Introduction
In this in-vitro study, we evaluated the temperature changes in the pulp chamber during bracket bonding using 4 different light sources.
Methods
Eighty intact extracted maxillary central incisors were used. The teeth were divided into 4 groups of 20 teeth each. Brackets (Mini Twin, Dentaurum, Ispringen, Germany) were bonded with Transbond XT (3M Unitek, Monrovia, Calif) adhesive and light cured with low-intensity halogen light for 40 seconds, high-intensity halogen light for 40 seconds, light-emitting diode (LED) light for 20 seconds, and plasma arc light (PAC) for 6 seconds. Light curing was performed 5 mm from tooth surfaces. A J-type thermocouple wire was positioned in the center of the pulp chamber. The results were analyzed with analysis of variance (ANOVA) and the Tukey HSD test.
Results
ANOVA and the Tukey HSD test showed that pulp chamber temperature changes were influenced by the type of light source. All groups showed significant differences between each other ( P <0.001). The intrapulpal temperature changes induced by different light sources were the following: high-intensity halogen (6.84°C ± 2.44°C), low-intensity halogen (4.71°C ± 0.96°C), LED (2.95°C ± 1.12°C), and PAC (0.96°C ± 0.83°C).
Conclusions
High- and low-intensity halogen light induced significantly higher intrapulpal temperature changes than did the LED and PAC. Except for the high intensity halogen light, orthodontic bonding with light-curing units did not exceed the critical 5.5°C rise in temperature reported to produce pulpal damage.
The desire to cure on demand has caused more orthodontic practitioners to use light-cured adhesives instead of the more traditional paste-paste adhesives requiring in-office mixing. The light-initiated resins now have become the most popular adhesives for most orthodontists. Photo-activated resin composites are the choice of adhesive for orthodontic bonding because of their ease of use and the extended time they allow for bracket placement. These resins offer the advantage of extended, though not indefinite, working time. During the 1990s, the bond strength of dual-cured and light-cured adhesives to transparent ceramic brackets and metallic brackets was studied. Most studies concluded that the light-cured material was not different from that for chemically cured adhesives, and even these materials were found to have higher bond strengths.
A fascinating improvement in orthodontic bonding was the introduction of powerful light sources, which greatly reduce the irradiation time of adhesives for the initiation of polymerization. High-intensity, low-intensity, light-emitting diode (LED), argon-laser, and plasma-arc light curing units have become commercially available in recent years. Zach and Cohen, using primate teeth, showed that, with their chosen experimental technique, a rise of 5.5°C in pulp caused considerable damage, resulting in complete loss of 15% of pulp vitality. They also reported that an increase of 11°C or more invariably resulted in necrosis. Meredith et al pointed out that the situation in vivo, when there is movement of blood or other fluids with the potential for heat dissipation, is different from in-vitro experiments with extracted teeth. Even a small rise in pulpal temperature, irrespective of its cause, seems to produce histologic evidence of pulpitis of varying severity in animals. In-vitro experiments can, however, also yield results that should alert clinicians to a potential hazard to the health of pulp.
The most widely used light source for photo-activating resin-based composite is quartz-tungsten-halogen (QTH) lights. QTH lights with higher light intensities (HQTH) have significant potential for use in dentistry. LEDs have narrow spectral ranges and are therefore highly efficient light sources. The plasma-arc curing (PAC) light is also designed for high-speed curing of composite filling materials in direct resin restorations.
However, some questions about the effects of these curing lights have been raised. In the curing session, these lights can cause the temperature to rise in pulp, and this can be harmful to the vitality of the tooth. In this in-vitro study, we evaluated the temperature changes in the pulp chamber during bracket bonding using 4 light sources: QTH, HQTH, LED, and PAC.
Material and methods
Eighty intact maxillary central incisors extracted from adults were used. Teeth of homogeneous size and shape were used to provide similar thicknesses of hard tooth structure and ensure similar distances from the pulp to the surface of the tooth. Pulp extensions were evaluated with radiographic films by using calibrated calipers. Periapical radiographs for all teeth were made with the same x-ray unit (Siemens, 60 kV, 10 mA, Munich, Germany) with a 5-cm fixed focal image distance. The radiographs were processed automatically with Periomat (Dürr Dental, GmbH & Co, Bietigheim-Bissingen, Germany). After radiographic evaluation, teeth with abnormally large or small pulp chambers were excluded from the study.
The maxillary central incisors were divided into 4 groups of 20 teeth each. The root portions were sectioned with carborundum disks (Komet, Gebr Brasseler, Lemgo, Germany) approximately 2 mm below the cementoenamel junction perpendicular to the long axis of the teeth. The opening into the pulp chamber was enlarged as needed to insert the thermocouple wire. The pulp chamber was cleaned of remaining pulpal tissues with a spoon excavator and sodium hypochlorite application for 1 minute. Then the pulp chambers of the teeth were rinsed with distilled water and air dried.
The teeth were etched for 30 seconds with 37% orthophosphoric acid (3M Dental Products, St Paul, Minn), rinsed with water from a 3-in-1 syringe for 30 seconds, and dried with an oil-free source for 20 seconds. Metallic orthodontic brackets (Dentaurum, Ultraminitrim, Ispringen, Germany) were bonded by using Transbond XT (3M Unitek, Monrovia, Calif) adhesive, excess composite was gently removed with a scaler before curing, and light cured with 4 light units ( Table I ). The light curing was done as follows: group 1, QTH (Hilux, Benlioğlu Dental, Ankara, Turkey) for 40 seconds; group 2, HQTH (Optilux 501, Kerr, Danbury, Conn) for 40 seconds; group 3, LED (Elipar Freelight, 3M ESPE, St Paul, Minn) light for 20 seconds; and group 4, PAC (Power PAC Plasma Curing, ADT, San Carlos, Calif) for 6 seconds.
Manufacturer | Diameter of tip (mm) | Power intensity (mW/cm 2 ) | Exposure time (s) | |
---|---|---|---|---|
Hilux | Benlioğlu Dental, Ankara, Turkey | 10 | 500 | 40 |
Optilux 501 | Kerr, Danbury, Conn | 8 | 850 | 40 |
Elipar Freelight | 3M ESPE, St Paul, Minn | 8 | 400 | 20 |
Power PAC Plasma Curing | ADT, San Carlos, Calif | 6.5 | 1200-1500 | 6 |
The outputs of the light tips from the Hilux, Optilux 501, and LED curing units were measured by using a digital curing radiometer (Demetron, Danbury, Conn). Output of the PAC system, which could not be measured with the cure radiometer, was 1200 to 1500 mW per square centimeter according to the manufacturer ( Table I ).
A J-type thermocouple wire of 0.36-in diameter (Omega Engineering, Stamford, Conn) was connected to a data logger (XR440-M Pocket Logger, Pace Scientific, Mooresville, NC) during the light-curing procedures. All light-curing procedures and measurements were performed by the same operator (T.U.) to ensure uniformity in the procedures. A silicone heat-transfer compound (Philips ECG, Waltham, Mass) was injected into the pulp chamber to facilitate the transfer of heat from the walls of the pulp chamber to the thermocouple. The thermocouple wire was put into the pulp chamber by touching the center region of the roof. Before temperature measurements were made, the position of the thermocouple was verified by using radiographs and corrected as needed. Calibration of the data logger was not required. Specification accuracy was maintained without user adjustments. The manufacturer reported a temperature accuracy of ± 0.15°C from 0°C to 40°C. The collected data were monitored in real time and transferred to a computer. The data were available in both tabular and graphic forms.
After the temperature measurements, the teeth were vertically sectioned in a mesiodistal direction by using a slow-speed diamond saw (Isomet, Buehler, Lake Bluff, Ill) under running water. With a calibrated caliper, the enamel and dentin thickness from the middle of the roof of the pulp chamber to the end of the procedure was measured. Teeth with extremely thick or thin enamel or dentin were excluded from the study, and new specimens were prepared with the same procedures.
For each group, temperature variation was determined as the change from baseline temperature to the highest or lowest temperature recorded after the various light curing procedures. A positive temperature variation value indicated an increase in pulp temperature, whereas a negative temperature variation value indicated a decrease in pulp temperature. Twenty calculated temperature changes were averaged to determine the mean value in temperature rise. A temperature increase of 5.5°C was set as the baseline value, above which Zach and Cohen reported pulpal damage.
Statistical analysis
Descriptive statistics, including means, standard deviations, and minimum and maximum values, were calculated for each of the 4 groups of light-curing units. Analysis of variance (ANOVA) and then the Tukey honestly significant difference (HSD) tests (version 10.0, SPSS, Chicago, Ill) were used to analyze temperature changes between the groups at a significance level of P ≤0.05.
Results
The descriptive statistics for each experimental group are shown in Table II . ANOVA and the post-hoc tests showed that pulp-chamber temperature changes were influenced by the type of light source.
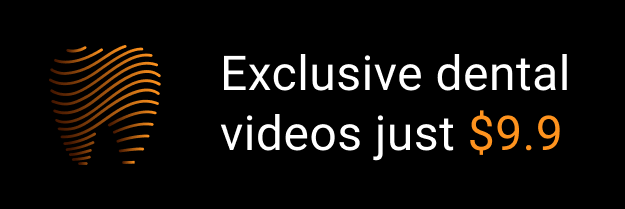