Dental stem cells (DSCs)
In vitro hepatic differentiation
Animal model
Remarks
Reference
Human tooth germ progenitor cells (TGPCs)
DMEM low glucose, 2 % FBS, 100 ng/mL a-FGF (5 days); DMEM low glucose, 2 % FBS, 20 ng/mL HGF (5 days); 20 ng/mL DMEM low glucose, 2 % FBS, 20 ng/mL HGF, 10 nM Dexamehtasone, ITS-X, 10 ng/mL oncostatin M (11 days)
Liver fibrosis in CCl4 treated rats
TGPCs subjected to in vitro hepatic differentiation had therapeutic effect on CCl4-induced liver injury (portal veins)
Ikeda E. et al. [4]
Human dental pulp stem cells (DPSCs)
20 ng/mL HGF (5 days), 2 % FCS; 10 ng/mL oncostatin M, 10 nM Dex, 1 % ITS-X (15 days)
No in vivo model
Differentiation of DPSCs into hepatocyte like-cells
Ishkitiev et al. [22]
Human dental pulp stem cells (DPSCs)
DMEM low glucose, 1 % FCS, 20 ng/mL HGF, 10 ng/mL oncostatin, 10 mM nicotinammide, 1.25 μg/mL LDL,, 10 ng/mL FGF-4, 4 μg/mL insulin, 1.25 g/L glucose, 180 mg/L linoleic acid (40 day)
No in vivo model
Hepatic function of differentiated DPSCs
Ferro et al. [18]
Human tooth-pulp stem cells (HTCP) CD117+
Serum free-DMEM, 1 % ITS-X, 100 μg/mL embryotrophic factor (ETF) (until 70 % confluence); 20 ng/mL HGF (5 days); 10 ng/mL oncostatin M, 10 nM Dex (15 days)
No in vivo model
H2S increased the ability of human tooth-pulp cells to undergo hepatogenic differentiation
Ishkitiev et al. [19]
Human dental pulp stem cells (DPSCs) and human exfoliated deciduous teeth (SHED) CD117+
Serum free DMEM, 1 % ITSX, 100 μg/mL embryotrophic factor (ETF) (until 70 % confluence); 20 ng/mL HGF (5 days); 10 ng/mL oncostatin M, 10 nM Dex (15 days)
No in vivo model
Hepatic differentiation of DPSCs and SHED in serum free medium
Ishkitiev et al. 2012 [17]
Cryopreserved human dental pulp stem cells (hDPSCs) isolated from vital extracted teeth with disease.
Serum deprived IMDM medium, 20 ng/mL HGF, 10 ng/ml β-FGF, nicodidamine 0.61 g/L (7 days). IMDM, 20 ng/mL oncostatin M, 1 μM Dex, 50 mg/mL ITS (34 days)
No in vivo model
Hepatic differentiation of hDPSCs from cryopreserved dental pulp tissues of vital extracted teeth with disease
Chen et al. [23]
Human exfoliated deciduous teeth (SHED) CD117+
DMEM serum free, 1 % ITS-x 100 μg/mL embryotrophic factor (ETF) (until 70 %); 20 ng/mL HGF (5 days); 10 ng/mL Oncostatin M, 10 nM Dex (15 days)
No in vivo model
The level of hepatic differentiation in SHED compared with BM-MSCs was the same or higher. H2S increased the level of hepatic differentiation.
Okada et al. [24]
Human exfoliated deciduous teeth (SHED) CD117+
Serum free medium, 20 ng/mL HGF (5 days); 10 ng/mL oncostatin M, 10 nM Dex, HGF 10 ng/mL (15 days)
Acute liver injury (ALI) and secondary biliary cirrhosis in rats
hdSHED engraft morphologically and functionally into the livers of rats (transplantation to the spleen)
Ishkitiev et al. [21]
Human dental pulp stem cells (DPSCs)
DMEM 2 % FCS, 20 ng/mL HGF (5 days); DMEM 2 % FCS, 20 ng/mL HGF, 10 ng/mL oncostatin M, 10 nM Dex (15 days)
CCl4−induced liver fibrosis in mice
Combined treatment of DPSCs and melatonin for the treatment of liver fibrosis (teil veins)
Cho et al. [25]
Human exfoliated deciduous teeth (SHED)
No in vitro hepatic differentiation
CCl4-induced liver fibrosis model mice
SHED transplantation recovered liver dysfunction and led anti-fibrotic and anti-inflammatory effects (intrasplenically transplanted into mice)
Yamanaza et al. [20]
Several studies have showed that biochemical factors that have an essential role in liver development are also shown to induce hepatic differentiation of DSCs. Moreover, those biochemical factors have also been useful for maintaining hepatocytes cultures in vitro [26]. For example, the use of HGF and FGF-4 as inductors for hepatic differentiation of multipotent adult progenitor cells (MAPS) [27] and human umbilical cord blood-derived mesenchymal stem cells [28] are reported. HGF is considered a key growth factor for liver growth and function that controls different processes, including morphogenesis, motogenesis and mitogenesis.
HGF initiates multifunctional regulatory roles by interaction with HGF receptor (c-Met) [29], a transmembrane protein-tyrosine kinase expressed in normal hepatocytes, intestinal, ovarian, epithelium and endometrial endothelia [7]. HGF possesses strong antifibrotic activity and contributes to the onset or progress of liver fibrosis/cirrhosis and directly promotes the differentiation of MSCs into hepatic lineages, and transplantation of these cells improves liver injury in rats [30]. Therefore, HGF can potentially be used for the treatment of acute hepatic failure (AHF) [31] and CCl4 induced liver necrosis [32]. FGF is another growth factor that works as mitotic agent for a variety of cells and tissues [33]. It stimulates differentiation and proliferation by binding to its receptor epidermal growth factor receptor (EGFR) [34]. Both, HGF and FGF have important roles in liver development, but it is unclear if they can induce definitive endoderm without the contribution of additional factors.
Recently, Lin et al. showed that hepatocytes differentiation of BM-MSCs is induced by cooperation of HGF and FGF-4 through the HGF/c-Met, a transmembrane receptor endowed with tyrosine kinase activity [35]. In some studies, in order to induce the late hepatic differentiation, the medium was supplemented with OSM [36], insulin-transferrin selenium (ITS) [37], Dex [38] and embryotrophic factor (ETF) [39]. OSM is a cytokine belonging to the interleukin 6 (IL-6) family and is recognized as having an essential role in liver development, it is essential for maturation of hepatocytes in combination with Dex [40]. Moreover, OSM plays a crucial role in progression of hepatocyte development through the signaling pathway of activator factor and signal transducer of transcription 3 (STAT 3) [41]. Some studies have shown that Dex is able to preserve the expression of transcription factors essential for the regulation of liver specific genes [38, 42]. Whereas, insulin-transferrin selenium (ITS) have been shown to induce survival and proliferation of primary hepatocytes [43].
In summary, HGF, EGF and OSM have important effects on the maintenance of primary human hepatocytes in vitro [44] and several studies have proposed that there is a need to expose cells to HGF and FGF4 in a sequential approach in order to increase the hepatic differentiation efficacy of BM-MSCs [45].
BM-MSCs and DPSCs have similar function and morphology, and thus several studies speculated that DSCs have the ability to differentiate not only in tissue of ectodermal and mesodermal origin, but also in those of endodermal origin. As a consequence, the combination of HGF, EGF, OSM, Dex and ITX previously established for ESCs and BMSCs was widely used in protocols to differentiate DSCs to hepatocytes [4, 17–19, 21, 22, 25].
Various Tissue Sources of DSCs
DSCs obtained from various sources can be directed towards hepatic differentiation using soluble factors as listed in Table 1. For example, Ikeda et al. demonstrated that stem cells derived from the neural crest-derived dental tissues can differentiate into endoderm cell lineages [4]. In particular, they showed that the tooth germ progenitor cells (TGPCs) were able to differentiate into functional hepatocytes. The differentiated hepatocytes were able to suppress inflammation and liver fibrosis in carbon tetrachloride (CCl4) treated rats [4]. They further showed that the hepatic differentiated TGPCs can improve to the restoration of liver function as evaluated by the expression of hepatic serum marker such as albumin, bilirubin, aspartate aminotransferase (AST) , and alanine aminotransferase (ALT) . In their protocol, TGPCs were first treated with a-FGF and HGF followed by the addition of the maturation factors OSM and Dex when the TGPCs already were committed to the hepatic fate. The changes in morphology were evident and the production of albumin was observed starting from day 10.
In a similar study, Ishkitiev et al. showed that the differentiation of DSCs into hepatic-like cells was possible from DSCs obtained from full-grown wisdom or primary tooth pulp [22]. In fact, they showed the differentiation of wisdom tooth pulp cells (WTPCs) and deciduous tooth pulp cells (DTCPs) into hepatocytes-like cells using HGF, Dex, OSM and ITS-x. The differentiated DSCs produced proteins specific to hepatocytes and acquired hepatic specific functions including the storing of glycogen and the production of urea [22]. Subsequently, the same research group showed that hydrogen sulfide (H2S) at physiological concentrations improved the capacity of human tooth-pulp stem cells (HTCP) to undergo hepatogenic differentiation [19]. The CD117-positive cells (CD117+) were isolated from deciduous HTCP by using a magnetic cell sorting system and then cells were grown in medium supplemented with HGF, ETF, OSM and insulin-transferrin-selenium-x (ITS-x) with or without H2S. The results demonstrated that the hepatic markers albumin (ALB), α-fetoprotein (αFP), and carbamoyl phosphate synthetase (CPS-1) were expressed more in H2S treated HTCP with respect to control. This study demonstrate that H2S at physiological concentrations is able to improve hepatogenic differentiation of HTCP in vitro [19].
More recently, Okada et al. have compared the hepatic differentiation of SHEDs with those of BM-MSCs in vitro with or without H2S [24]. In their experiments they observed that either SHEDs or BM-MSCs CD117+ without H2S expressed similar levels of stem cell transcription factors. However, after differentiation, the expression of hepatic markers such as ALB, αFP, CPS-1 was significantly higher in SHEDs compared to BM-MSCs. Unlike the previous results, no significance differences were observed between H2S-treated SHEDs and BM-MSCs after hepatic maturation in terms of ALB, αFP, CPS-1 expression, glycogen production and urea concentration. These results strongly suggest the possibility to use SHEDs in combination with H2S for clinical treatment of liver disease.
In another study, Ferro et al. further demonstrated the hepatic-differentiation potential of DSCs [18]. They reported that under hepatic stimuli, DSCs increased their mRNA expression of albumin and hepatocyte growth factor receptor (C-met/HGF-r). Moreover, they showed that DSCs acquired the pattern of cytokeratin expression (CK8, CK18 and CK19) of normal hepatocytes and exhibited some hepatic functions such as the production and secretion of albumin [18].
The previous studies used fetal bovine serum (FBS) complemented medium for hepatic differentiation. The use of animal products in the differentiation medium represents a enormous obstacle for the application of in vitro cell-therapy methods in clinical applications due to the fact that FBS can cause hypersensitivity reaction and can be a potential vector for prion transmission [46]. Therefore, in order to overcome these limitations, Ishkitiev et al. described the differentiation of DPSCs CD117+ and SHEDs CD117+ into a high-purity hepatic lineage using serum-free medium [17].
Recently, Chen et al. have studied the hepatic potential after cryopreservation of DSCs isolated from human dental pulp tissues of teeth affected by disease such as periodontitis and pericoronitis [23]. In their experiments they observed that hepatically differentiated DSC had a polygonal shape and expressed the specific liver genes including αFP, ALB, HNF1α and CK18.
Moreover, urea production and glycogen storage results denoted that the differentiated human DPSCs were functionally similar to normal hepatocytes-like cells. Although research studies regarding hepatic differentiation of DSCs are at beginning, the use of cryopreserved tissue to generate hepatocytes-like cells represents an encouraging alternative for the treatment of liver diseases.
To highlight the clinical potential of DSCs for the treatment of hepatic diseases, Ishkitiev et al. showed that human hepatically differentiated CD117+ SHED (hdSHED) were able to engraft functionally in the liver of experimental rat models of hepatic diseases [21]. In fact, human specific markers such as albumin and αFP were found in the serum of the rats, confirming that transplanted hdSHED can be incorporated into structural components of the liver of rats having acute injury. This study showed that in vitro differentiation of DSCs in hepatocyte-like cells and the following transplantation could be a useful method to treat human liver diseases.
As described above, the transplantation of human TGPS in the liver of CCl4 injured mice were able to inhibit fibrosis and inflammation [4]. Using the same animal model, Cho et al. recently reported that the use of melatonin in combination with the transplantation of human DPSCs drastically decrease liver fibrosis demonstrating that the treatment of DPSCs transplantation combined with melatonin administration could be used to improve liver functions [25]. Melatonin promoted hepatic differentiation by regulating the NF-κB pathway, p38, BMP, and ERK. Moreover, the grade of liver fibrosis measured as restoration of ALT, ASP and levels of ammonia was lower in CCl4-injured mice treated with a combination of DPSCs transplantation and melatonin administration compared to either melatonin administration or DPSCs transplantation alone [25].
In the earlier studies, DSCs were pre-differentiated in vitro before transplantation in animal model [4, 21, 25]. Recently, Yamanaza et al. demonstrated that naïve SHEDs, not previously in vitro differentiated, were able to improve hepatic dysfunction and directly transform into hepatocytes in CCl4 treated mice [20]. Moreover, these in vivo SHEDs converted hepatocytes participated in the hepatic recovery via tissue replacement and possessed anti-fibrotic and anti-inflammatory effects.
DSCs-Derived Induced Pluripotent Stem Cells (iPSCs) as Potential Cell Source
Recently, human somatic cells have been reprogramming into induced pluripotent stem cells (iPSCs) by addition of four transcription factors [47, 48]. These results offer the possibility of using adult somatic cells to obtain cells that shared many features with ESCs but with the advantage of being obtained from an adult source. As a consequence, in the last years, it became possible to obtain iPSCs from several adult tissues, including the hepatic tissue. iPSCs are typically generated by ectopic expression of four transcription factors, OCT3/4, Sox2, Klf4 and c-Myc in fibroblasts. Several researchers have demonstrated that iPSCs can be derived from different cell sources including skin fibroblasts [47], keratinocytes [49], blood progenitor cells [50], pancreatic cells [51] and primary human hepatocytes [52].
It has recently been showed that iPSCs can be considered a new cell source for stem cell therapy of liver diseases because they were able to differentiate into functional hepatocytes [53, 54]. Because of its accessibility and differentiation potential, DSCs has gradually drawn attention in the applications of iPSCs in regenerative medicine, in fact, DSC has been widely demonstrated as a source of iPSCs [55, 56]. The knowledge previously acquired in hepatic-differentiation of DSCs was successfully applied in iPSCs and two reports of efficient methods to obtain hepatocytes-like cells from dental pulp derived-iPSCs (DP-iPSC-Heps) have been published [57, 58]. A summary of these reports is shown in Table 2.
Table 2
Studies that assessed the use of human dental pulp derived iPSCs (DP-iPSCs) for liver regeneration
Dental stem cells (DSCs)
|
In vitro hepatic differentiation
|
Animal model
|
Remarks
|
Reference
|
---|---|---|---|---|
Induced pluripotent stem cells (iPSCs) from dental pulp stromal cells
|
RPMI 0.5 mg/ml Albumin fraction V, 100 ng/mL activin A (1 day); 0.15 and 1 % ITS (2 days); 30 ng/mL FGF-4, 20 ng/mL BMP-2 (4 days); 20 ng/mL HGF, 20 ng/mL KGF, oncostatin M, 0.1 μM Dex (5 days); DMEM N2 B27, glutamax, 0.1 mM β-mercaptoethanol (3 days)
|
Acute hepatic failure (AHF) mouse model induced by thioacetamide (TAA)
|
Engraftment of iPSC-Heps with injectable hydrogel (CHC) containing HGF reduced the TAA-induce hepatic necrosis and rescued liver function
|
Chiang et al. [58]
|
Transduction of pMX vectors encoding the transcription factors: OCT4, Sox-2, Klf4 and c-Myc
|
||||
Induced pluripotent stem cells derived from human dental pulp (DP-iPSCs)
|
Nanostructured amphiphatic carboxymethyl–hexanoyl chitosan (CHC)/ Polyurethane-graft-short-branch polyethylenimine copolymer/ miR122 mixture to accelerate the hepatic differentiation of iPSCs
|
Acute hepatic failure (AHF) mouse model induced by thioacetamide (TAA)
|
miR122-iPSC-Heps improved liver functions and rescued recipient survival
|
Chien et al. [57]
|
Transduction of pMX vectors encoding the transcription factors: OCT4, Sox-2, Klf4 and c-Myc
|
Interestingly, Chiang et al. reprogrammed human dental pulp fibroblasts into iPSCs and induced the differentiation of these dental pulp-derived iPSC (DP-iPSCs) into functional iPSC-Heps [57]. Microarray analysis indicated that DP-iPSC-Heps, normal liver and ESCs derived hepatocytes (ESC-Heps) possessed a similar gene expression profile. Moreover, similar to mature ESC-Heps, DP-iPSCs-Heps acquired regular functions for glycogen storage and LDL uptake during hepatic differentiation. Moreover, DP-iPSCs-Heps expressed several hepatic markers including αFP, hepatocyte nuclear factor 3β (HBF-3β) and cytochrome c P450 enzyme CYP2E1. In their in vivo studies, Chiang et al. used an injectable carboxymethyl-hexanol chitosan hydrogel (CHC) having a continuous release of HGF (HGF-CHC), as delivery vehicle for transplantation of DP-iPSCs-Hep in immunocompromised acute hepatic failure (AHF) mouse model induced by thioacetamide (TAA) [58]. They observed that intrahepatic delivery of CHC-HGF hydrogel coupled with iPSC-Heps decreased the TAA-induced hepatic necrotic area and recovered liver functions, suggesting that HGF-CHC is an excellent vehicle for iPSCs-Heps engraftment in iPSCs based therapy against AHF [58].
In another study from the same group, Chien et al. investigated an alternative strategy to obtain mature hepatocytes-like cells from DP-iPSCs using a nanotechnology based delivery microRNA (miRNA) system. At this purpose, they used CHC to encapsulated the complex of polyurethane-graft-short-branch polyethylenimine copolymer (PU-PEI) and microRNA122 (miR122), a hepatocyte-specific miRNA [57]. Subsequently, PU-PEI complex was used as vehicle to deliver miR122 into DP-iPSCs. miR122 has been chosen because it is associated with some biological functions of the liver [59]. They observed that combination of miR122 plasmids with PU-PEI in nanostructured CHC (CHC/PU-miR122) were able to enhance miR122 delivery into human DP-iPSCs. As consequence, after the entry of miR122 into DP-iPSCs, these cells were able to successfully differentiate into iPSC-Heps having mature functions of hepatocytes [57]. In this work, using microarray analyses it has been observed that CHC/PU-PEI-miR122 shifted gene expression profile of DP-iPSCs toward that of the liver. Moreover, they observed that the delivery of a construct containing CHC/PU-miR122 and iPSCs-Heps into thioacetamide (TAA)-injured rat liver was able to significantly rescued liver functions. These results demonstrated for the first time that miR122-iPSCs-Heps transplantation could be used as new therapeutic approach to obtain liver regeneration of recipients with acute hepatic failure (AHF) .
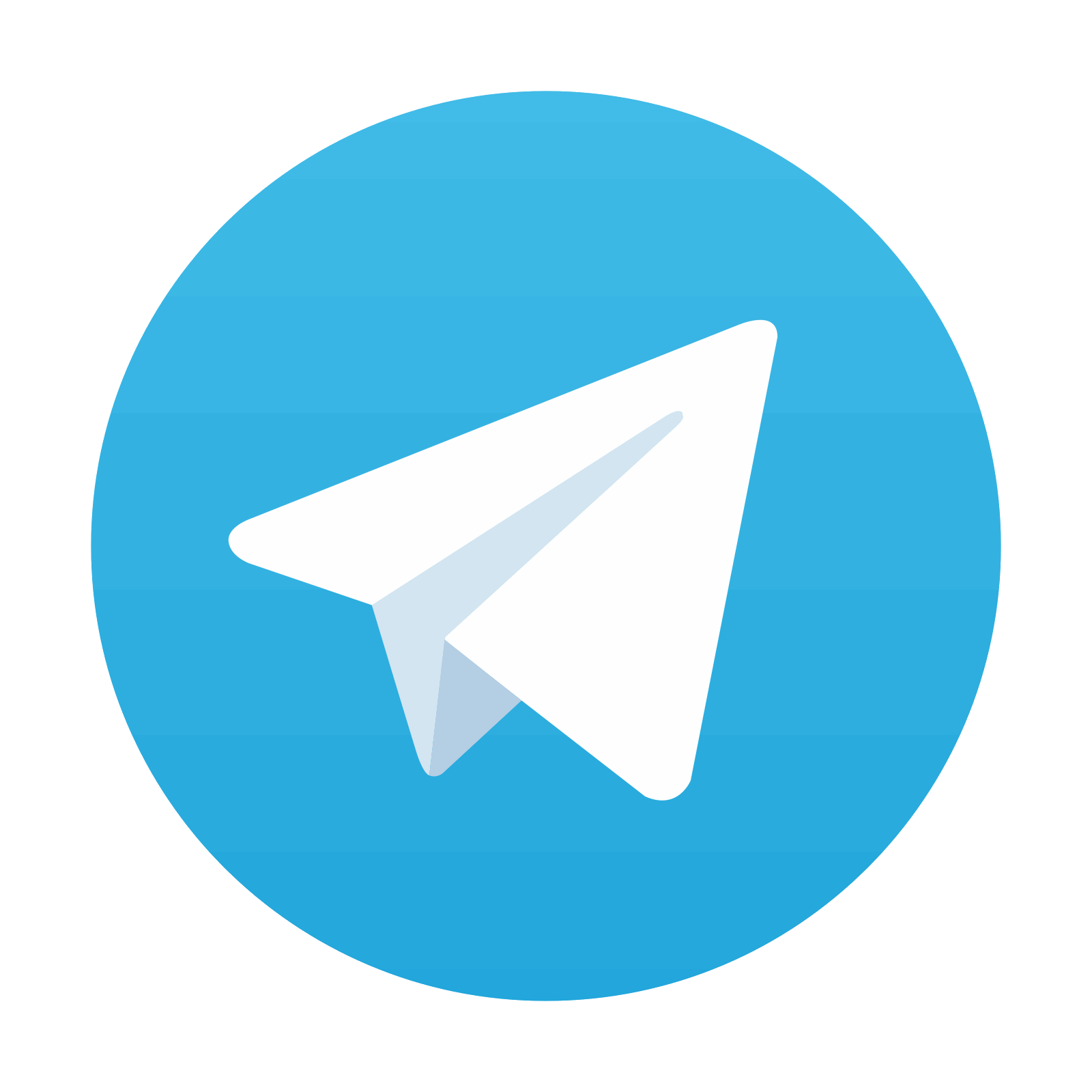
Stay updated, free dental videos. Join our Telegram channel

VIDEdental - Online dental courses
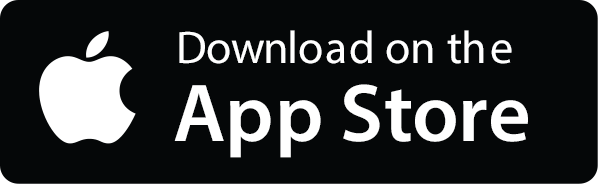
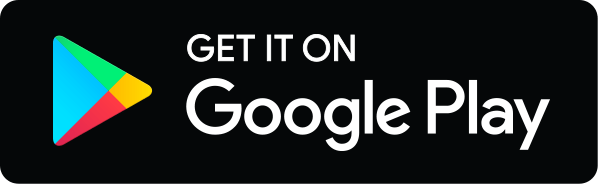