The science of bone regeneration has evolved into a highly complex and specialized area of study. The understanding of the molecular and cellular cascade of events involved in bone repair and regeneration continues to evolve at a rapid pace. Promising technologies, such as tissue engineering, recombinant growth factors, and gene therapy, offer tremendous hope for the future. Notwithstanding these recent advances, the principle of guided tissue regeneration (GTR) continues to play a major role in contemporary clinical practice and is particularly important in the management of localized bone defects associated with dental implants.
The development of GTR has a long and interesting history. As early as 1944, the principle of compartmentalization to achieve regeneration of specific tissues was under investigation. Researchers in the field of neural regeneration, using allogeneic aorta as a protective conduit over the cut ends of a peripheral nerve, reported regeneration of nerve fibers, even in the absence of a distal segment. It had previously been observed that nerve regeneration was prevented by fibrous tissue ingrowth and scarring.
In 1957, Murray found that if a section of cortical bone was removed from the dog ilium and subsequently protected with a plastic cage, the interior of the cage would fill with new bone. Murray also found that if the three-dimensional design of the cage permitted, new bone would form on the cortical surface surrounding the defect and also proliferate vertically within the cage. Hurley et al., seeking to improve bone regeneration in spinal fusion, found that insertion of a cellulose acetate filter over the grafted site effectively excluded the overlying, fully differentiated tissues from the bony defects. Subsequently, Linghorne reported on tube-guided osteogenesis in fracture repair. In this study, discontinuity defects 15 mm in length were produced in the fibulae of dogs by removal of a section of bone and periosteum. A hollow polyethylene tube was then placed across the gap, connecting the cut ends of the bone. It was demonstrated that in the presence of the tube, osseous healing occurred instead of a fibrous union.
The work of Murray and Linghorne was expanded on by Melcher using dome-shaped cellulose acetate barriers over surgically created defects in the rat. Melcher theorized that the barrier served two purposes: to provide protection of the hematoma from invasion by nonosteogenic extraskeletal soft tissue and to prevent physical distortion of the hematoma by pressure from the overlying tissues.
The use of GTR in the realm of oral surgery was first reported by Boyne, who used a cellulose acetate filter to regenerate bone in surgically created mandibular defects. Later he would use the same material to line the interior of metallic mesh cribs in combination with autogenous bone to treat major mandibular defects. Using a slightly different material, Kahnberg later described the successful use of perforated Teflon mantle leaf on regeneration of mandibular bone defects in rabbits.
The potential for the regeneration of specific, differentiated periodontal structures was described by Linghorne and O’Connell in 1950. In surgically created bone defects adjacent to teeth and grafted with autogenous bone chips, they observed a new connective tissue attachment and regeneration of bone, cementum, and the periodontal ligament. An occlusive membrane was not used in this study; however, they observed that in cases where a new connective tissue attachment formed coronal to the bone defect, effectively excluding the more aggressive gingival epithelial cells, regeneration of alveolar bone and cementum occurred. Conversely, if connective tissue attachment failed to occur coronally, allowing the down growth of oral epithelium, there was subsequent failure of osteogenesis and cementogenesis.
In 1976, Melcher published a frequently quoted paper on the repair of periodontal tissues. Taking the concept well beyond the epithelial exclusion theory, his work delineating the specific role and kinetics of progenitor cells would herald the development of regenerative therapy in periodontics.
The use of GTR membranes in implantology, more specifically the concept of guided bone regeneration (GBR) or osteopromotion, was recognized as a method of alveolar ridge augmentation and for managing localized defects associated with the placement of dental implants. These concepts would fundamentally change the practice of implantology, increasing the ability of clinicians to provide more predictable restoration of form, function, and aesthetics through more ideal implant placement.
▪
FUNCTIONAL AND DESIGN REQUIREMENTS OF GTR MEMBRANES
The requirements for achieving successful bone regeneration include the attraction of appropriate precursor cells to the target site, an abundant nutrient supply, appropriate molecular signals, and a stable, protected environment conducive to the development of the target tissue. A primary function of the GTR membrane is to provide isolation and protection of the initial blood clot, which is crucial for the proper elaboration, distribution, and function of growth factors, cytokines, and inflammatory mediators involved in wound healing. In addition, the membrane should provide sufficient protection from bacterial invasion. The membrane should have adequate density to prevent infiltration of cells from nonosteogenic tissues, and the membrane must remain intact for as long as necessary for the defect to be populated by cells derived from adjacent bone.
STABILITY
During the first few hours and days, inflammatory cells and fibroblasts are predominant within the blood clot. Fibroblasts then lay down the collagenous framework, which is ultimately mineralized by osteoblasts to form new bone. Stability of the wound, as in the case with fracture healing and osseous implant integration, is required for conversion of mesenchymal cells into the osteoblastic phenotype. Continuous motion results in the production of prostaglandins and prolongation of the inflammatory response. Thus, the overlying membrane must provide a stable environment with minimal micromotion; otherwise, formation of fibrous tissue rather than bone will result.
SPACE MAINTENANCE
The earliest investigations of GBR revealed that successful GBR was dependent on the creation and maintenance of adequate space under the membrane to allow migration of cells and ingrowth of blood vessels from adjacent osteogenic tissues to occur. In the oral cavity, pressure from overlying soft tissues, muscles, or prostheses may result in membrane collapse and failure. In large defects, this requires the presence of particulate bone grafting materials or some other method of providing mechanical support to the membrane, such as the use of titanium tenting screws. Alternatively, membranes reinforced with titanium struts may be used, or titanium mesh may be used in conjunction with an occlusive membrane. Smaller, vertically oriented defects tend to be less critical in this regard as long as the membrane is being adequately supported by the adjacent bone.
BIOCOMPATIBILITY
Upon implantation, the membrane material should evoke minimal inflammatory response and remain biologically inert for the duration of the regeneration procedure. Biocompatibility is related to the chemical, structural, and physical characteristics of the material. Bioresorbable materials may present additional concerns with regard to inflammatory or cytotoxic by-products released during their degradation. Other consequences of degradation, such as proteolytic activity, free radical formation, or local change in pH, all of which have the potential to interfere with bone formation, are of concern. Materials that have been found to be suitable for GBR include cellulose acetate, Teflon mantle leaf, expanded polytetrafluoroethylene (ePTFE), high-density polytetrafluoroethylene (dPTFE), type I bovine collagen, porcine collagen, laminar freeze-dried bone, polylactide and polyglycolide copolymer, silicone sheet, lyophilized dura mater, autologous periosteum, and latex rubber dam.
EASE OF USE
Because alveolar bone defects often have complex three-dimensional morphology, GTR membranes should be manufactured from materials that are easy to trim, adapt, and place with a high degree of precision. Once placed, the membrane should lie passively within the wound and adapt well to the surrounding bone and soft tissues, yet should have enough stiffness to withstand collapse into the defect.
▪
SURGICAL PRINCIPLES
Although a wide variety of membrane materials are available, both resorbable and nonresorbable, the fundamental surgical principles involved in their use are remarkably similar. Achieving predictable success in GBR requires careful planning, meticulous technique, and attention to detail. Clinicians becoming familiar with this technique should also realize that certain defects are more challenging than others, and some defects are better managed with alternative techniques. A number of factors have been identified over the years that, taken in their entirety, will help ensure success, regardless of the material used.
PATIENT SELECTION AND PREPARATION
Because infection is the most significant risk factor associated with augmentation procedures, candidates for GTR should exhibit excellent oral hygiene. Chronic periodontal disease should be controlled, and the dentition should be in an optimal state of health. Sites with inadequate soft tissue thickness or poor tissue quality will benefit from soft tissue grafting before regenerative therapy. The use of tobacco in any form in the perioperative period should be strongly discouraged. Cigarette smoking, heavy alcohol use, uncontrolled systemic diseases such as diabetes mellitus, or any other condition typically associated with poor wound healing will increase the incidence of complications. The presence of active infection at the graft site, characterized by pain, erythema, swelling, or purulent drainage, is a contraindication to regenerative therapy.
In the preoperative phase, consideration should be given to the type of prosthesis, if any, to be used during the healing period following GTR procedures. The use of soft tissue borne prostheses should be avoided in favor of tooth-supported prosthetics, if possible. This will help to prevent compressive forces that can result in a reduction of graft volume, dehiscence, or other wound healing complications. If the use of a tissue-borne prosthesis is unavoidable, it should remain out of functional occlusion and be removed from the mouth as much as possible for the first 3 weeks.
Systemic antibiotic prophylaxis should be used for regenerative procedures involving the placement of membranes and/or graft materials, but is generally not necessary beyond the first 7 days postoperatively. Chlorhexidine rinse should be prescribed to begin 12 hours preoperatively and then locally applied to the surgical site until suture removal. Where membrane exposure occurs, local application of chlorhexidine should be done until the time of membrane removal. Patients should be instructed specifically to keep these areas clean and free of bacterial plaque and debris.
INCISION DESIGN
Conservation of blood supply to the flap overlying a GTR membrane is of paramount importance in maintaining viable, healthy soft tissues and minimizing complications. Midcrestal incisions are typically used in edentulous sites, and vertical incisions are made at least one tooth width (5 to 10 mm) from the site of the anticipated membrane margin. A tension-relieving cut-back incision, as described by Sclar, can be employed at the apical extent of vertical incisions as an alternative.
DÉBRIDEMENT
Following flap reflection, meticulous débridement of all soft tissues within and adjacent to the defect should be done with sharp curettage or piezo surgery. In extraction sockets, sharp curettage should be used to remove all remnants of the periodontal ligament. The apex of the socket should be carefully explored, and any residual periapical soft tissue should be thoroughly removed. The surrounding bone should be smooth, level, and free of projections, which would interfere with adaptation of the membrane.
DECORTICATION
Decortication is a principle used in the placement of autogenous grafts in a variety of orthopedic and maxillofacial procedures and may be done in all GBR procedures. Successful bone regeneration cannot occur without the availability of osteoprogenitor cells and an adequate blood supply. The adjacent marrow space is a source of both, and more rapid access to the endosteal compartment is believed to have the potential to enhance bone regeneration. This can be accomplished by the use of a slow-speed round bur and copious irrigation (see Case Report 25-4 , Figure 25-35 ).
The preoperative view ( Figure 25-34 ) demonstrates inadequate ridge width requiring augmentation before placement of endosseous implants. A midcrestal, full-thickness incision and reflection reveals the atrophic ridge, which is perforated with a #2 round surgical bur in preparation for augmentation ( Figure 25-35 ). A composite graft consisting of autogenous bone shavings ( Figure 25-36 ) combined with mineralized and demineralized allograft putty (Exactech, Inc. Gainesville, Fla.) is placed ( Figure 25-37 ). A type I bovine, cross-linked bovine collagen membrane (Osteogenics Biomedical, Inc. Lubbock, TX) is positioned to completely cover the graft ( Figure 25-38 ). Tension-free primary closure is achieved with dPTFE suture ( Figure 25-39 ). After 6 months, the augmented site was well healed ( Figure 25-40 ). On exposure, the graft was found to be well consolidated and endosseous implants were placed ( Figure 25-41 ). Histologic analysis of the grafted ridge revealed 43% bone by volume, 97% of which was vital bone, and 3% residual, nonvital allograft ( Figure 25-42 ). The implants were allowed to integrate for 3 months and then progressively loaded with an acrylic provisional restoration ( Figure 25-43 ).
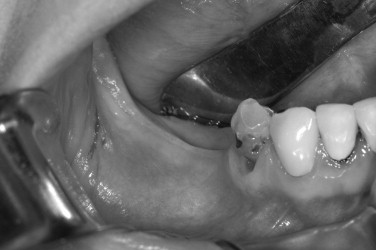
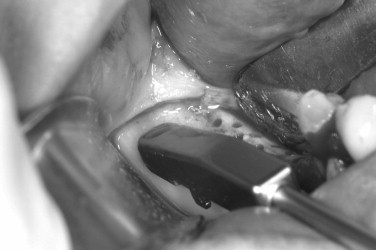
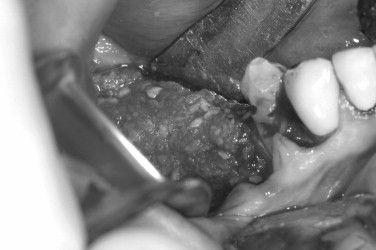
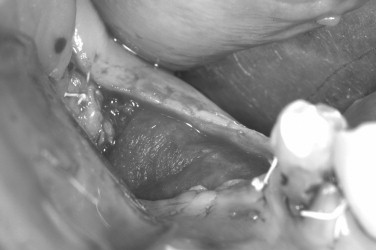
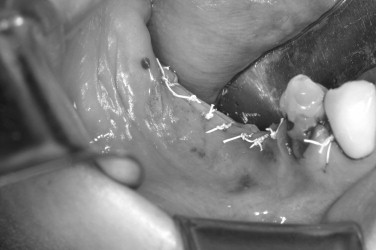
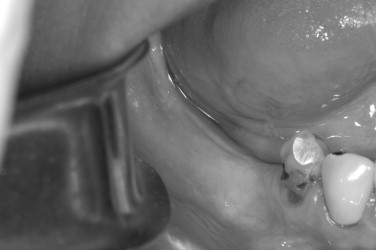
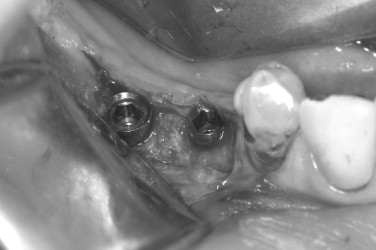
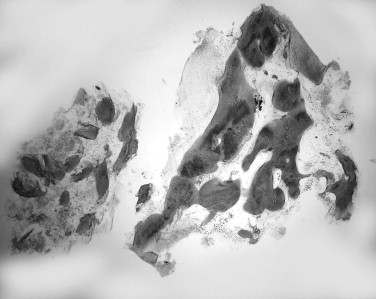
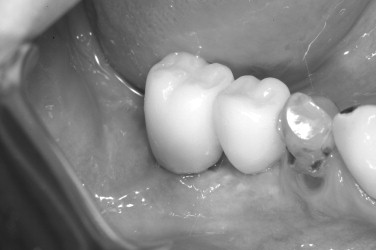
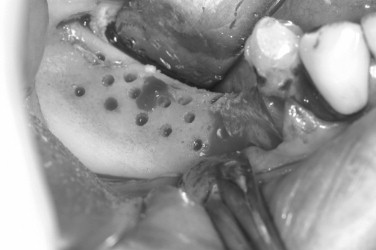
MEMBRANE TRIMMING AND PLACEMENT
When handling and trimming membranes, talc-free gloves should be used to prevent contamination of the surface. To minimize contamination of the membrane during trimming, a template made from sterile foil or surgical wrap may be used to determine the final outline. This information is then transferred to the actual membrane. Sharp corners or frayed edges on the membrane should be eliminated because this can lead to irritation or perforation of the overlying soft tissue. With titanium-reinforced membranes, avoid trimming within 2.0 mm of the titanium struts to prevent inadvertent delamination of the membrane. Properly trimmed, the membrane should completely cover and extend 3 mm to 4 mm beyond the margins of the defect. This distance is usually sufficient to provide an adequate seal between the margin of the membrane and the defect.
A notable exception, however, is when placing a membrane adjacent to natural teeth or between natural tooth roots. In this case, to prevent sloughing of the flap and loss of the interdental papilla, the membrane should be trimmed so that it is no closer than 1.0 mm to the adjacent tooth roots. In addition, avoiding contact of the membrane with the tooth root prevents percolation of oral fluids beneath the membrane, an occurrence that can lead to infection. Similarly, if placement of two membranes is required, they should not overlap in an area that may subsequently become exposed to the oral cavity, such as near the crest of the ridge.
Once the membrane has been trimmed and is in place, the surgeon should reposition the soft tissue flaps to ensure that the membrane is stable and that no wrinkles or folds occur during closure. Membranes that are soft and compliant with the overlying soft tissues may be stabilized adequately by tucking the membrane subperiosteally under the flaps, achieving stability via cell ingrowth or attachment. More rigid materials, which are sometimes required in certain cases, require stabilization with tacks or microscrews to prevent micromotion.
SPACE MAKING
Consideration must be given to creating and maintaining sufficient space under the membrane for bone regeneration to occur. The tendency for membrane collapse is related to both the morphology of the bone defect and the inherent stiffness of the membrane. Narrow, intrabony defects with three or four adjacent walls present less risk of membrane collapse than broad defects with only two or three walls. The most common method of providing additional membrane support and stability is the addition of resorbable particulate bone grafting materials under the membrane. Autogenous bone, allogenic bone, xenografts, or alloplasts may be used individually or in combination. Other methods include the use of tenting screws or perforated mesh in addition to a GTR membrane. Titanium-reinforced membranes are also available, which provide additional stability and added protection against collapse ( Figures 25-1 and 25-2 ). When using large titanium-reinforced membranes, strong consideration should be given to stabilizing these devices with bone tacks or screws.
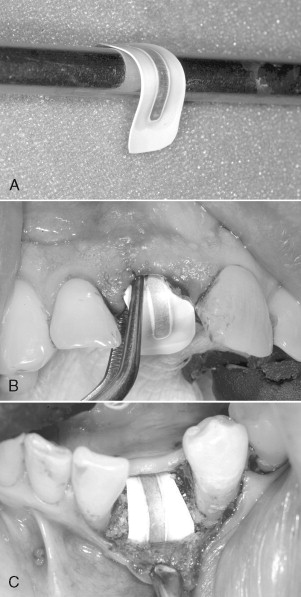
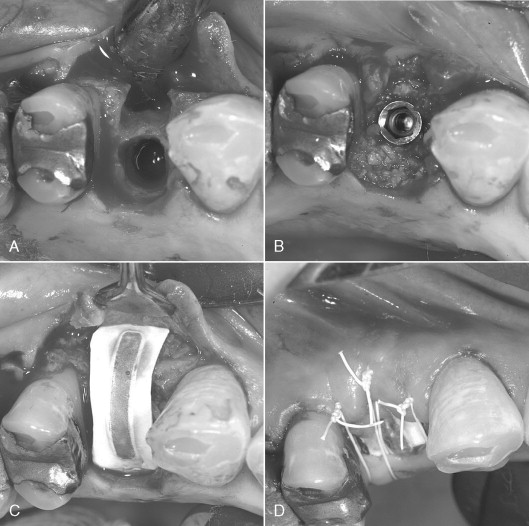
▪
SURGICAL PRINCIPLES
Although a wide variety of membrane materials are available, both resorbable and nonresorbable, the fundamental surgical principles involved in their use are remarkably similar. Achieving predictable success in GBR requires careful planning, meticulous technique, and attention to detail. Clinicians becoming familiar with this technique should also realize that certain defects are more challenging than others, and some defects are better managed with alternative techniques. A number of factors have been identified over the years that, taken in their entirety, will help ensure success, regardless of the material used.
PATIENT SELECTION AND PREPARATION
Because infection is the most significant risk factor associated with augmentation procedures, candidates for GTR should exhibit excellent oral hygiene. Chronic periodontal disease should be controlled, and the dentition should be in an optimal state of health. Sites with inadequate soft tissue thickness or poor tissue quality will benefit from soft tissue grafting before regenerative therapy. The use of tobacco in any form in the perioperative period should be strongly discouraged. Cigarette smoking, heavy alcohol use, uncontrolled systemic diseases such as diabetes mellitus, or any other condition typically associated with poor wound healing will increase the incidence of complications. The presence of active infection at the graft site, characterized by pain, erythema, swelling, or purulent drainage, is a contraindication to regenerative therapy.
In the preoperative phase, consideration should be given to the type of prosthesis, if any, to be used during the healing period following GTR procedures. The use of soft tissue borne prostheses should be avoided in favor of tooth-supported prosthetics, if possible. This will help to prevent compressive forces that can result in a reduction of graft volume, dehiscence, or other wound healing complications. If the use of a tissue-borne prosthesis is unavoidable, it should remain out of functional occlusion and be removed from the mouth as much as possible for the first 3 weeks.
Systemic antibiotic prophylaxis should be used for regenerative procedures involving the placement of membranes and/or graft materials, but is generally not necessary beyond the first 7 days postoperatively. Chlorhexidine rinse should be prescribed to begin 12 hours preoperatively and then locally applied to the surgical site until suture removal. Where membrane exposure occurs, local application of chlorhexidine should be done until the time of membrane removal. Patients should be instructed specifically to keep these areas clean and free of bacterial plaque and debris.
INCISION DESIGN
Conservation of blood supply to the flap overlying a GTR membrane is of paramount importance in maintaining viable, healthy soft tissues and minimizing complications. Midcrestal incisions are typically used in edentulous sites, and vertical incisions are made at least one tooth width (5 to 10 mm) from the site of the anticipated membrane margin. A tension-relieving cut-back incision, as described by Sclar, can be employed at the apical extent of vertical incisions as an alternative.
DÉBRIDEMENT
Following flap reflection, meticulous débridement of all soft tissues within and adjacent to the defect should be done with sharp curettage or piezo surgery. In extraction sockets, sharp curettage should be used to remove all remnants of the periodontal ligament. The apex of the socket should be carefully explored, and any residual periapical soft tissue should be thoroughly removed. The surrounding bone should be smooth, level, and free of projections, which would interfere with adaptation of the membrane.
DECORTICATION
Decortication is a principle used in the placement of autogenous grafts in a variety of orthopedic and maxillofacial procedures and may be done in all GBR procedures. Successful bone regeneration cannot occur without the availability of osteoprogenitor cells and an adequate blood supply. The adjacent marrow space is a source of both, and more rapid access to the endosteal compartment is believed to have the potential to enhance bone regeneration. This can be accomplished by the use of a slow-speed round bur and copious irrigation (see Case Report 25-4 , Figure 25-35 ).
The preoperative view ( Figure 25-34 ) demonstrates inadequate ridge width requiring augmentation before placement of endosseous implants. A midcrestal, full-thickness incision and reflection reveals the atrophic ridge, which is perforated with a #2 round surgical bur in preparation for augmentation ( Figure 25-35 ). A composite graft consisting of autogenous bone shavings ( Figure 25-36 ) combined with mineralized and demineralized allograft putty (Exactech, Inc. Gainesville, Fla.) is placed ( Figure 25-37 ). A type I bovine, cross-linked bovine collagen membrane (Osteogenics Biomedical, Inc. Lubbock, TX) is positioned to completely cover the graft ( Figure 25-38 ). Tension-free primary closure is achieved with dPTFE suture ( Figure 25-39 ). After 6 months, the augmented site was well healed ( Figure 25-40 ). On exposure, the graft was found to be well consolidated and endosseous implants were placed ( Figure 25-41 ). Histologic analysis of the grafted ridge revealed 43% bone by volume, 97% of which was vital bone, and 3% residual, nonvital allograft ( Figure 25-42 ). The implants were allowed to integrate for 3 months and then progressively loaded with an acrylic provisional restoration ( Figure 25-43 ).
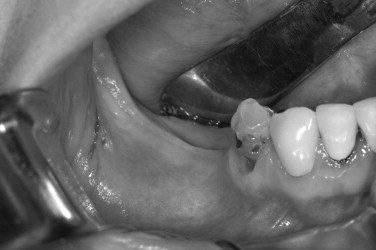
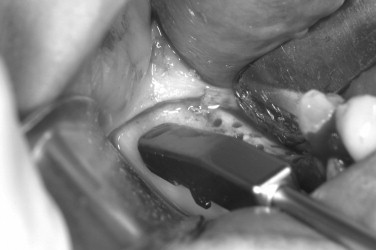
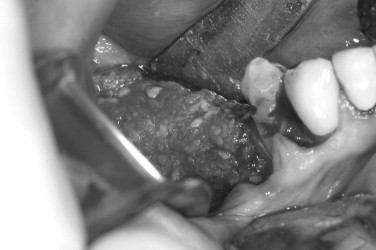
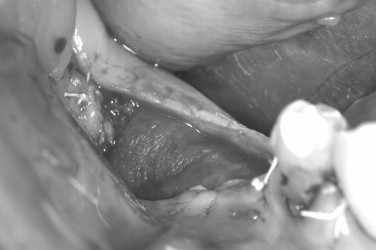
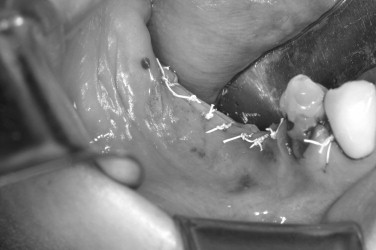
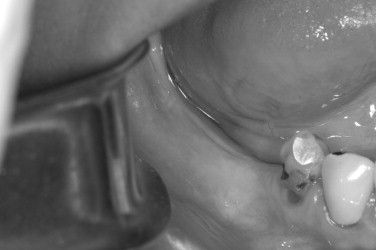
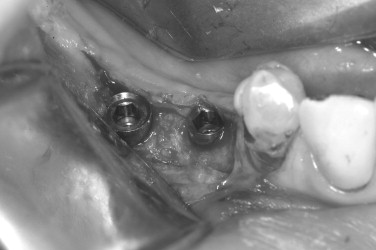
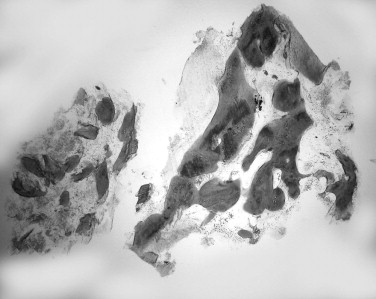
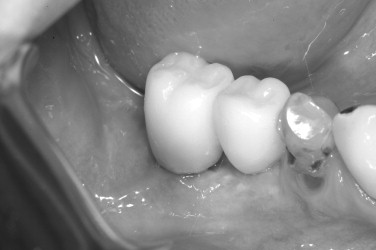
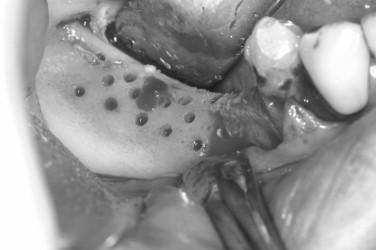
MEMBRANE TRIMMING AND PLACEMENT
When handling and trimming membranes, talc-free gloves should be used to prevent contamination of the surface. To minimize contamination of the membrane during trimming, a template made from sterile foil or surgical wrap may be used to determine the final outline. This information is then transferred to the actual membrane. Sharp corners or frayed edges on the membrane should be eliminated because this can lead to irritation or perforation of the overlying soft tissue. With titanium-reinforced membranes, avoid trimming within 2.0 mm of the titanium struts to prevent inadvertent delamination of the membrane. Properly trimmed, the membrane should completely cover and extend 3 mm to 4 mm beyond the margins of the defect. This distance is usually sufficient to provide an adequate seal between the margin of the membrane and the defect.
A notable exception, however, is when placing a membrane adjacent to natural teeth or between natural tooth roots. In this case, to prevent sloughing of the flap and loss of the interdental papilla, the membrane should be trimmed so that it is no closer than 1.0 mm to the adjacent tooth roots. In addition, avoiding contact of the membrane with the tooth root prevents percolation of oral fluids beneath the membrane, an occurrence that can lead to infection. Similarly, if placement of two membranes is required, they should not overlap in an area that may subsequently become exposed to the oral cavity, such as near the crest of the ridge.
Once the membrane has been trimmed and is in place, the surgeon should reposition the soft tissue flaps to ensure that the membrane is stable and that no wrinkles or folds occur during closure. Membranes that are soft and compliant with the overlying soft tissues may be stabilized adequately by tucking the membrane subperiosteally under the flaps, achieving stability via cell ingrowth or attachment. More rigid materials, which are sometimes required in certain cases, require stabilization with tacks or microscrews to prevent micromotion.
SPACE MAKING
Consideration must be given to creating and maintaining sufficient space under the membrane for bone regeneration to occur. The tendency for membrane collapse is related to both the morphology of the bone defect and the inherent stiffness of the membrane. Narrow, intrabony defects with three or four adjacent walls present less risk of membrane collapse than broad defects with only two or three walls. The most common method of providing additional membrane support and stability is the addition of resorbable particulate bone grafting materials under the membrane. Autogenous bone, allogenic bone, xenografts, or alloplasts may be used individually or in combination. Other methods include the use of tenting screws or perforated mesh in addition to a GTR membrane. Titanium-reinforced membranes are also available, which provide additional stability and added protection against collapse ( Figures 25-1 and 25-2 ). When using large titanium-reinforced membranes, strong consideration should be given to stabilizing these devices with bone tacks or screws.
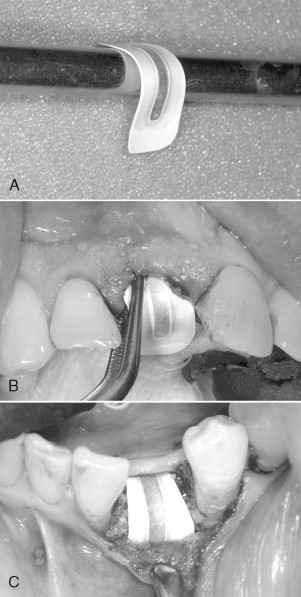
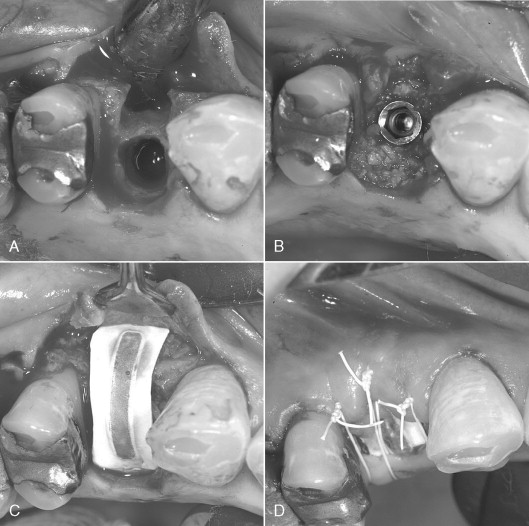
▪
CLOSURE
PROCEDURES REQUIRING PRIMARY CLOSURE
Generally, ePTFE membranes require primary closure as a result of the open surface microstructure and the risk of infection and graft failure when exposed. Collagen membranes, although more forgiving of exposure, perform more predictably when primary closure is maintained. Vertical incisions can be used to assist in achieving primary closure provided they are made remote from the position of the membrane. Horizontal periosteal releasing incisions made at the flap base, followed by tissue release via blunt dissection, can be used to ensure a tension-free primary closure. However, it should be appreciated that this technique can result in damage to blood vessels and therefore compromise the blood supply to the flap margin. A bilayered suturing technique can be employed using a combination of horizontal mattress and interrupted sutures. For closure of crestal incisions, a monofilament suture material, such as PTFE, is ideal because it will not encourage wicking of bacteria into the wound. Crestal sutures should be left in place for 10 to 14 days. Vertical incisions can be closed with fine chromic gut suture.
PROCEDURES NOT REQUIRING PRIMARY CLOSURE
dPTFE and certain high-density collagen membranes have been used successfully without intentional primary closure in a so-called open technique. The membrane in this technique can be viewed as a “second flap” to cover extraction sites, either for immediate implant placement or ridge preservation procedures. This approach can be advantageous, particularly in applications, such as anterior extraction sites, where minimal disruption of the soft tissue architecture is desirable. Because primary closure is not required, vertical incisions can be avoided, and the mucogingival junction is left in its native position. Furthermore when a flapless technique is employed, the gingival margin can be left in its native position, maximizing the width of keratinized gingiva. Because the membrane will be exposed, special precautions and slight variations in standard GBR technique are required.
When preparing for final closure in the open technique, care should be taken to ensure precise soft tissue adaptation and the development of an adequate seal at the membrane margins. There should be no exposed membrane edges, and the membrane should be carefully positioned so that it is not wrinkled or folded. If the use of two or more membranes is required, they should be arranged so that they do not overlap in an exposed region. It is imperative that no stray graft particles are left between the flap and membrane; otherwise, a channel for migration of oral fluids and bacteria under the flap can occur. This is best accomplished by using copious irrigation and suction, followed by inspection under magnification. Stability of the membrane will be dependent on the soft tissue flaps, so it is imperative that the membrane should extend a minimum of 3 to 5 mm beyond the margins of the bone defect and at least this far beyond the flap margins. Sutures are recommended to eliminate dead space and further stabilize the flap and membrane, using an interrupted, horizontal mattress, or crisscross technique. A monofilament suture is recommended and should be left in place for 14 days.
▪
POSTOPERATIVE CONSIDERATIONS
The use of removable dental prostheses is commonly associated with wound healing complications in GBR procedures. Whenever possible a tooth-supported provisional restoration should be used, either fixed or removable. If a conventional, tissue-supported, removable prosthesis is used, it should be removed from the mouth except for brief periods of use for the first 4 to 5 days of healing. It is prudent for the surgeon to generously relieve the tissue surface of the acrylic prosthesis at the time of surgery to ensure that there is no contact with the incision line or soft tissue overlying the GBR membrane. Adequate space should be provided to accommodate postoperative swelling. Patients should be instructed to use the prosthesis only for cosmetic reasons for the first 2 to 3 weeks. A soft diet should be prescribed, and patients should be advised to avoid functional loading of the surgical site until soft tissue healing has occurred. Oral hygiene should be meticulous, and local application of chlorhexidine rinse should be done until sutures are removed. If an open technique is used as described earlier, local application of chlorhexidine should be continued until the time of membrane removal.
In contrast to the relatively predictable outcomes with exposure of dPTFE, premature exposure of microporous PTFE (ePTFE) barriers can lead to complications or even failure of the procedure. Macheti, in a meta-analysis of regenerative procedures, found that exposure of microporous membranes resulted in a sixfold decrease in bone formation compared with sites where the membranes remained covered by soft tissue. Exposure of ePTFE leads to rapid surface contamination, migration of bacteria within the interstices of the material, and finally entry of bacteria into the surgical site. Small exposures do not necessarily indicate impending failure, but if infection occurs under the barrier, failure of the augmentation procedure will occur. Exposures of ePTFE should be managed expectantly with local cleansing and treatment with chlorhexidine. Weekly observation is suggested, and removal of the barrier should be done at the first sign of infection. If exposure of the edge of the barrier occurs or if there is instability or mobility of the barrier observed, the barrier should be removed at that time. Exposure of collagen membranes and subsequent colonization by oral bacteria can lead to premature degradation by bacterial proteases. However, highly cross-linked membranes and those with sufficient thickness and density are claimed to better withstand clinical exposure without graft failure.
▪
MEMBRANE SELECTION RATIONALE
Since it is often stated that bioresorbable membranes were developed to avoid a second surgical procedure for membrane removal, one might question the rationale for the continued use of nonresorbable barriers. There are at least three major indications for their use. First, nonresorbable barriers are indicated in large, non–space-making types of defects. In this instance, the use of titanium-reinforced membranes is highly desirable. Second, there may be cases where achieving primary closure is difficult and there is a high risk of incision line opening. In this case, the use of a dPTFE membrane may be advisable, allowing the clinician to “buy time” in the event of an exposure. Third, with the emphasis on noninvasive surgical techniques, sometimes the maneuvers necessary to achieve primary closure may be undesirable. In this case, one may elect to use an open technique with a high-density membrane, leaving the soft tissues in their native position.
In the nonresorbable category, there are two products available in the U.S. market: ePTFE (Gore-Tex W.L. Gore & Associates, Inc. Flagstaff, AZ) and dPTFE (Cytoplast, Osteogenics Biomedical, Inc. Lubbock, TX). Although chemically identical, the two products differ primarily in density and pore size. ePTFE is a sintered material, having pores in the size range of 8 to 30 μm. dPTFE is a nonsintered material, having a nominal pore size less than 0.2 μm ( Figures 25-3 and 25-4 ).
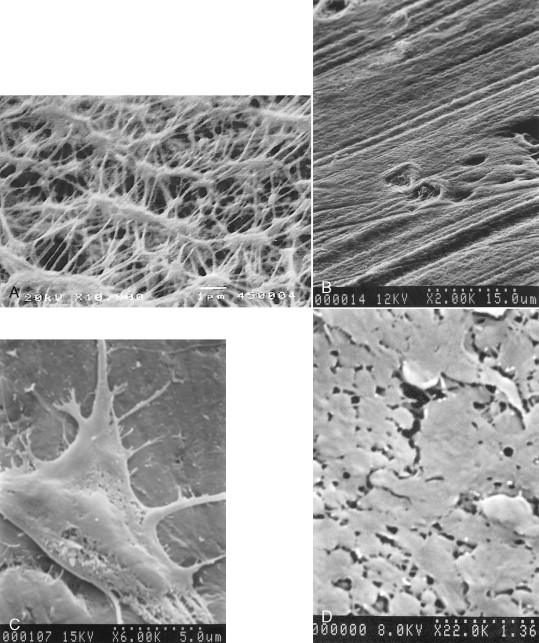
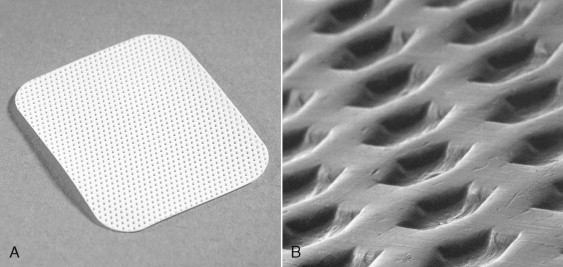
In the resorbable category, there are a wide variety of products available, differing substantially in terms of resorption time and material density. Collagen may be derived from human, bovine, and porcine sources. Bovine collagen products are manufactured from reconstituted dermis or Achilles tendon. The raw material is ground, hydrolyzed in weak organic acid, and then dispersed in an aqueous solution. The solution is further purified to remove noncollagenous protein, lyophilized, and then formed into the final product ( Figure 25-5 ). Various techniques are used to cross-link the collagen to achieve biologic stability and a clinically useful resorption profile. Chemical cross-linking methods include glycosylation and exposure to formaldehyde, glutaraldehyde, hexamethylene diisocyanate (HMDC) cyanamide, 1-ethyl-3-(3dimethylaminopropyl) carbodiimide (EDC), hydrazine, or diphenylphosphoryl azide (DPPA). Nonchemical methods include the use of ultraviolet radiation and dehydrothermal cross-linking.
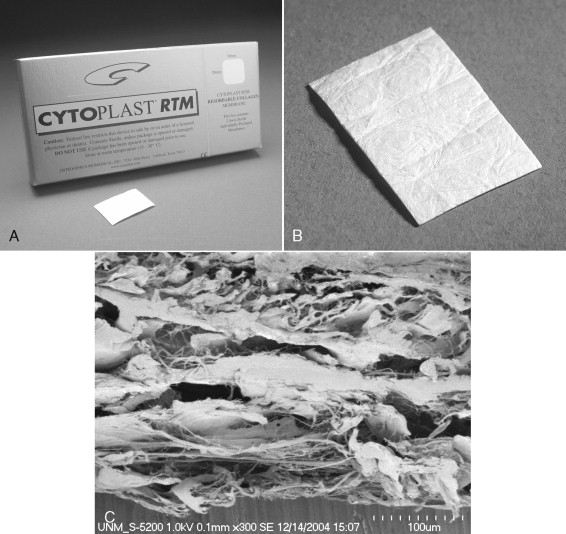
GTR membranes manufactured from native collagen are also available. One such product is manufactured from porcine dermis (Bio-Gide, Osteohealth Co. Shirley, N.Y.), resulting in a bilayered material with both a cell occlusive and porous layer. A similar approach using acellular processed human dermis results in a dense collagen membrane, relying on native cross-links for stability (Alloderm, LifeCell Inc. Branchburg, NJ). A related example is a product manufactured from thin sheets of demineralized human cortical bone (Lambone Pacific Coast Tissue Bank, Los Angeles), which relies on the native collagen matrix for strength and stability.
As a result of a desire to have alternatives to the use of animal- and human-derived products, synthetic bioresorb-able membranes have been introduced. Currently the poly(hydroxyortho esters), such as polylactic acid (PLA), polyglycolic acid (PGA), and their copolymers, are most commonly used. These biomaterials have a long history of use, primarily as suture materials and surgical mesh.
Specific biomechanical and resorption characteristics can be engineered into these products by varying the ratio of PLA : PGA in the final product. PGA is the weaker of the two biomechanically, more hydrophilic, more flexible, and exhibits a more rapid degradation rate. PLA is strong biomechanically, more hydrophobic, more brittle, and has a very long degradation rate. The inherent stiffness of these materials is overcome either by using a mesh-type structure, by the addition of a plasticizing agent, or by the addition of a third polymer.
In vivo degradation of these materials occurs through hydrolysis of the polymer backbone into organic acids and esters, which ultimately are metabolically eliminated as CO 2
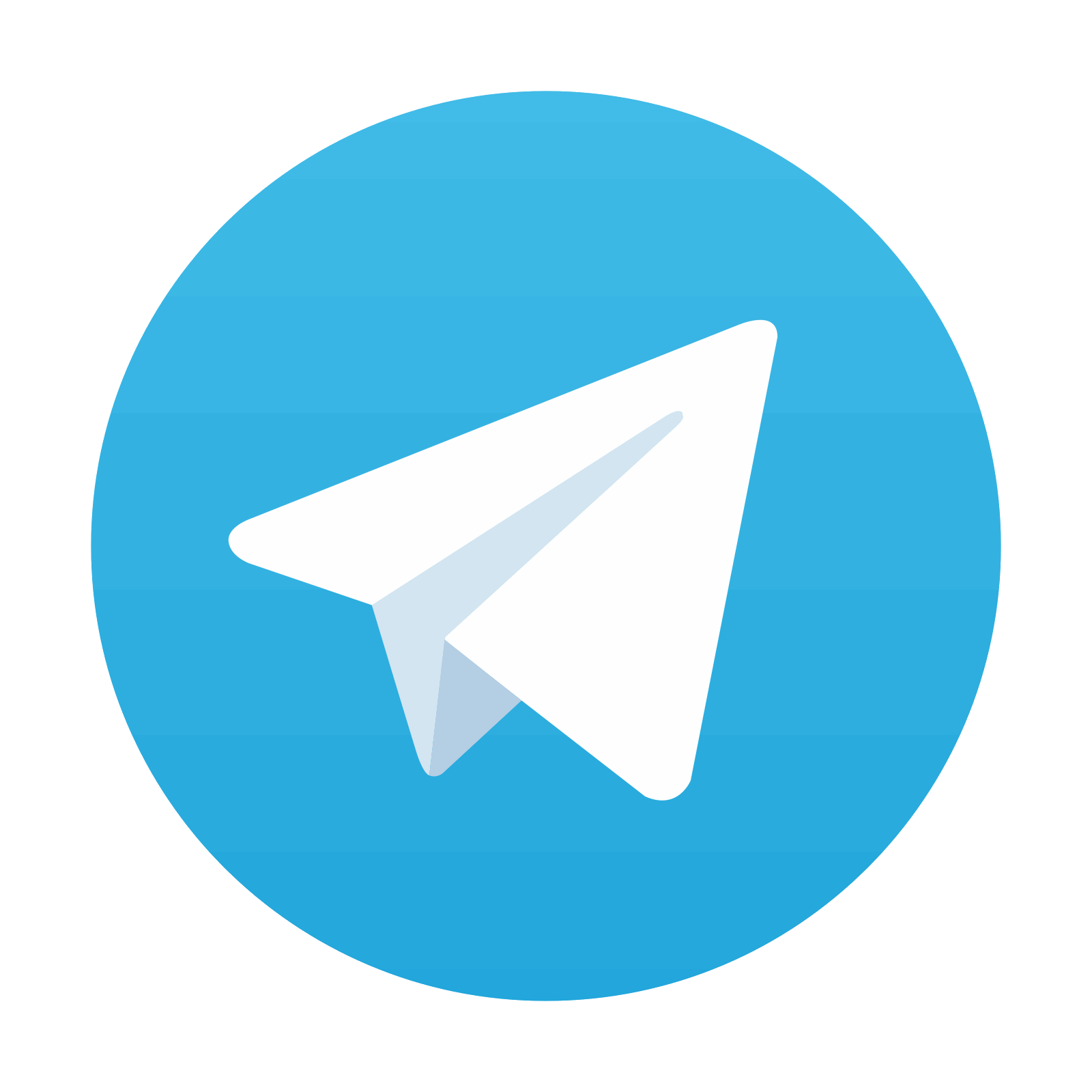
Stay updated, free dental videos. Join our Telegram channel

VIDEdental - Online dental courses
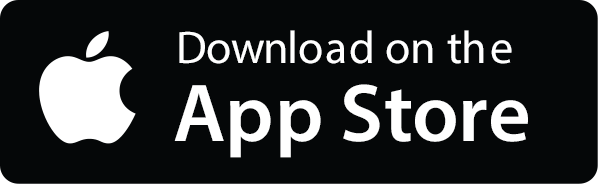
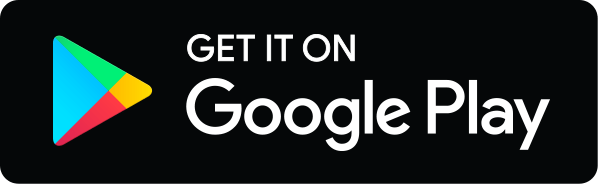