42 Influence of (Chemo-) Radiotherapy on Salivary Gland Function and Its Impact on Quality of Life
Radiation Techniques for Sparing the Salivary Glands
Photon Therapy and Sparing of the Salivary Glands
Proton Radiotherapy and Sparing of the Salivary Glands
Amifostine and Radiation-Induced Xerostomia
Pilocarpine for Preserving Parotid Function
Introduction
Radiotherapy is an important treatment option in patients with head and neck cancer. Their quality of life may be impaired due to the side effects of radiotherapy, such as xerostomia and dysphagia. This chapter focuses on the measurement of xerostomia after radiotherapy and the relationship between xerostomia and the radiation doses and technique used. The use of technical improvements to spare salivary gland function and of amifostine and pilocarpine is discussed.
General Aspects
Treatment
Radiotherapy is the initial treatment of choice for early-stage head and neck cancer located in the nasopharynx, oropharynx, hypopharynx, and larynx, and in some cases the oral cavity. In more advanced cases, when the patient is not suitable for surgery, combined chemoradiotherapy is the treatment of choice, as a meta-analysis by Pignon et al. showed.1 If combined chemoradiotherapy is not possible due to the patient’ s age or comorbid conditions, a combination of radiotherapy with cetuximab may result in cure rates comparable with those achieved using combined chemoradiotherapy, with less toxicity.2
For patients treated with radiation alone and with intermediate-sized tumors, alternative fractionation schedules such as accelerated fractionation and hyperfractionation may result in improved cure rates, with late toxicity comparable with conventional radiotherapy. However, the acute toxicity will be increased in comparison with conventional fractionation.
Xerostomia
Radiotherapy for head and neck cancer generally results in a high radiation dose to normal structures such as the swallowing muscles, epiglottis, and major and minor salivary glands. As a result, patients may experience symptoms of xerostomia as a result of reduced salivary flow. The xerostomia may cause a decrease in the patient’s quality of life.3 In most publications, the mean dose to the parotid gland has been found to correlate with reduced salivary flow and an impaired quality of life as assessed using questionnaire scores.
However, increasing emphasis is being given to the dosage to the submandibular gland, as the submandibular glands produce most saliva at rest. Several hospitals use objective methods such as salivary flow measurements and/or subjective observer-based or patient-based toxicity scoring systems to quantify the xerostomia.4,5
It is well known that the radiation dose to the parotid gland is related to reduced salivary flow. Little is known about the relationship between the radiation dose to the submandibular glands and its influence on salivary flow.
Dysphagia
Xerostomia has been shown to result in an impaired quality of life and oral discomfort.6 In addition to xerostomia, dysphagia is a frequently reported late side effect of radiotherapy. Intensification of treatment, as in accelerated radiation schedules and combined chemoradiotherapy, results in improved tumor control and survival. However, an increase in severe acute mucositis and swallowing dysfunction over the long term is also seen. In a series of 529 patients reported by Langendijk et al., grade 2–4 swallowing dysfunction was observed in 23% of the patients after 6 months, 15.6% after 12 months, and 13.2% after 24 months.7
The treatment modality used has also been found to be a significant prognostic factor, with a high risk of swallowing dysfunction after accelerated radiotherapy and after concomitant chemoradiotherapy. These dysphagia disorders are significantly related to the radiation dosage to the superior and middle constrictor muscles.8 In patients treated for laryngeal cancer, in addition to damage to the pharyngeal constrictors, swallowing disorders may also be caused by damage to the glottic and supraglottic larynx.9
However, xerostomia and dysphagia may be related. In a group of patients treated with radiation alone for oropharyngeal cancer in Utrecht, there was a strong correlation between symptoms of xerostomia and symptoms of chronic dysphagia.10 In patients treated with chemoradiotherapy, Langendijk et al. showed that the patients’ major symptom 1 year after treatment was dysphagia, whereas 2 years after radiotherapy xerostomia was the patients’ most frequent symptom.7 To prevent or to reduce the risk of xerostomia after (chemo-) radiotherapy for head and neck cancer, it is important to be familiar with the dose–response relationship for the salivary glands and objective and subjective measurements of xerostomia. On the basis of the relevant studies, a threshold dosage for salivary glands (parotid, submandibular, and minor glands) can be defined. Using these thresholds, the risk of xerostomia after (chemo-) radiotherapy can be reduced using modern radiation techniques such as intensity-modulated radiotherapy (IMRT) and reliable imaging techniques.
Dysphagia after radiotherapy is not only related to xerostomia but also to damage to the pharyngeal constrictor muscles.
Xerostomia after Radiotherapy
Influence of Chemotherapy
The correlation between xerostomia and chemoradiotherapy in comparison with radiotherapy alone or with no chemoradiotherapy was studied using the Surveillance Epidemiology and End Results (SEER) database.11 In 2000 patients aged over 65, the risk of having xerostomia 2.5 years after treatment was nine times higher for combined chemoradiotherapy and six times higher with radiotherapy alone in comparison with no chemotherapy and no radiotherapy. This finding was based on quality of life measurements.11 In another nonrandomized, prospective study, also based on quality of life measurements, 92 of 214 patients with head and neck cancer were treated with combined chemoradiotherapy.12 In the multivariate analysis, chemotherapy was not found to be a prognostic factor for a reported grade 2 or higher Radiation Therapy Oncology Group (RTOG) xerostomia score. The only significant factors for ≥ grade 2 xerostomia were IMRT and tumor site (oropharyngeal and nasopharyngeal tumors fared worse).12 However, in a smaller study in Leuven including 75 patients, concomitant chemoradiotherapy was associated with a significantly increased risk of xerostomia.13
Combined chemoradiotherapy may increase the risk of xerostomia after treatment.
Influence of Radiotherapy
Measurements of Xerostomia Used in Several Studies
Objective methods of measuring and reporting xerostomia include salivary flow measurement, salivary gland scintigraphy, magnetic resonance sialography, and diffusion-weighted magnetic resonance imaging (MRI). Subjective measurements include observer-based toxicity scoring and patient self-reporting using score questionnaires.
Saliva Flow Measurements
The most commonly applied method is to collect saliva produced by the parotid gland separately using Lashley cups placed on the orifice of the Stensen duct (Fig. 42.1). Saliva produced by the submandibular glands and sublingual glands is collected by suction with a micropipette at the orifices of the Wharton ducts. Stimulated and unstimulated measurements of saliva production have been used. Most of the saliva is produced by the parotid glands in an active phase during daytime, and by the submandibular glands at rest.
Fig. 42.1 Measuring parotid flow using Lashley cups.
The above measurements do not allow measurement of saliva production in the minor salivary glands, such as those in the oral cavity, larynx, and pharynx. Whole saliva production can be collected with the use of spitting, drainage, or weighted cotton rolls inserted into the mouth for a period of time.14 The results of salivary flow measurements vary widely in reports on saliva production due to the different methods used, the duration of collection, and under the influence of other factors such as the use of medications that influence saliva production.15 This wide variation in saliva production data was also demonstrated in a report by Roesink et al.16
In general, saliva production 6 weeks, 6 months, and 1–5 years after radiotherapy is compared with saliva output before radiotherapy. In the literature, a salivary flow equivalent to less than 25% of the preradiotherapy flow is considered to be relevant and is used as a threshold value for the presence of a complication.17
Scintigraphy and Xerostomia
In 1999, Hermann et al. evaluated commonly cited numeric indices in saliva scintigraphic studies and their accuracy for identifying Sjögren syndrome and radiation sialadenitis. The stimulated excretion fraction used in the study was able to distinguish patients with radiation sialadenitis from healthy individuals. The sensitivity and specificity of the test was 73%.18 In a large group of 108 patients treated with radiotherapy for head and neck tumors, studies were carried out after administration of technetium Tc 99 m pertechnetate.19 After 15 minutes, 5% citric acid was administered orally to induce the excretion of saliva. The response to citric acid was evaluated using the salivary excretion factor (SEF), defined as the uptake after excretion per gland as a function of the maximum uptake. The uptake and excretion response were analyzed per patient and subsequently per individual gland. The best correlation between mean parotid dose and complication risk was seen for an SEF ratio of less than or equal to 25% at a time point 6 weeks after radiotherapy.19
These measurements have been compared with parotid flow measurements using Lashley cups. One year after radiotherapy, the broad flow measurements using Lashley cups correlated best with mean parotid gland dose, and these were superior to the use of 99mTc pertechnetate.17 Münter et al. studied not only the parotid, but also the submandibular maximum uptake and relative excretion rate after radiotherapy, and demonstrated a correlation between the mean parotid gland dose and the reduction in the maximum uptake and relative excretion rate. Since almost none of the submandibular glands could be spared in the study, no dose–response relationship could be established for the submandibular glands using these scintigraphic data.20 In a small study in Finland, also using 99mTc pertechnetate scintigraphy, a dose–response relationship was observed for submandibular gland function.21 However, the current data in the literature show remarkably wide variation in glandular function in the submandibular and parotid glands with both direct salivary flow measurements and 99mTc scintigraphy.
Another major drawback of salivary gland scintigraphy is the lack of spatial information. However, single photon emission computed tomography (SPECT) is capable of providing three-dimensional information about salivary gland function and makes it possible to evaluate dysfunction after irradiation of different areas in the parotid and submandibular glands.22
MR Sialography
Magnetic resonance scintigraphy can be carried out to analyze radiation-induced xerostomia. This is a noninvasive three-dimensional imaging technique in which the saliva itself is displayed as “contrast agonist” along the full trajectory of the major salivary ducts. from the intraglandular part to the oral cavity. This method has been shown to depict radiation-induced changes in the salivary glands and ducts after radiotherapy.23 In a small group of nine patients, a visibility score for the ducts correlated with the mean dose to the parotid and submandibular glands. These results were confirmed in a study by Wada et al. MR sialography allowed visualization of the secretion response of the submandibular gland and parotid gland following stimulation. Radiotherapy in the submandibular gland had the strongest influence on the secretion response, resulting in insufficient secretion as demonstrated by MR sialography.24
MR sialography may therefore be a promising method. However, the reported series are very small and more experience is needed before the real value of this new technique can be assessed.
Diffusion-Weighted MRI Evaluation of Salivary Gland Function
This is also a noninvasive technique. Diffusion-weighted MRI displays the diffusion of molecules. With this technique, differences in water mobility generates differences in the image contrast in a T2-weighted sequence. A second gradient is used. The movement of water molecules results in dephasing, resulting in a signal loss on diffusion-weighted images. The loss of this signal due to the movement of water molecules correlates with the strength of the gradients used. When different gradients are used, an apparent diffusion coefficient (ADC) can be quantified. In a small study of eight patients, the preradiotherapy ADC values at rest were significantly lower for the parotid glands than for the submandibular glands.25 In nonspared salivary glands, the baseline ADC at rest was significantly higher after radiotherapy than before.25 The value of this method has yet to be tested in larger studies. In these studies, it was possible to combine MR sialography with diffusion-weighted MRI.
Quality of Life Questionnaires
The European Organization for Research and Treatment of Cancer Quality of Life Questionnaire on the Head and Neck (EORTC QLQ–H&N) includes two questions about xerostomia—one about xerostomia itself and one about sticky saliva (for further details on the questionnaires available, see Chapter 41). However, only two simple questions may not reflect the many aspects of xerostomia.15 More extensive questionnaires therefore need to be developed to inquire about the relationship of xerostomia to chewing, swallowing, speaking, and sleep and the rate at which patients need to sip water while eating dry food or at rest.15
In the LENT–SOMA scoring system (Late Effects in Normal Tissues—Subjective, Objective, Management, and Analytic), objective findings are incorporated in addition to a subjective grading by patients and grading by a physician. There may be a poor correlation between observer-based toxicity and the patient-reported degree of xerostomia. As found in a prospective study by Kam et al., IMRT for nasopharyngeal cancer significantly increased the salivary flow and significantly improved the observer-based toxicity, but no significant improvement in the patient-reported degree of dry mouth was seen.26 The study used questionnaires with a lack of detailed information. For example, xerostomia symptoms in daytime may differ from at those occurring at night.27 Particularly during the night, at rest, saliva production depends on the submandibular gland.15 Sparing one or both parotid glands may still not be sufficient to prevent nocturnal xerostomia symptoms. More detailed questionnaires therefore need to be used in studies on xerostomia symptoms.
Dose–Response Curves
The Parotid Gland
Research on xerostomia and the dose–response curve of the salivary glands has mainly focused on the parotid glands. One of the first detailed studies was by Eisbruch et al. at Ann Arbor University. In 1999, they published results for 88 patients treated with conformal radiotherapy techniques with the aim of sparing the contralateral parotid gland. They used objective measurements of parotid flow using Lashley cups, and used the Lyman model to analyze the relationship between the mean dose to the parotid gland and a salivary flow of less than 25% compared with the preradiotherapy level. One year after radiotherapy, there was a steep dose–response curve with a threshold of 26 Gy.5
In 2001, Roesink et al. published the results of a study in Utrecht including 108 patients treated with conventional radiotherapy, also using objective measurements of parotid flow. They found a less steep dose–response curve, with a median toxic dose (TD50; 50% complications seen for a certain dose) of 28 Gy after 6 weeks and 39 Gy after 1 year.4
Updated results from both institutes were combined in 2010, allowing analysis of the largest series so far published in the literature.28 A total of 384 parotid glands were available for analysis 1 year after radiotherapy. The mean dose to the parotid gland leading to a 50% complication probability in this analysis was around 40 Gy. The results were comparable between the two institutes (Fig. 42.2). The authors concluded that in attempts to preserve parotid gland function after radiotherapy for head and neck tumors, a gradual increase in the probability of complications is seen with an increasing mean dose to the parotid gland. A treatment planning constraint of 25–30 Gy corresponds to a 17–26% complication probability at 1 year. At a mean parotid dose of 40 Gy, there is a 50% probability of a reduction in parotid gland flow to less than 25% of the preradiotherapy flow rate.28 For a much lower probability, one should aim for a planning constraint on the mean dose to the parotid gland of less than 25 Gy.
Recovery of salivary gland function is observed during the first year.4,29 A long-term study by Braam et al. showed that continuing recovery of the parotid flow can still be seen even 1–5 years after radiotherapy.30
One of the possible explanations for the differences observed between the initial results the Ann Arbor and Utrecht studies is that they were due to different radiotherapy techniques being used; IMRT was used in Ann Arbor and conventional techniques were used in Utrecht. Different techniques may spare different parts of the parotid gland. In a subsequent prospective study in Utrecht, the results for a large number of patients treated with conventional radiotherapy or with IMRT were analyzed. With IMRT, the dosage to the medial part of the parotid gland is significantly higher in comparison with that to the lateral part of the parotid (Fig. 42.3). The authors found that 6 weeks and 6 months after radiotherapy, parotid glands in patients treated with IMRT showed a higher risk of flow reduction for the same mean parotid gland dosage in comparison with patients treated with conventional radiotherapy.31 However, the dose–response curves were equivalent after 1 year, independently of the treatment administered. The results after 6 weeks and 6 months may be related to the high dose to the medial lobe of the parotid glands in IMRT, in comparison with the high dose to the cranial part of the parotid gland that occurs with conventional radiotherapy techniques. A relatively high dose to the medial lobe of the parotid gland with IMRT may result in more damage to ducts and blood vessels. After 1 year, however, no regional dose-dependent damage to the parotid gland was found. Early after radiotherapy, therefore, the Lyman model based on the mean dose failed to fully describe the effect of radiotherapy on the parotid glands, although no difference between IMRT and conventional radiotherapy with regard to the dose–response curve was observed 1 year after radiotherapy.31
Fig. 42.2 The normal probability curve for tissue complications (< 25% flow) in the parotid glands: combined results from the Utrecht and Michigan studies. (Reproduced with permission from Elsevier, Inc., from Dijkema et al., International Journal of Radiation Oncology, Biology, Physics 2010;78: 449–453.28)
Fig. 42.3 Intensity-modulated radiotherapy for a pharyngeal tumor. the parotid glands are outlined in black and the tumor target volumes in white. High-dose areas are shown in red and low-dose ones in green/blue. A higher dose is delivered to the medial part of the parotid gland.
The Submandibular Gland
Very few reports on dose–response curves for the submandibular glands have been published. The submandibular glands appear to be less radiosensitive.32 A threshold has been shown for a submandibular mean dose of 39 Gy.33 The mean dose to the submandibular gland is related to patient-rated sticky saliva symptoms.34 The pathogenesis of xerostomia is apparently quite complex and may depend not only on the parotid glands, but also on damage to the submandibular glands.34 Sparing of a single submandibular gland by surgically transferring it to the submental space before radiotherapy can successfully prevent xerostomia—confirming the important role played by the submandibular glands.35 However, this surgical technique has several drawbacks and has not been widely applied.6
It is as yet unclear how submandibular gland sparing should be incorporated into predictive salivary function models.36,37
The Minor Salivary Glands
A correlation between xerostomia symptoms and the radiation dose to the salivary glands in the oral cavity, pharynx, and larynx and is not well established.
In conclusion, to prevent severe xerostomia at least one parotid gland has to be spared to a mean dose of less than 20 Gy, or both glands to a mean dose of less than 25 Gy.36 In general, however, the mean dose for the parotid glands should be as low as possible. For submandibular gland sparing, doses should be less than 35–40 Gy. The role of sparing of the minor salivary glands in the oral cavity is still unclear.36
Radiation Techniques for Sparing the Salivary Glands
Photon Therapy and Sparing of the Salivary Glands
Conventional Radiotherapy Techniques
In the head and neck region, normal structures such as the salivary glands, swallowing muscles, and spinal cord are generally in close proximity to tumor growth. In the past, conventional radiotherapy techniques were used to treat these patients. The definition of target volumes was generally based on bone and cartilage structures that are visible on radiography. Usually, opposing lateral fields were used for target coverage of the primary tumor and the neck nodes, combined with an anterior field for the supraclavicular nodes. A lateral field and oblique opposed lateral field were used for the boost to the primary tumor (Fig. 42.4). To boost the posterior region of the neck at the level of the spinal cord, electron beams were used at 40 Gy after shielding of the spinal cord. The clinical target volume includes the macroscopic tumor along with a margin for microscopic invasion, normally around 1 cm. An extra margin of around 0.5 cm is used for variation in the treatment set-up. Typical target doses are 46–50 Gy, five times a week, in fractions of 2 Gy to clinically negative neck nodes, and 70 Gy in 35 fractions of 2 Gy for definitive radiotherapy of the primary tumor and positive neck nodes. Sparing of critical structures such as the salivary glands and swallowing structures is not generally possible with this treatment technique.
Contrast-Enhanced Computed Tomography
A first step toward improvement of the technique described above was the use of contrast-enhanced computed tomography (CT), performed in the treatment position with the patient immobilized in the mask used for radiotherapy. The CT data are transferred to a planning system. It is also possible to carry out MRI or positron-emission tomography (PET) scanning with the same mask. The data are matched with the CT data and used to delineate the target volumes. MRI is preferable if there are dental artifacts, for delineation of the salivary glands, and for tumor target volume delineation in the nasopharynx and oropharynx. Fluorodeoxyglucose PET (FDG–PET) images are used to confirm or exclude borderline lymph nodes seen on CT. The radiation oncologist defines the gross target volume (GTV), the clinical target volume (CTV) in the elective lymph nodes, and the organs at risk—the spinal cord, brain, major salivary glands, and swallowing structures. These are delineated on each CT slice, and three-dimensional reconstructions are made. Delineation of the neck nodes on the CT scan follows internationally accepted guidelines.38 More conformal radiotherapy with three-dimensional radiotherapy planning can be designed on the basis of the target volumes and normal structures delineated.
Fig. 42.4 Parotid function after conventional radiotherapy (CRT) in comparison with intensity-modulated radiotherapy (IMRT). Dose distributions and percentage parotid flows are shown for the findings 6 weeks, 6 months, and 1 year after radiotherapy in comparison with before radiotherapy. (Reproduced with permission from Elsevier, Inc., from Braam et al., International Journal of Radiation Oncology, Biology, Physics 2006; 66: 975–980.40)
Intensity-Modulated Radiotherapy
In conformal radiotherapy, more beams are used instead of the parallel opposed beams in conventional radiotherapy. Three-dimensional conformal radiotherapy techniques provide better sparing of normal structures, but it is still not optimal. Intensity-modulated radiotherapy (IMRT) has now been available for over 10 years. With this technique, all of the target volumes and organs at risk are identified with CT, MRI, and/or PET. The required dose to the tumor is specified, as well as the maximum acceptable dose to the normal structures. In IMRT, five to seven non-opposing coplanar beams are usually combined around the tumor-bearing targets, with a steep dose gradient outside these regions. Several dose intensity levels, called segments, are used for each beam (Fig. 42.5). A multileaf collimator (MLC) in the head of the linear accelerator consists of individual leaves that are able to move independently, allowing different types of field configuration to be created. The mean number of segments may vary from 40 to 100.
In a step-and-shoot IMRT plan, the leaves move to the side position when the beam is off, but do not move when the beam is on. With dynamic IMRT, the leaves move continuously during radiation. In what is known as the tomotherapy system, a linear accelerator continuously rotates around a translating couch. In this way, helical IMRT is delivered, and a megavolt computed-tomography imaging facility is available. The megavolt CT images are acquired before each radiotherapy fraction and are used to reposition the patient before treatment, allowing smaller margins to be used for accurate treatment delivery.
The IMRT technique also makes it possible to use simultaneous moderated accelerated radiotherapy schedules. With this technique, the prescribed fraction dose to the GTV is 2.3 Gy—for example, 2.2 Gy to the clinical target volume around the GTV, and 1.8 Gy for elective radiation of the lymph nodes. This technique makes it possible to deliver a higher dose to the tumor without increasing the toxicity (Fig. 42.6).
Two randomized phase 3 trials comparing IMRT with conventional radiotherapy in head and neck cancer have so far been published. The first was a prospective randomized study in patients with early-stage nasopharyngeal carcinoma treated in China.26 Sixty patients were randomly assigned to either IMRT or two-dimensional radiotherapy. One year after treatment, patients treated with IMRT had a lower incidence of observer-rated xerostomia and a higher salivary output of stimulated parotid saliva in comparison with the conventionally treated group. However, there was only a trend toward improvement in patient-reported xerostomia. The authors concluded that sparing of other salivary glands in addition to the parotid is also necessary with IMRT.26 The first results of a phase 3 multicenter randomized controlled trial performed in the United Kingdom (the PARSPORT trial) were presented at the 2009 European Cancer Organization (ECCO) conference in Berlin. In patients with a T1–T4 N0–N3 M0 head and neck tumor, opposing lateral fields were compared with parotid-sparing IMRT.39 LENT–SOMA 1-year grade 2 or more xerostomia was reported in 64% of the patients with conventional radiotherapy, compared with 41% of those with IMRT (P = 0.006). After a long follow-up, improvement in salivary flow was seen in the IMRT group, but not in the conventional group.39 In a prospective nonrandomized trial in Utrecht including patients treated for oropharyngeal tumors, the mean dose to the parotid gland declined significantly from 48 Gy with conventional radiotherapy to 33 Gy with IMRT.40 There was also a significant improvement in parotid flow 6 months after radiotherapy, from 18% with conventional radiotherapy to 64% with IMRT.40 However, in this study as well, no significant differences between the treatments were observed with regard to subjective patient-rated xerostomia using the LENT–SOMA scales.
Fig. 42.5 a–c Fluence distribution in intensity-modulated radiotherapy with a multileaf collimator.
a The head of a linear accelerator with individual beams; the leaves enter from the edges.
b The beam arrangements.
c Individual beam dose intensity levels, based on approximately 10 different segments per beam.
Fig. 42.6 a, b
a A tumor in the tonsil, treated with seven-beam inverse-planned integrated boost intensity-modulated radiotherapy
b The fraction doses were 2.3 Gy to the gross target volume. (GTV), 2.2 Gy to the clinical target volume (CTV; 1 cm around the GTV), and 1.8 Gy to the elective nodes.
More detailed questionnaires were used in the same institute for patients treated with IMRT. Xerostomia symptoms at night and during the day were compared in the questionnaires. Sparing one or both parotid glands led to significantly improved results for xerostomia symptoms during the day, but the majority of patients still reported nocturnal xerostomia.27 At night, most saliva is produced by the submandibular gland. Sparing one submandibular gland is therefore essential. In a large series from the Free University of Amsterdam, IMRT was compared retrospectively with conventional radiotherapy. Xerostomia with an RTOG grade of 2 or more was significantly reduced with IMRT, and the same also applied to general quality of life questions relating to the head and neck.12
In a retrospective study, Münter et al. compared the influence of amifostine plus conventional radiotherapy with IMRT. IMRT was more effective than amifostine plus conventional radiotherapy for sparing the salivary glands. 99mTc pertechnetate was used in the study; the TD50 for conventional radiotherapy was 34 Gy and the TD50 for IMRT was 36.8 Gy.20
As mentioned above, sparing at least one submandibular gland may also be important for reducing xerostomia symptoms. A dose–response curve was reported in a study by Murdoch-Kinch et al.33 in which submandibular recovery over time was observed. The authors showed that with IMRT, in a planning study, it was possible to lower the submandibular dose by 12 Gy in five out of eight patients, resulting in a mean submandibular dose of less than 35 Gy. Keeping the mean dose to the contralateral submandibular gland to 40 Gy is a challenging goal. An advanced IMRT technique was used in a planning study in Utrecht including 10 patients with oropharyngeal cancer. Reducing the dose coverage to the contralateral elective node II level planning target volume to 90% and reducing the safety margin from 5 to 2 mm resulted in a reduction of toxicity in the contralateral submandibular gland to below 50%.41 Submandibular gland sparing was also found to be feasible in a study in Helsinki. One year after IMRT, the mean stimulated saliva flow in the submandibular gland was 60% of the baseline value in patients in whom one submandibular gland was spared and 25% in those in whom it was not spared. This resulted in less grade 2 and grade 3 xerostomia.32
Using IMRT significantly decreases the mean parotid gland dose. However, patient-reported xerostomia symptoms may be further improved by reducing the dosage to one of the submandibular glands.
< div class='tao-gold-member'>
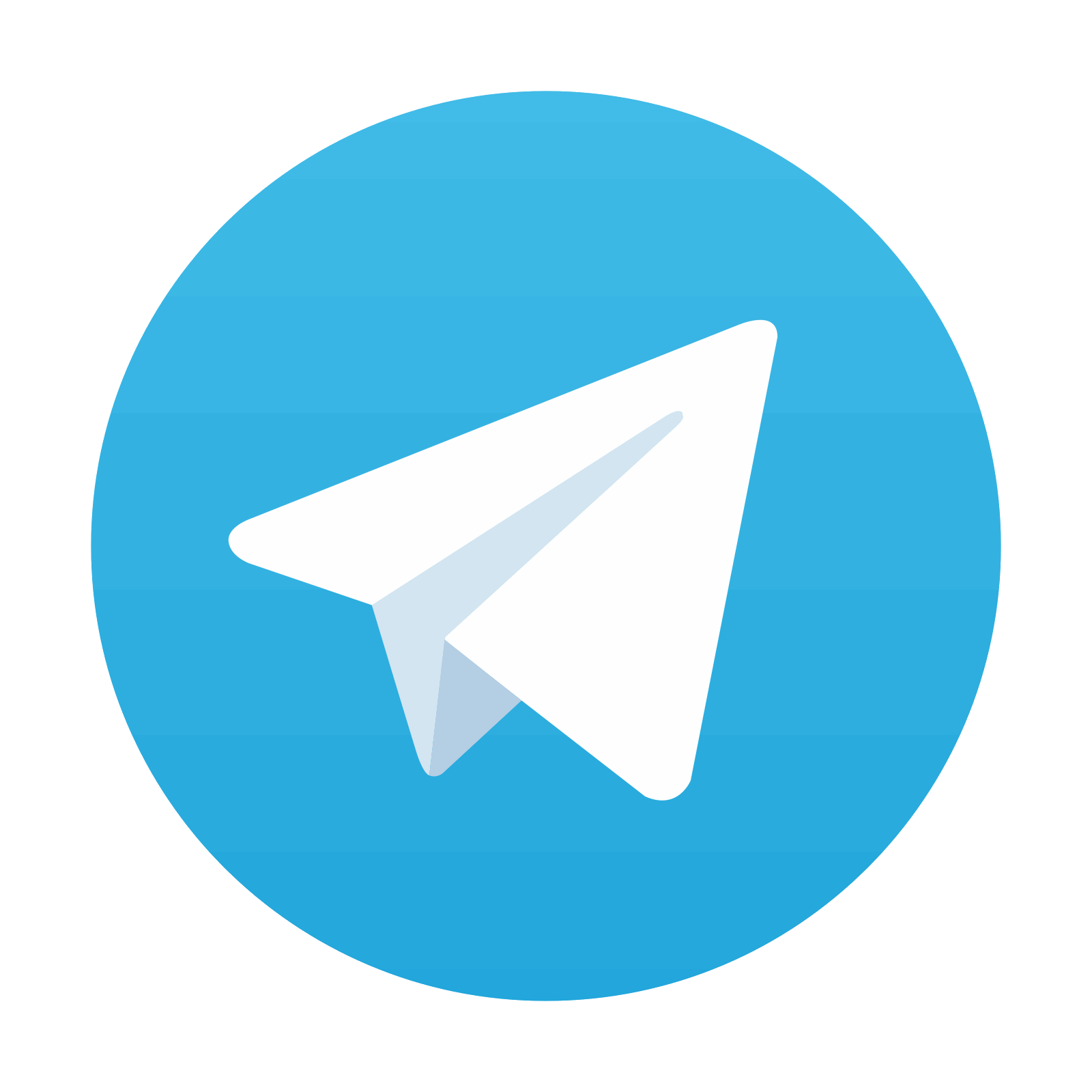
Stay updated, free dental videos. Join our Telegram channel

VIDEdental - Online dental courses
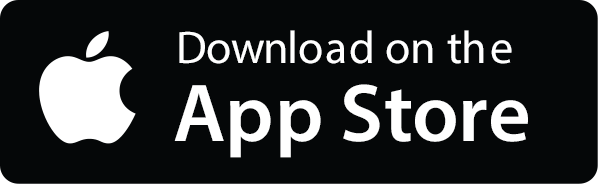
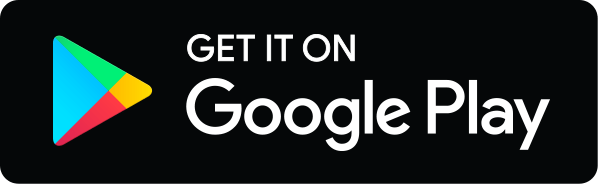