Graphical abstract

Highlights
- •
In vivo aging kinetics was assessed for biomedical grade 3Y-TZP ceramics.
- •
2-year exposure showed ∼3-times faster aging kinetics compared to in vitro extrapolation.
- •
Airborne-particle abrasion significantly suppresses in vivo aging.
- •
Surface degradation after 2-year in vivo was within clinically acceptable range.
Abstract
Objective
In vivo aging of biomedical grade 3Y-TZP ceramics in the oral environment was assessed and compared to artificially accelerated in vitro hydrothermal aging extrapolations at 37 °C.
Methods
88 discs were pressed and sintered (1450–1500 °C) from two commercial 3Y-TZP compositions containing 0.25% Al 2 O 3 to generate finer- and coarser-grained specimens. As-sintered (AS) and airborne-particle abraded (APA; 50 μm Al 2 O 3 ) surfaces were investigated. In vivo aging was performed by incorporating specimens in lingual flanges of complete dentures of 12 edentulous volunteers who wore them continuously for up to 24 months. For comparison, in vitro hydrothermal aging at 134 °C was also performed and analysed by XRD and (FIB)-SEM. Data was statistically analysed with linear regression models.
Results
Finer and coarser-grained specimens exhibited statistically insignificant differences in aging in vivo . The monoclinic fraction ( X m ) on AS surfaces abruptly increased to ∼8% after 6 months. The aging process then proceeded with slower linear kinetics (∼0.24%/month). After 24 months, X m reached ∼12%. The calculated maximum transformed layer was 0.385 μm representing one layer of transformed grains. APA surfaces were highly aging resistant. The initial X m of ∼4.0% linearly increased by 0.03%/month in vivo . In vitro aging exhibited an initial induction period, followed by linear aging kinetics. Coarser-grained AS surfaces aged significantly faster than fine-grained (2.41%/h compared to 2.16%/h). APA discs aged at a rate of 0.3%/h in vitro . Microcracking within a single grain and pull-out of grain clusters were observed on aged AS surfaces.
Significance
Biomedical grade 3Y-TZP was susceptible to in vivo aging. After 2 years in vivo , the aging kinetics were almost 3-times faster than the generally accepted in vitro-in vivo extrapolation.
1
Introduction
In the past three decades, there have been major advances in the application of 3 mol.% yttria partially stabilized zirconia polycrystalline (3Y-TZP) ceramics for dental restorations. This is due to their superior mechanical properties compared to other, especially glass-based dental ceramics . 3Y-TZP’s high strength and fracture toughness are governed by the stress-induced transformation ( t − m ) toughening mechanism, where under applied stress the metastable tetragonal phase transforms to monoclinic. This causes volumetric expansion and creates compressive stresses that oppose the tensile stress in the vicinity of a propagating crack tip . Transformation toughening has made 3Y-TZP an attractive alternative to traditionally used metals and led to its use for all-ceramic prosthodontic restorations, post-and-core systems and dental implants.
3Y-TZP’s tetragonal phase metastability also has a well-documented, deleterious aspect known as low-temperature degradation (LTD) or aging . In humid environments, the t − m transformation can be triggered spontaneously . This nucleation and growth process initiates from isolated surface grains and gradually proceeds into the bulk via a stress-corrosion-type mechanism . The growth rate appears to be linear without any retardation or limit . The transformed zone is a gradientless layer with an amount of residual non-transformed zirconia separated from unaffected material by a clear boundary . The accepted mechanistic theory states that the negatively charged water species annihilate the oxygen vacancies within the crystal lattice, destabilizing the t-ZrO 2 domains . The mechanism leading to the t-m transformation is thus temperature-dependent and diffusion-controlled, while the t-m phase transformation, once nucleated, is martensitic and is accompanied by extensive micro-cracking, which ultimately leads to strength degradation .
At the onset of the present millennium, several hundreds of 3Y-TZP femoral heads failed in a short period following implantation, with the origin of the fracture indirectly associated with LTD . Since then, extensive studies have been conducted to investigate the aging mechanisms and kinetics, resulting in a general definition of the conditions when LTD is prone to occur. Hydrothermally-induced transformation of 3Y-TZP ceramics is known to be a grain-size dependent process , which can be further influenced by composition, chemical and microstructural inhomogeneities , materials background and treatment ( i.e. surface mechanical/thermal/chemical modifications) and/or testing conditions . The role of the aqueous medium is not entirely clear, but will likely influence the transformation rate . The same holds for the influence of mechanical surface treatment.
Aging kinetics of 3Y-TZP ceramics were commonly investigated using accelerated hydrothermal protocols in autoclaves. According to Deville and Chevalier , the relationship between the amount of transformed monoclinic phase and the aging time at various temperatures can be used as guidance to estimate the equivalent aging time under in vivo conditions. Based on results obtained from failed zirconia femoral heads, these authors have calculated that 8 h of accelerated aging in an autoclave at 134 °C in distilled water, should result in ∼35% of the monoclinic fraction and correspond to approximately 32 years in vivo for this particular material and application. However, this generally accepted in vitro-in vivo extrapolation has been shown to be a major underestimation of the aging process .
Previous investigations by Keuper et al. who studied LTD of 3Y-TZP at 37 °C and normal pressure conditions showed that ∼35% of the transformed surface layer (∼0.5 μm per year) was obtained after only 4 years, which is 8-times faster than the above mentioned in vitro-in vivo extrapolation. Accelerated aging also suffers from known reproducibility complications when using different autoclaves, as water vapour pressure is a decisive factor for the transformation rate . Therefore, studying in vivo aging behavior in the oral environment is mandatory to better understand its real-time behavior and severity. Literature, however, remains remarkably scarce on this subject. A short, intra-oral aging study by Miragaya et al. reported that 60 days of aging already resulted in the evolution of 4.7–7.7 wt.% of m-ZrO 2 and substantially affected flexural strength, Young’s modulus, Vickers hardness and indentation toughness .
Our research group approached the problem by using complete dentures as vehicles to hold the zirconia ceramic specimens in patients’ mouths during normal function. In this manner the aging processes could be studied at body temperature in the aggressive environment of the oral cavity. Flat specimens allowed for easy removal and good patient compliance, which enabled us to conduct thorough microstructural and crystallographic analyses over the course of several years. The results were extensive and we decided to report them in two co-published articles, this being the first of the two . This study was designed to monitor the propagation of the t-m transformation of two biomedical grade, classic zirconia ceramics containing 0.25 wt.% of alumina differing in their mean grain size and transformability. The aim was to assess the extent of in vivo aging behavior in the oral environment, compare it to in vitro hydrothermal aging and assess their relationship with the established aging extrapolations at 37 °C .
2
Material and methods
2.1
Specimen preparation
Two high-purity “bio-medical-grade” powders TZ-3YB-E and TZ-3YSB-E, from Tosoh, Japan, were used to produce disc-shaped specimens (7.8 mm in diameter and 0.8 mm thick), which were labeled as B-E and SB-E, respectively. The discs were formed by uni-axial dry-pressing at 150 MPa. Due to their higher surface area the finer B-E pressed compacts were sintered at 1450 °C resulting in a nearly theoretical density (>99% TD), whereas a higher sintering temperature of 1500 °C was needed to reach a similarly high relative density of the coarser SB-E pressed compacts. After sintering, specimens from each material were divided into four groups of ten. Two groups of each material were left as-sintered (AS) and two groups were sandblasted or airborne-particle abraded (APA). The FEG-SEM micrographs illustrate the characteristic topography of the AS surfaces, whereas the grooves mimic those produced with the CAD/CAM technique ( Fig. 1 a) . After sintering, the AS B-E and SB-E specimens exhibited mean grain sizes of 0.59 μm and 0.51 μm, respectively ( Fig. 1 b and c). Airborne particle abrasion was performed at a distance of 30 mm for 15 seconds with 50-μm (diameter, Ø ) fused alumina particles at 2.5 bar, since this is a preferential method to clean the cementation surface and to increase surface roughness ( Fig. 1 d) for improved bonding with luting cements [49].

2.2
In vivo aging protocol
The in vivo aging experiment was based on the participation of 12 healthy volunteers (9 men and 3 women) willing to have the ceramic specimens incorporated into their complete dentures. The participants were recruited from the pool of edentulous patients treated with complete dentures at the Department of Prosthodontics, Faculty of Medicine, University of Ljubljana. The following criteria were used to select the patients: good rating of the existing dentures based on the visual scale questionnaire, dentures worn 24 h/day, no head and neck radiotherapy. Patients received verbal and written information on the study design and gave signed consent to participate.
The study was approved by the National Medical Ethic Committee of the Republic of Slovenia. (approval no. 61/04/1011). All the procedures were in accordance with the 1975 Declaration of Helsinki, as revised in 1983. Disc-shaped cavities were prepared in the lower complete denture of each participant with a tungsten-carbide bur (SH 72E, Shofu, Japan). Two fine-grained and two coarse-grained discs were implanted in the lingual flange of the lower denture with the abraded or as-sintered surface exposed to the oral environment. Cold curing acrylic resin (Ivoclar, Liechtenstein) was used to fix the 3Y-TZP specimens in the dentures ( Fig. 1 f–h). The retention and stability of dentures were checked and patients reported no discomfort at the insertion of this newly constructed intraoral device.
Volunteers were instructed to remove the dentures for cleaning twice a day using a soft toothbrush and soapy water. They were also instructed to wear the dentures continuously for 24 h/day and not to use any additional mouthwash or denture cleaner for the duration of the study. After 6 months the discs were explanted, gently cleaned by ultrasonication in sodium hypoclorite to remove the adhered proteins and subjected to XRD and FEG-SEM surface analyses. The visits were part of a regular recall program to check the function of the dentures. After analyses were complete, the specimens were re-implanted with cold-curing acrylic resin for the next 6-month period. The same protocol was followed until the end of the study at 24 months.
For comparison, specimens of each material and surface treatments were exposed to accelerated artificial aging by autoclaving in an artificial saliva under isothermal conditions at 134 °C for 10 h.
2.3
In vitro aging protocol
Accelerated in vitro aging was performed by autoclaving groups of 5 specimens in artificial saliva under isothermal conditions at 134 °C for up to 10 h.
2.4
Specimen characterization
The microstructural observations were made by field emission scanning electron microscope (FE-SEM Carl Zeiss, Supra 35LV, Oberkochen, Germany) using accelerated voltage of 1 kV. Grain size evaluations were made on polished (3 μm diamond paste) and thermally etched (1380 °C, 1 h), uncoated specimens, using the linear intercept method, based on the ASTM E112-13 standard without any correction factors used .
X-ray diffraction patterns (XRD) were collected from all the specimens from each group with X’Pert PRO X-Ray diffractometer equipped with a PIXcel detector using Cu-Kα radiation at 45 kV and 40 mA over the range of 25°–40° 2 θ (PANalytical, Almelo, The Netherlands). The relative amount of transformed monoclinic zirconia ( m -ZrO 2 ) on the specimens’ surfaces was determined from the integrated intensities of the monoclinic (1 ̅11) m and (111) m , and the tetragonal (101) t peaks according to the method of Garvie and Nicholso , which can be applied to determine the phase composition of zirconia with randomly distributed m -ZrO 2 and t -ZrO 2 phases at any distance from the surface exposed to XRD. To generate additional information related to aging kinetics, the thickness of the propagating transformed zone depth (TZD) layer was calculated in μm using the XRD method developed by Kosmač et al. for ceramics containing tetragonal ZrO 2 .
For the FIB-SEM analysis (FEI Helios Nanolab 650) the regular cross-sections were made using ion-beam machining and were finalized by ion polishing. Prior to machining and polishing, a 0.5 μm layer of platinum film was sputtered on the area of interest, using the ion-beam-assisted gas injection system at 30 kV and 0.43 nA to prevent the extensive curtain effect. As-prepared cross-sections were observed in situ , at an angle of 52°, using the electron probe at 2 kV and 100 pA.
2.5
Statistical analysis
Data on the monoclinic content was statistically analyzed with linear models. Tukey’s HSD test was used to examine the pairwise differences between treatment groups. The statistics software package R 3.1.2 was used for the analyses . The significance level was set to α = 0.05.
3
Results
The participants displayed good adherence to the study setup and reported no issues with wearing the experimental dentures. One participant withdrew due to ill health after 6 months, one was not able to continue participating after 12 months due to relocation and one was lost to follow-up after 18 months. Other participants were able to attend the visits as planned until the end of the study.
3.1
Diffractograms of the exposed specimen surfaces
According to the results of the XRD analysis, AS SB-E and B-E materials initially consisted of monoclinic-free t -ZrO 2 ( Fig. 2 a) . In addition, a significant amount of t ’-ZrO 2 (usually referred to simply as the cubic phase) was also present, as indicative by the small double peak positioned at 35° 2 θ in between the (002) t and (110) t peaks of t-ZrO 2 . This phase can represent more than 21% in the 2-h-long sintering regimen at 1450–1500 °C .

After 6 months in vivo , XRD diffractogram of AS surfaces developed noticeable monoclinic peaks ( m -ZrO 2 ) at 28.15°, 31.3° and 34°. They corresponded to (1 ̅11) m , (111) m and (002) m and indicated the initiation of the aging process ( Fig. 2 a). With longer aging times of 12 and 24 months, the intensity of the monoclinic peaks slightly increased, but not substantially. APA, on the other hand, resulted in more complex diffractograms commonly seen in mechanically damaged 3Y-TZP surfaces ( Fig. 2 b) . These diffractograms exhibited much lower peak intensities due to uneven surfaces. In addition to the initial occurrence of the monoclinic phase ( X m ) of about 4%, they also exhibited a low-2 θ -angle broadening of the main tetragonal peak (101) t , a reversed intensity of tetragonal doublet peaks (002) t and (110) t , at 35° 2 θ , and an increased full width at half maximum (FWHM) of the (002) t peak as compared to the (110) t counterpart.
The diffractograms of AS specimens subjected to in vitro aging in Fig. 2 c and d appear similar to the in vivo counterparts ( Fig. 2 a and b), but the dynamics of t − m transformation were different. In AS SB-E specimens, there was only a slight emergence of the (1 ̅11) m monoclinic peak after 2 h, suggesting the existence of an induction period, an initial stage of slower aging kinetics. After 6 and 10 h in vitro , the intensities the m -ZrO 2 peaks already exceeded those encountered after 24 months in vivo ( Fig. 2 a).
APA specimens exhibited a visible initial increase of the (1 ̅11) m peak immediately after 2 h in vitro . However, this remained practically unchanged for the subsequent 8 h of aging. An interesting observation was that the (111) m peak did not seem to increase at all. This is indicative of the preferred orientation of the formed monoclinic domains after APA. It was shown that when a large shear component is present, it causes a stress-induced preferred orientation of both tetragonal and monoclinic variants .
3.2
Analysis of the monoclinic fraction ( X m ) and transformed zone depth (TZD)
The mean values for the transformed monoclinic fraction ( X m ) with their respective standard deviations are summarized in Table 1 ( in vivo aging) and Table 2 ( in vitro aging).
Aging in vivo (months) | Material | Surface treatment | X m in % (SD) |
---|---|---|---|
0 | BE | As sintered | 0.0 i (0.00) |
0 | SBE | As sintered | 0.0 i (0.00) |
0 | BE | APA | 4.1 gh (0.42) |
0 | SBE | APA | 3.8 h (0.36) |
6 | BE | As sintered | 7.3 e (0.61) |
6 | SBE | As sintered | 8.5 de (1.40) |
6 | BE | APA | 6.0 f (0.41) |
6 | SBE | APA | 5.7 f (0.57) |
12 | BE | As sintered | 9.4 cd (0.70) |
12 | SBE | As sintered | 10.0 bc (1.55) |
12 | BE | APA | 5.9 f (0.54) |
12 | SBE | APA | 5.5 f (0.63) |
18 | BE | APA | 5.7 f (0.91) |
18 | SBE | APA | 5.8 f (1.06) |
24 | BE | As sintered | 11.5 ab (0.67) |
24 | SBE | As sintered | 12.9 a (0.76) |
24 | BE | APA | 5.4 fg (1.53) |
24 | SBE | APA | 5.7 f (1.71) |
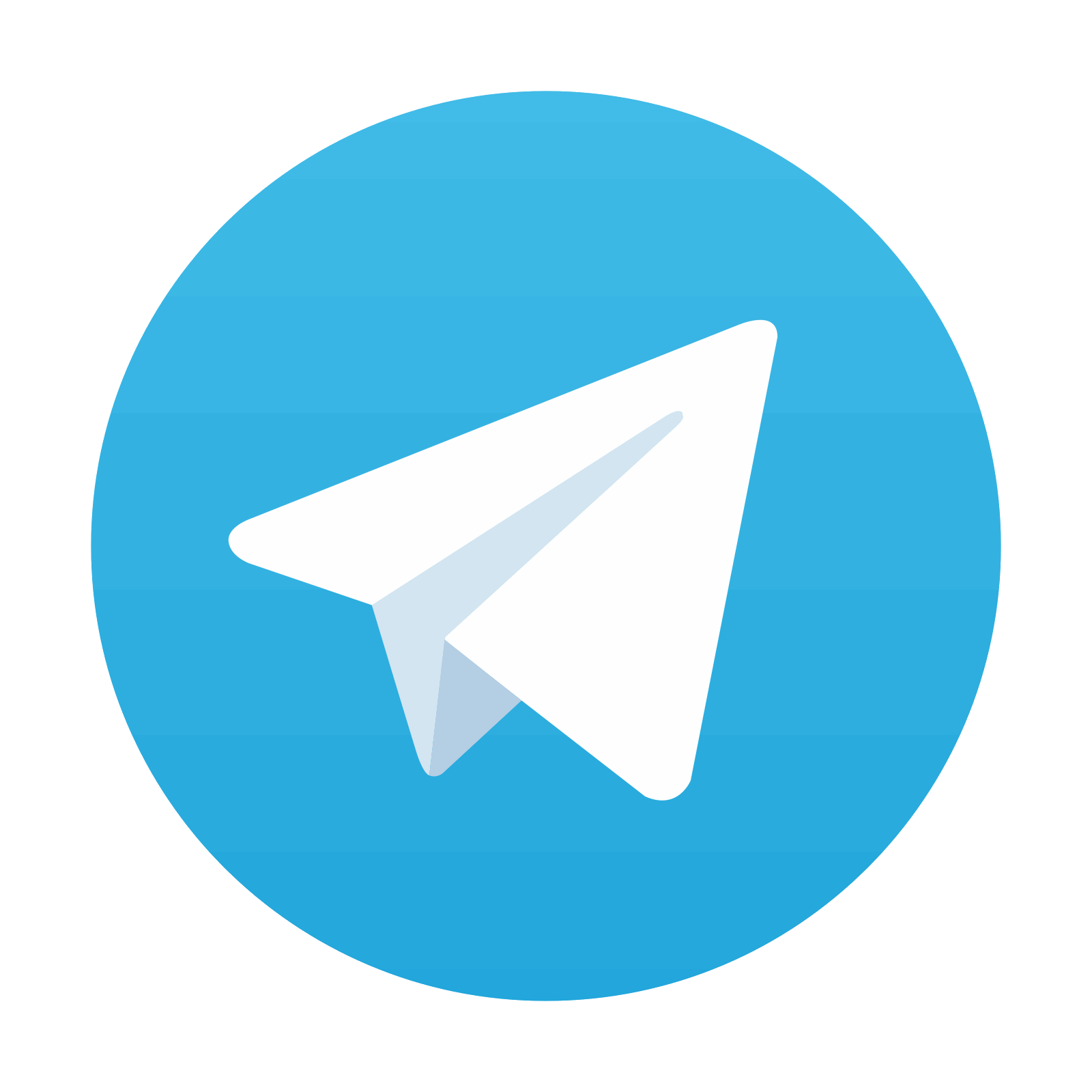
Stay updated, free dental videos. Join our Telegram channel

VIDEdental - Online dental courses
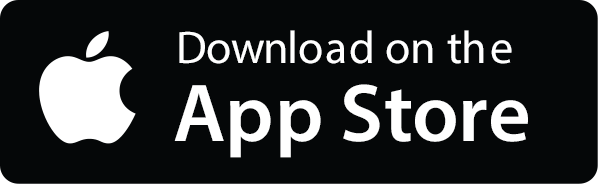
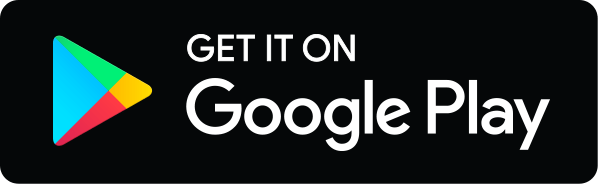
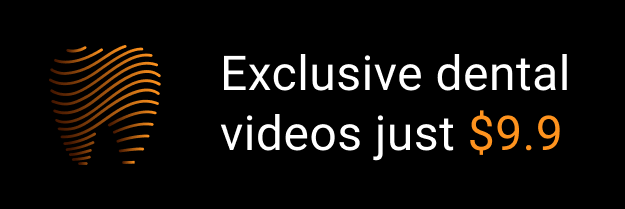