Fig. 6.1.
Spectral output of one quartz-tungsten-halogen source (Optilux 501) and two light-emitting diode sources (Freelight-1 and Ultralume-2)
Further Variables with Light Sources
Alternative curing routines using stepped, pulsed or ramped energy delivery have been developed with the intent of improving restoration interfacial integrity by reducing composite curing rate, thereby increasing its flow capacity. A slow polymerisation of a resin composite may give rise to reduced polymerisation shrinkage in restorations because of stress relief. This may be achieved by applying short pulses of light energy (pulsed delay), pre-polymerisation at low light intensity followed by final exposure at high intensity (soft start), or a combination of both (ramp curing).
In pulse-delay cure the polymerisation is initiated by a short flash of light followed by a waiting time of several minutes before the final polymerisation is performed. It was shown that pulse activation and soft-start polymerisation regimens did not significantly reduce post-gel shrinkage [30], and that pulse-delay technique resulted in a polymer structure with lower crosslink density compared with slow-start polymerisation [31].
The soft start was found to reduce the speed of shrinkage while maintaining degree of cure [3, 32]. Other potential benefits of soft start include reduced polymerisation shrinkage and temperature rising [33], lower solubility and equivalent hardness values compared with a standard halogen source [34].
The ramped light intensity can slow the rate of cure and help to reduce the rate and maximum polymerisation stress without compromising the physical properties of the material [35].
Radiometry: Irradiance (Radiant Incidence and Radiant Exitance) and Total Energy Concept
Irradiance is a term that can legitimately be used for light output from a source and also for light input onto a defined surface area; thus, there are two possible conditions. The flux can be arriving at the surface (Fig. 6.2a), in which case the radiant flux density – or optical power received per unit area on the surface – may also be referred to uniquely as radiant incidence. The flux can arrive from any direction above the surface, as indicated by the rays. Irradiance in this case is defined as:

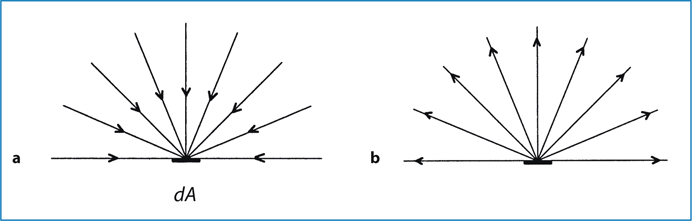
Fig. 6.2.
a Radiant incidence; b radiant exitance
where Φ is the radiant flux arriving at the point and dA is the differential area surrounding the point.
The flux can also be leaving the surface due to emission and/or reflection (Fig. 6.2b). The radiant flux density is then referred to as radiant exitance. The flux can leave in any direction above the surface. Irradiance in this case is defined as:

where Φ is the radiant flux leaving the point and dA is the differential area surrounding the point.
Since irradiance is equivalent to power (or energy/time) per unit area, then the integral of irradiance and time is equivalent to total energy per unit area. In cases where the irradiance is constant over a time interval, then the product of the irradiance and time gives the value of the total-energy integral. This parameter is often used when investigating the dependence of photo-polymerisation upon irradiation conditions.
Light Penetration into and Through Oral Biomaterials
Depth of Cure, and Top/Bottom Surface Hardness
A major characteristic of visible light-cured (VLC) composites is the variable depth of cure, which arises from attenuation of light transmission in these materials. The variation of cure with depth into composite filling materials has been studied by several techniques, including microhardness profiles [36, 37] and DC [38]. Several techniques have been compared [39, 40, 41].
Several parameters may be derived to characterise the depth of cure; these include the depth at which hardness falls to 80 % of its peak value [37] and the depth at which the hardness falls to the lowest measurable value [42]. A resin which is not fully polymerised will show reduced mechanical properties, poorer colour stability and greater susceptibility to stain.
With the development of light sources of improved irradiance, at least a 2- to 3-mm depth of material can be polymerised in 20-30 s irradiation. Irradiance falls within the body of the material because of absorption and scattering by the resin and filler particles, and by tooth substance.
Surface Reflection, Absorption and Scattering Processes in Composites
Aesthetic restorative materials should match the optical properties of hard dental tissues, especially in colour and translucency. The colour of the composites reflects the reflectance spectrum (i. e. light reflection) of the composites, which are considerably affected by the background colour and the thickness of the composites [43].
According to Fresnel’s relationship, for light incident to a normal plane boundary between media of refractive index n1 and n2, the reflectance [r] is:
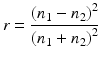
Hence, a composite is only translucent when there is matching of refractive indices between resin matrix and filler [44]. For methacrylate resins, the n is ~1.5. Adjustments in refractive index over a small range can be made by change in the ratio of primary and diluent monomers, such as Bis-GMA/TEGDMA. Of the glass fillers, n = 1.552 at the peak wavelength, ca. 470 nm, for a widely used barium glass. Under the same conditions, n = 1.506 for a strontium glass.
The theory of scattering is not well developed for particulate glass composite resins of the appropriate particle size ranges, volume fractions and refractive index increments (Δn). Rayleigh scattering describes the case where particles are small compared with wavelength, Rayleigh-Gans scattering applies to larger particles provided that An is small. The more general Mie theory is still not fully applicable and empirical relationships have been widely employed.
Kubelka and Munk (K-M) [45] mathematically expressed optical properties, such as light reflection and transmission of translucent light-scattering materials, in terms of simple hyperbolic functions of their thickness, dependent upon two optical constants such as absorption and scattering coefficients at each wavelength in the visible light spectrum. Because K-M’s theory could successfully predict reflection spectra and colour of translucent light-scattering materials of differing thickness on various backgrounds, several dental materials scientists and clinicians have applied this theory to the evaluation of optical properties, especially light reflection, of set dental aesthetic restorative materials [43, 46 – 49]. There are, however, few studies of optical properties, especially light transmission, of uncured VL-cured composites by K-M’s theory [48].
Composites may be provided in both opaque and translucent forms to match different areas of the dentition. Metallic pigments are added in small quantities to give different tints; these absorb rather than scatter light.
The optical properties of composites can change as a result of polymerisation. The extent of change is dependent upon the characteristics of the material and wavelength [50]. When VLC resins are cured, nresin and hence Δn change slightly. This can produce a significant change in light transmission and appearance of the composite. The translucency can increase or decrease, depending upon whether nresin > n glass’ or vice versa.
The camphoroquinone photo-sensitiser imparts a yellow tint to the monomers, which is lost as CQ is consumed. Residual amine-reducing agents may degrade in clinical service and cause discolouration of the composite.
Surface reflectivity and gloss depends strongly on the mean particle size. Polishable composites, which retain a gloss caused by specular reflection, are based on particle sizes ca. 0.5 µm. Colour matching of composites for use under artificial lighting, which may include UV components, is difficult.
Application of Beer-Lambert Law
Regarding the Beer-Lambert law, light is attenuated with increasing cross-sectional distance from the irradiated surface [51] as a result of light absorption and scattering caused by fillers and other additives [52], leading to limited depth of cure [37].
To express this mathematically, firstly, the relationships must be established between the light levels incident upon the surface and those available at depth within the specimen. This is given by the Lambert law:

where Io is the light intensity entering the specimen surface and I is the light intensity at depth d below the surface. Ɣ is the Naperian absorption coefficient of the medium. Changing to a decadic exponent, the transmittance τ,which expresses the available light intensity within the medium at depth d, is defined as:
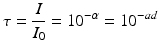
where ɑ is the absorbance and γ=2.303x a. The (decadic) linear absorption coefficient [a] includes the effects of absorption by all components of the resin-composite system, as well as scattering effects. (If the camphorquinone concentration is unnecessarily high, this will lead to attenuation of radiation through the material.)
Secondly, the light intensity absorbed by the photo-sensitiser across a thickness element δd, at depth d in the medium, is then:
![$${I_a} = I.[1 - {e^ - }^{{a_s}\delta d}] \approx I.{a_s}\delta d$$](/wp-content/uploads/1008/A978-3-540-28559-5_6_Chapter_TeX2GIF_Equ6.gif)
where as is the absorption coefficient of the photo-sensitiser. The value of asδd is given by Beer’s law:

where εs is the molar absorptivity (extinction coefficient) of the photo-sensitiser [concentration CS], at the particular frequency of radiation absorbed.
The overall expression for nonuniform monochromatic light absorption of the composite system, in terms of the number of quanta absorbed by the photo-sensitiser per unit volume at a distance d below the surface, may be given by:
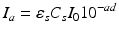
It may often suffice to assume that, at depth within the composite, the concentration CS of the photo-sensitiser is constant, where this is present in excess of the photon intensity.
Photo-Initiators for Free-Radical and Cationic Polymerisation
Most VLC dental composites are supplied as single-component formulations. Resin composites formulated as dual-component types undergo either self-cure upon mixing, or dual cure upon mixing and application of visible light, i.e. dualcure systems incorporate both self-cure and VLC activator/initiator chemicals. These are commonly, but improperly, denoted the “catalyst” system(s).
The organic monomer phase usually includes low concentrations of inhibitors and stabilisers together with the chemicals required for activation and initiation of monomer polymerisation or “cure”. The initiation process has a significant effect on the kinetics of polymerisation and the polymer structure; thus, it can affect several properties, such as rheology and long-term performance, of the network. The structure of radicals and their effect on the rate of reaction is critical to the development of an understanding of polymerisation [53].
Camphorquinone
The VLC resin-composite materials usually employ photo-sensitised free-radical initiators, commonly, an α-1,2 diketone, such as benzyl or camphorquinone (CQ; Fig. 6.3), and an amine-reducing agent such as dimethylaminoethyl methacrylate (DMAEMA) or dimethyl p-toluidine (DMPTI) [54, 55].
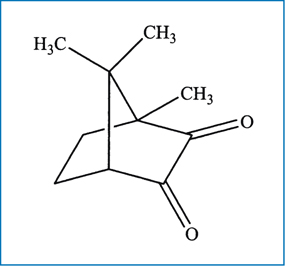
Fig. 6.3.
Chemical structure of camphoroquinone (CQ)
The CQ/amine photo-initiator system for generating radicals is widely used for the polymerisation of dental restorative materials. Light is absorbed by CQ in the blue region and leads to the n→π* transition of the dicarbonyl group [56]. The nonbonding electrons can be promoted to a π* antibonding orbital. This excited state has a half-life of approximately 0.05 ms. The excited n→π* transition interacts with an amine molecule and forms an exciplex, which is an excited short-lived state. Within this exciplex, CQ accepts an electron from amine and from the radical ion pair, and abstracts a hydrogen atom from the tertiary amine which results in the primary radical. This latter radical then attacks the carbon double bonds of the monomers. The former CQ radical may retard polymerisation through termination reactions [57].
The concentration of CQ photo-sensitiser is in the range 0.17-1.03 mass percent of the resin phase and that of DMAEMA reducing agent is 0.86-1.39 mass percent [55]. The combined photo-sensitiser/ reducing-agent complex has an extended absorption band within the VL spectrum.
1-Phenyl-1,2-propanedione
Camphorquinone is inherently yellow, which causes problems in colour matching. This, in turn, places practical limits on the concentration of CQ and, consequently, limits the degree of polymerisation and depth of cure that can be attained; therefore, alternative photo-sensitisers have been considered. Diacetyl (2,3-butanedione) and propanal have been studied by Peutzfeldt and Asmussen [58, 59]. A prominent initiator is 1-phenyl-1,2-propanedione (PPD) which has an aromatic group on one side of the dicarbonyl and a methyl group on the other (Fig. 6.4) and λmax≈410nm. Recent experiments showed that PPD would be an efficient visible light photo-sensitiser, comparable with camphorquinone, for the initiation of the dental resin polymerisation [60], and that resins initiated with PPD showed better mechanical properties than those initiated with CQ [61]. Furthermore, PPD can act synergistically with CQ to increase the monomer conversion to polymer and/or reduce the photo-sensitiser concentration (and, hence, colour). This blend of photo-sensitisers may produce a better balance between surface cure and bulk cure [62]. The combination will also contribute to a reduction in chroma (from deep yellow to a pale yellow) when the total photo-sensitiser (PPD + CQ) concentration is held constant since the PPD wavelength (λmax≈410nm) will shift the hue to a less yellow shade (shorter dominant wavelength). The absorption spectra of CQ + PPD is shown in Fig. 6.5.
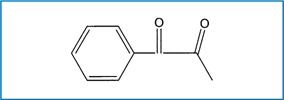
Fig. 6.4.
Chemical structure of 1 phenyl 1,2 propanedione (PPD)
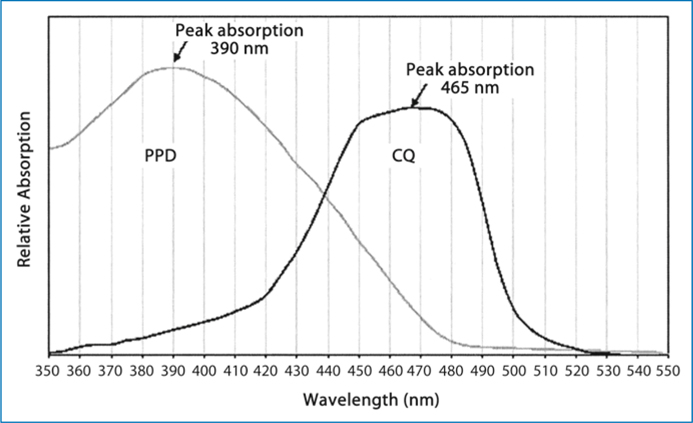
Fig. 6.5.
Absorption spectra of CQ + PPD
Photon Numbers and Photo-Initiation
It is useful to calculate the typical numbers of photons produced by a light source per second and to compare this with the numbers of photoinitiator molecules commonly employed.
Regarding photons produced by a light source, the following terms and relationships are defined:
I = irradiance of light source (mW/cm2),e.g. 500
λ = wavelength of light (nm), e. g. 470
υ = frequency of light (Hz)
c = velocity of light [3×108m/s]
h = Planck’s constant [6.626×10-34J.s]
E = h.υ = h.c/λ
E = energy of 1 photon of light = 4.226.10 -1 J, for λ470
Nph = number of photons emitted per second and per square centimetre.
Nph = I/E = I [mW/cm2 or mJ/(s.cm2)/E [J]
Thus, for a typical blue light wavelength: λ470
Nph = 1.185 × 1018.1 × 1018 photons/(s.cm2)
How many molecules of CQ are in a disk of monomer?
d = 5mm
h = 2mm
A = π(2.5)2 ≈ 20 mm3. V = A.h = 40 mm3.
If monomer density = 1.0g/cm3 = 0.001g/mm3,
then monomer mass = 0.001×40=0.04 g.
The concentration of CQ typically = 0.2 mass %.
Hence, within the monomer, the CQ mass fraction is f =0.002.
Hence, the mass of CQ is MCq = f × 0.04 = 8×10-5g.
The molecular mass of CQ is m = 166g/mole;
hence, in 40mm3 the number of moles of CQ = MCQ /m = 8×10-5/166.4.8×10-7.
Hence, in 40 mm3 the number of molecules of CQ = (MCQ/m) × NA (Avogadro’s number).
= (4.8×10-7) × (6.023×1023) = 0.289×1018 0.3×1018
With a composite consisting of 30 % monomer, the number of CQ molecules in a 40-mm3 disc is calculated as follows:
NCQ = (0.3×1018) × 0.3 = 0.09×1018 0.1×1018
This is the CQ content, in a material thickness of 2 mm.
This can be compared with the number of photons supplies per second per 20 mm2 disc area:
Nph.0.2 × 1018 photons per second per 20 mm2.
It is concluded that the number of photons arriving per second is comparable to the number of CQ molecules in the corresponding area or volume of the material.
Extent of Polymerisation
It is evident from many studies [63 – 66] that all of the dimethacrylate monomers exhibit considerable residual unsaturation in the final product. This ranges from 25 to 45 %, or degree of conversion (DC) ranging from 55 to 75 %. The nature of the unpolymerised resin is of considerable concern, especially in terms of deleterious effects on the mechanical properties and dimensional stability of the restoration, but less so in terms of biocompatibility [67]. The unconverted methacrylate groups must reside in the polymer network either as residual monomer or (a majority) as pendant side chains (PSC) which extend from the main chains by virtue of having reacted at only one end of the difunctional molecule. A further possibility is a cyclisation reaction. As residual monomer, these molecules function as plasticising agents which can reduce the properties of the polymer network. This occurs until such time as the monomers leach from the composite into the oral environment. Pendant side chains act as permanent plasticisers in the composite; hence, it is desirable to increase DC in order to produce stiffer and more durable resins, although, for a given composite, shrinkage increases with DC.
For a given VLC product the DC depends on the (a) light irradiance reaching the composite surface, (b) time of exposure and (c) thermal energy within the system.
Thermal and Spectroscopic Probes
Several techniques have been used to measure the extent of the reactions and to identify the formation of radicals.
Photo-DSC
Differential scanning calorimetry (DSC) can determine the rate of reaction and can be applied to the development of kinetic models. It has been used successfully to predict the incomplete polymerisation in commercial dental light-cured composites [71].
FTIR Spectroscopy
The unpolymerised carbon-carbon double bonds give rise to an infrared (IR) absorption at 1638 cm-1, which enables determination of DC either by analysis of the filled composite, using multiple internal reflection IR, or by analysis of the unfilled resin films in transmission after extraction of the filler with a suitable organic solvent. The methods appear to provide comparable results for the same resin composites, although data suggest that DC is slightly reduced in composites containing large-volume fractions of quartz or barium glass [72].
NIR Spectroscopy
Near-infrared spectroscopy can measure Overton and combination bands of the C = C vibrations [75]. This has the capability of measuring relatively thick specimens.
FT-Raman
Solid-State NMR
EPR Spectroscopy
During photo-polymerisation, radicals are generated from the CQ/amine exciplex and these in turn generate radicals on the growing polymer chains. The former radicals are unlikely to have an extended lifetime, but the polymer radicals may be expected to persist for some time after the apparent cessation of polymerisation following the vitrification of the resin-composite mass. The presence and lifetime of free radicals may be detected by electron paramagnetic resonance (EPR) spectroscopy [83]. In other cases, radicals have been detected with much longer lifetimes. The persistence of these free radicals is a necessary, but not a sufficient, condition for further polymerisation, for instance, at elevated temperatures [84 – 86].
DMTA and DETA
DMTA and DETA were used to investigate the formation of network in dental resin formulations [87].
Development of Mechanical Properties
Surface Hardness
The hardness of the top surface of a VLC composite resin specimen is observed to increase with time at ambient temperatures after the cessation of light irradiation, although there has been variation in the reported time scale [88 – 90]. The surface hardness changes should at least partially reflect changes in the bulk properties. An empirical mathematical model was proposed for this effect by Johnson et al. [91] based on non-linear regression. This incorporates exponential terms of the form derived previously, but involves two time constants.
Bulk Elastic and Visco-Elastic Compliance
The time dependence of development of Young’s modulus in flexure was reported [92], and this followed a similar pattern to that of shrinkage and hardness. Similar changes in visco-elastic properties, such as static creep and creep recovery [93], are apparent following cure initiation as the network develops.
General Polymerisation Mechanism for Light-Activated Monomers
The principal chain-reaction steps involved subsequent to photo-activation of free radicals (A*) are as follows, with the associated rate constants [k…]:
Initiation [kj]
|
A*
|
+
|
M
|
6
|
AM
|
Propagation [kp]
|
Mn⋅
|
+
|
M
|
6
|
Mn+1⋅
|
Termination [kt]
|
Mn⋅
|
+
|
Mm⋅
|
6
|
M(n+m)
|
Inhibition/retardation [kx]
|
Mn⋅
|
+
|
X
|
6
|
MnX⋅ (inactive)
|
R⋅
|
+
|
X
|
6
|
RX⋅ (inactive)
|
A, M and X denote the photo-reducer (usually an amine), the monomer and the inhibitor, while R and Mnare the initiator and polymer radicals. In the presence of certain chemicals, chain transfer is a further mechanistic possibility.
An active centre is created when a free radical attacks the π-bond of a molecule of monomer. Propagation involves growth of the polymer chain by rapid sequential addition of monomer to the active centre. With vinyl chain polymerisation (e.g. methyl methacrylate or methacrylate end groups) there is head to tail or head to head; the former predominates because attack at the methylene [CH2
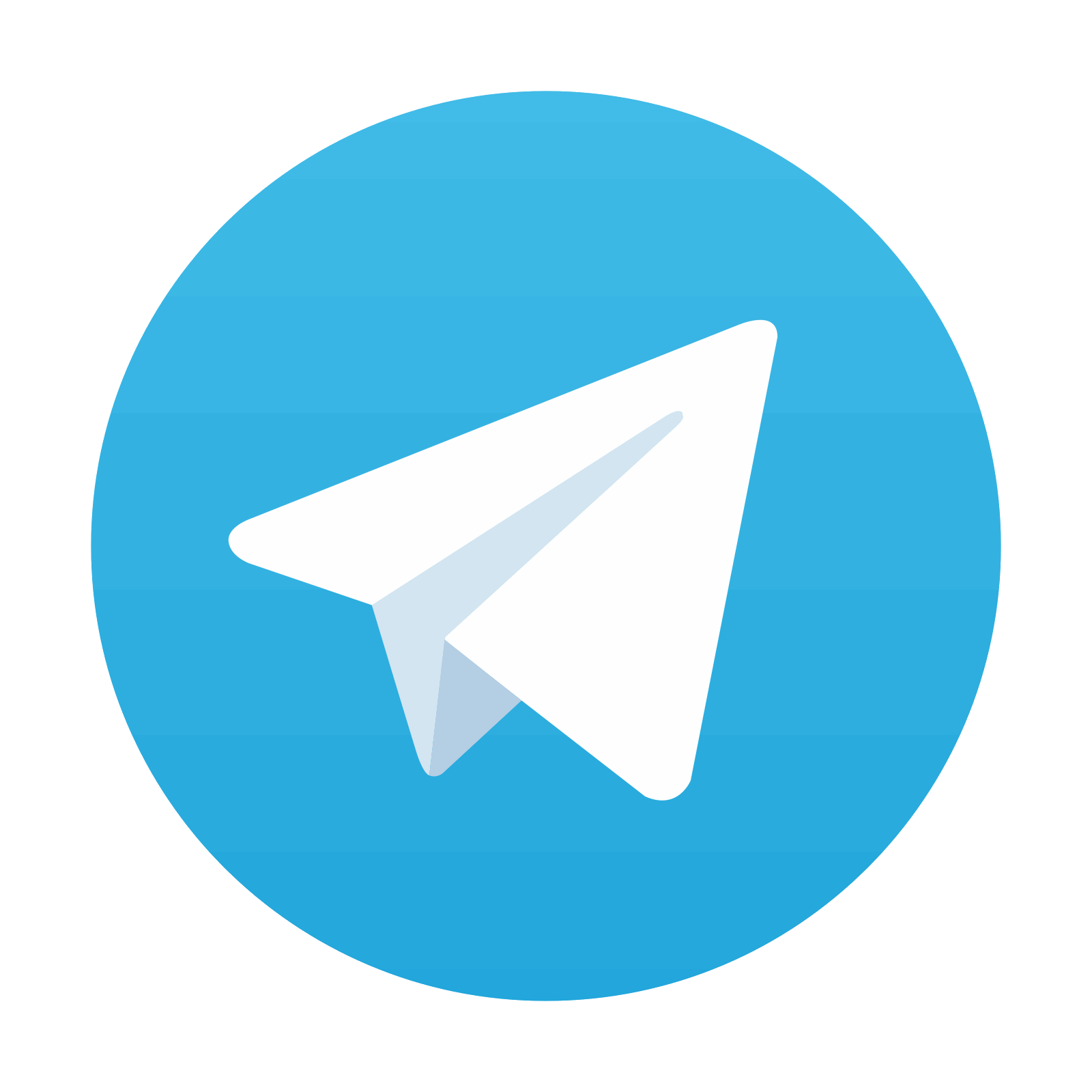
Stay updated, free dental videos. Join our Telegram channel

VIDEdental - Online dental courses
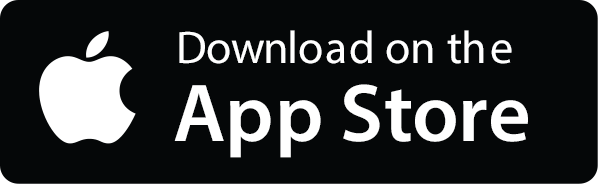
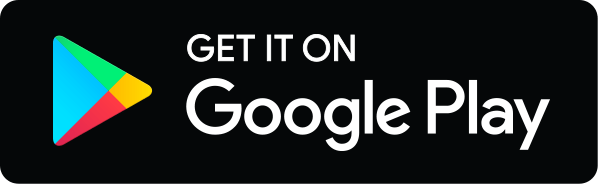