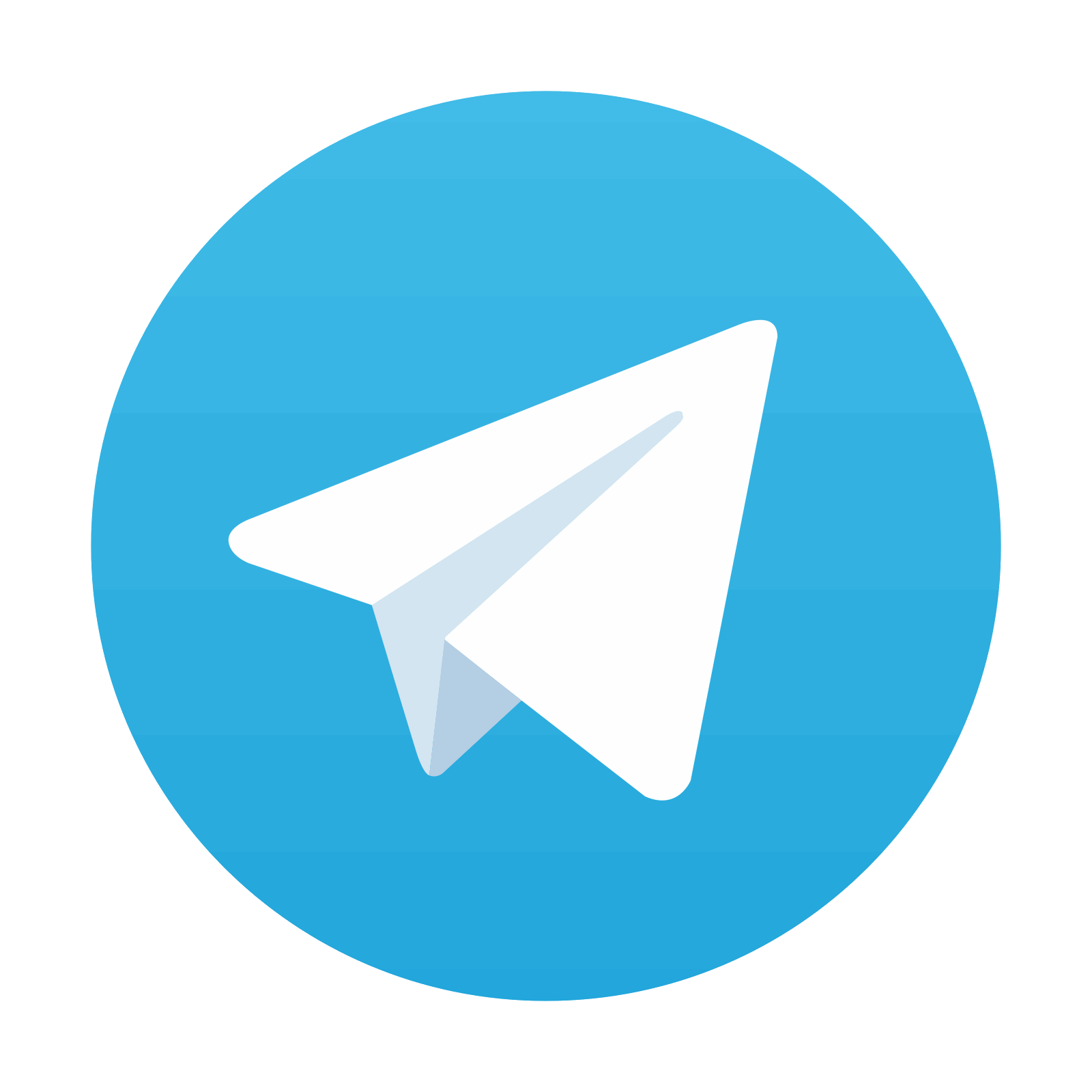
Stay updated, free dental videos. Join our Telegram channel

VIDEdental - Online dental courses
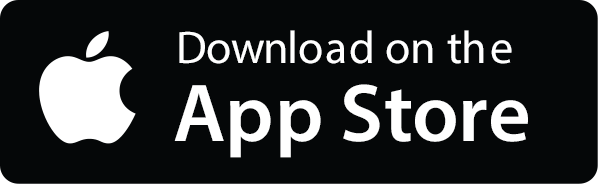
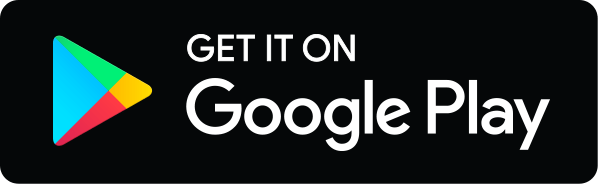