Gene Therapy
Gene therapy was recently reviewed by Baum [30]. With the above described knowledge, the group of Baum hypothesized that since irradiated glands are devoid of functioning acinar cells, the remaining duct cells could be changed in function to allow fluid secretion. To achieve this it was proposed to generate an osmotic lumen with a large interstitium gradient over duct cells facilitating water permeability. A serotype 5, adenoviral (Ad5) vector encoding the human water channel protein aquaporin-1 (hAQP1) was constructed. Human aquaporin-1 can facilitate the extremely rapid movement of water over cell layers. Expression of this protein in cells, normally not transporting water, can lead to increases in osmotic gradient-driven water movement. First, retrograde Stensen’s duct injection of the AdhAQP1 in rats [31] and minipigs [32] was tested. In both species the dramatically radiation-induced reduction in salivary fluid secretion was restored to almost normal levels, albeit transiently. Indeed, the hAQP1 transgene was expressed only in duct cells, implicating that the increased salivary secretion observed is most likely due to enhanced water permeability in the normally water-impermeable duct cells [32, 33]. Next a large toxicity study in rats was performed which showed that retrograde ductal delivery in submandibular glands of AdhAQP1 to salivary glands appears to induce at most some early toxicity [33]. Now very recently [34], a phase I study was performed to determine whether hAQP1 gene transfer is safe and effective in humans (http://www.clinicaltrials.gov/ct/show/NCT00372320). This trial was performed in individuals previously treated for HNC with salivary hypofunction more than 5 years after radiotherapy. No deaths or serious dose-limiting toxicities and only few mild to moderate adverse events were observed. Most importantly, in about half of the patients, objective responses were seen with even a subjective improvement in xerostomia. Therefore, AdhAQP1 vector delivery to a single parotid gland was considered safe and effective in a subset of subjects [34]. Permanent gene transfer and the use of less immunogenic vectors, however, will be necessary to induce long-lived expression of hAQP1 in salivary glands. Therefore, further studies for the use of gene therapy to relieve xerostomia patients from their burden are warranted.
Prevention
Radioprotectors
Many approaches have been pursued to protect normal tissues and specifically the salivary gland from radiation damage. The most obvious one is the reduction of the physical dose to the tissue, as radiation exerts its effect through the generation of free electrons and free radicals, which subsequently damage cellular proteins. Of these cellular proteins, the DNA and, as mentioned above, the membranes are the most important targets, inducing cell death of cell dysfunction [15]. For years radical scavengers have been tested for their efficacy to reduce damage to normal tissues. Radical scavengers oxidize free radicals and peroxides, thereby reducing the radiation dose effect. However, when radical scavengers are being used during cancer treatment, a clear difference should be demonstrated between the protection of the tissue in question and the tumour showing an actual improved therapeutic ratio.
A potential interesting scavenger is Tempol. In a mouse head and neck radiation model, it was shown that Tempol reduced irradiation-induced salivary gland hypofunction [35] without protection of tumour tissue [35]. However, no clear therapeutic ratio has been determined and no clinical data are available yet [36].
More extensively studied is the oxygen radical scavenger amifostine (WR-2721 or Ethyol). Murine studies showed a high and rapid accumulation of amifostine in a selective number of healthy tissues among which the salivary glands [37] and a poor and slow accumulation in tumours [38]. Several animal studies showed radioprotection of parotid glands’ function and structure [39]. Currently, amifostine is not widely used due to some toxicity, price and an unclear therapeutic ratio. However, depending on the type of cancer treatment, symptoms can be reduced to some degree [40]. Interestingly, the protective ability of amifostine strongly depends on the irradiated glandular region and is observed for later damage only. The major effect of the drug seems to be the prevention of volume effects caused by secondary damage occurring in shielded parts of the gland [39].
Three decades ago, the acute response of salivary glands to radiation was hypothesized to be due to damage to the granulas inside the acinar cells. These membrane-enclosed organelles are rich in heavy metals (Zn, Mn and Fe) and could be subjected to radiation-induced lipid peroxidation, evoked by a metal catalysation process. As a consequence granulas could leak proteolytic enzymes that would evoke immediate cell lysis and subsequent loss of granula-containing cells [16]. Degranulation prior to irradiation would therefore protect the salivary gland [16, 17]. Although this is an attractive explanation for the acute response and offers means to protect the salivary gland from radiation, it was found not to be the sole cause. Proteolytic enzymes are exclusively present in GCT cells and can therefore not explain protective effects on acinar cells, and actually no significant cell lysis (i.e. cell loss) was observed in rat glands after clinical relevant doses [41]. Interestingly, also drugs that stimulated saliva secretion without degranulation protected against radiation-induced damage [42, 43]. Moreover, recently it was suggested that this at least in part is due to compensatory mechanisms through increased proliferation of undamaged cells.
Stimulation of Regeneration
Many factors play a role in the response of a tissue to radiation [44]; however, the onset and the severity of the radiation effects are ultimately determined by the (in)ability of stem/progenitor cells to reconstitute functional cells [18, 45]. Recovery and compensatory responses in non-irradiated regions presumably containing stem/progenitor cells have been observed after radiation, indicating the potential of surviving stem/progenitor cells to regenerate the tissue [15, 46].
In every tissue, homoeostasis is maintained by proliferation and differentiation of the tissues’s progenitor/stem cells compensating for cell loss due to aging or cytotoxic insults. Progenitor cells are often responsible for the bulk of proliferation and regeneration, whereas the stem cell becomes only active after substantial damage. In the adult salivary gland, nearly all of the differentiated cell types seem to retain the ability to replicate [22, 47]. Although it is still not completely clear what the exact stem and progenitor cells of the salivary gland are, it has been proposed that the intercalated ducts contain progenitor cells [11, 23] and the excretory ducts harbour the stem cells [24, 48, 49]. Immediately after irradiation, all proliferation ceased, where after 3 days of compensatory proliferation is started in the intercalated duct compartment [50]. However, after some months, hardly any proliferation is detected anymore [51], indicative of a strong reduction in regenerative capacity. Strangely, when taken out of the environment, salivary gland stem cells can still be cultured in vitro [52] indicating that radiation-induced anti-proliferative signals are being produced within the irradiated gland. Stimulating the proliferative process of the remaining stem/progenitor cells in the salivary gland (as described above) seems to at least induce some regenerative response. Indeed, stimulation of proliferation and differentiation of radiation surviving stem cells have been shown to be beneficial in salivary glands. Cytokines like EGF [53, 54], insulin growth factor [54, 55] and bFGF [55] have been suggested not only to enhance proliferation, but also inhibit apoptosis. Especially for keratinocyte growth factor (KGF or FGF7), induction of proliferation and expansion of stem/progenitor cells have been suggested to be the mechanism of reduction of radiation-induced salivary gland damage in the mouse submandibular gland [52]. ΔN23-KGF treatment for 4 days prior to irradiation was shown to induce general proliferation including stem/progenitor cells, increasing the stem and progenitor cell pool. As such the absolute higher number of stem/progenitor cells and acinar cells that survived irradiation increased, although the relative radiation sensitivity of the stem/progenitor cells was not affected. Post-irradiation treatment with ΔN23-KGF accelerated the expansion of the pool of progenitor/stem cells that survived the irradiation treatment to further improve gland function [52].
Another cytokine pathway is that of Wnt/β-catenin known to be essential for the maintenance and activation of various adult stem cells. Transient over-expression of Wnt1 in basal epithelia in a Wnt1 transgenic mice activated Wnt/β-catenin pathway in submandibular glands of male mice. This prevented radiation-induced salivary gland dysfunction potentially through the inhibition of apoptosis in stem cells [56], allowing enhanced regeneration. Also, basic fibroblast growth factor (bFGF) was shown to prevent salivary gland dysfunction after irradiation. Again, this effect was attributed to the inhibition of radiation-induced apoptosis in the secretory salivary gland tissue and the paracrine effect of this factor on these tissues [57]. Next to this signalling molecules like roscovitine, which inhibit cyclin-dependent kinase and acts to transiently inhibit cell cycle progression allowing improved DNA repair and suppress apoptosis, has potential to reduce radiation-induced hyposalivation [58].
All these cytokines, growth factors or signalling molecules clearly show some beneficial effect increasing salivary flow after irradiation, either through inhibition of apoptosis or enhanced proliferation of progenitor and/or stem cells. However, whether or not these substances ever make it to the clinic is rather unsure, as potential inhibition of cell death or induction of proliferation of (remaining) tumour cells is a big drawback. First, increases in therapeutic ratio should be shown before such substances can be used. The use of these substances ex vivo, however, could yield promises.
Mesenchymal Stem Cells
Many attempts have been made to use multipotent stem cells derived from several tissues to induce regeneration of damage salivary glands. Long-term adherent cultures of cells derived from the human submandibular gland have been established [59]. These cells could undergo chondrogenic, osteogenic and adipogenic differentiation, hallmarks for mesenchymal stem cells (MSCs). Although these salivary gland-derived MSCs were shown to be supportive in the regeneration, differentiation into all salivary gland lineages was not shown [47]. Mesenchymal cells from other tissues may also have therapeutic potential for salivary gland disorders. Bone marrow MSCs have been suggested to be able to differentiate into myoepithelial cells or exert a supportive action through the secretion of growth factors and immunosuppression [60]. As such these supportive salivary gland MSCs may offer therapeutic potential during recovery and regeneration of damaged salivary glands [47]. Indeed, allogeneic bone marrow-derived MSCs were shown to suppress autoimmunity and restore salivary gland secretory function in both mouse models of Sjögren syndrome (SS) [61] and SS patients [61, 62]. Interestingly, the soluble intracellular content of lysed whole BM cells was shown to be advantageous in the repair of irradiation-damaged salivary glands [63], indicating that indeed probably the supportive action of secreted components is inducing the regeneration and not major transdifferentiation into salivary gland lineages [47]. Regretfully, still viable stem and progenitor cells are necessary for regeneration, since complete sterilization of the progenitor/stem cells of the salivary gland with higher radiation doses abrogates the supportive action of bone marrow-derived cells [29, 64] (Fig. 13.2).
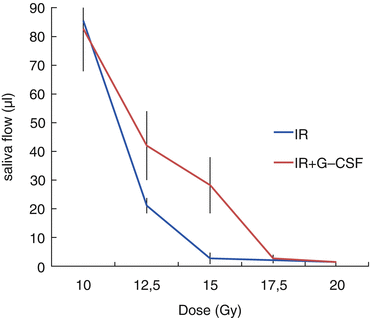
Fig. 13.2
Window of effect of G-CSF on saliva flow 30 days following different radiation doses (Adapted from [29])
Stem Cell Therapy
The field of stem cell research has made a huge progress in the last decades indicating exciting possibilities for stem cell-based therapies for regeneration of tissues. Stem cells play a pivotal role in the response of salivary glands to radiotherapy as they play an important role in tissue repair [47]. With the discovery and experimental handling of many different types of stem cells in general, stem cell-based therapies may in the near future be used to protect, accelerate or enhance salivary gland regeneration. A prerequisite is however that the stem cells potentially used for therapy are shown to be able to self-renew (produce more stem cells) and produce more committed progenitor or functional salivary gland cells (differentiation) (Fig. 13.3).
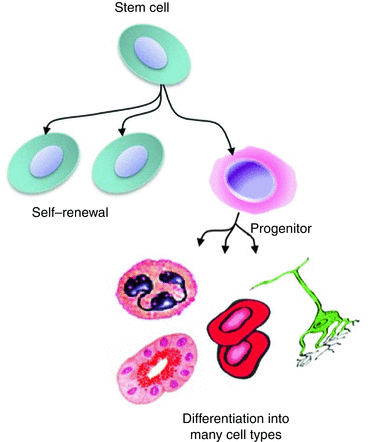
Fig. 13.3
Tissue stem cells are able to self-renew and differentiate in precursors and functional cells
Three different types of stem cells exist; embryonic stem (ES) cells, induced pluripotent stem (iPS) cells and adult stem cells (ASCs). ES cells, derived from the inner cells mass of blastocysts, can easily be cultured for experimental purposes and can form all tissues of the body. In 2006 [65], 2012 Nobel Prize winner Shinya Yamanaka’s team at Kyoto University, Japan, managed to culture ES cell-like iPS cells from adult somatic cells, such as fibroblasts, by introduction of pluripotency genes like the transcription factors Oct-3/4, SOX2, c-Myc and Klf4. Similarly to ES cells, these cells can indefinitely self-renew, are pluripotent and able to produce all cell types of the body after injection into blastocysts. iPS cells hold high promises for fundamental and therapeutic applications. Adult stem cells are a population of undifferentiated cells that are maintained through development and into adulthood. Such ASCs are believed to be present in every tissue or organ in the body and are committed to that specific tissue type to repair damage.
So far to the best of our knowledge, no salivary gland cells have been derived from iPS cells nor have iPS cells been transplanted into salivary glands. Murine early ES cells, however, seem to differentiate in three-dimensional structures of the salivary glands after co-culturing with human salivary gland-derived fibroblasts and display some neogenetic ability after transplantation [66]. Regretfully, both ES cells and iPS cells are prone to develop teratoma’s and are not ready to use for stem cell therapy until fully differentiated into salivary gland stem/progenitor or differentiated cells. Therefore, for the purpose of stem cell therapy, the focus lies mostly on the ASCs. Currently, salivary gland stem cells have not been fully characterized but some promising results have been obtained so far (reviewed in [49]).
Although adult stem cell therapy for the hematopoietic system has been in use already for many decades, for other tissues, such a therapy is only in its infancies. Several groups have been working on salivary gland stem cells and the potential application in therapy (see, e.g., [47, 49, 67, 68]).
Label retaining, stem cell marker and duct ligation studies (see [49]) have conclusively indicated that the tissues’ stem progenitor cell resides in the major ducts of the salivary gland. ASC Isolation prior to radiotherapy culturing during and transplantation after radiotherapy, similar to the bone marrow transplantation protocols could restore tissue homoeostasis and regenerative potential. However, bone marrow-derived stem cells are relatively easy to obtain, whereas ASCs are mostly invisibly integrated in the tissue. Therefore, several techniques have been developed to obtain putative murine salivary gland stem cells. One option is to mince and enzymatically digest the tissue and subsequently use stem cell markers to select the ASCs, either directly after digestion or after some days of culturing [69]. The latter method is based on the idea that only stem progenitor cells are able to proliferate extensively and form spheres (named salispheres) that consist of many cells [48]. From the tissue material and the spheres, several cell types have been selected that have shown to have at least some potential to self-renew and differentiate either in vitro or in vivo (see [49]). As such cells expressing CD24, CD29, CD49f, CD117, ALDH, Sca-1 and Ascl-3 have been isolated from rodent and CD29, CD34, CD49f, CD90, CD117, CD166 and ALDH from human salivary glands. Cells expressing these markers have shown at least some in vitro differentiation potential [49]. Regretfully, only view studies have shown functional restoration after transplantation of marker expressing cells.
In our laboratory, we developed culture method, which allows the growth of salispheres from mouse and human salivary glands [48, 70]. Interestingly, salisphere-derived cells were able to self-renew in vitro and differentiate into salivary gland lineages suggesting that they encompass ASCs. We also showed that murine salispheres express adult stem cell marker proteins CD117, CD24, CD29, CD49f, Sca-1, Mushashi-1, CD44, CD90 and CD34, all of which could be localized to ducts of naïve SGs [49]. Of these, especially CD117 cells were shown to be able to reconstitute homoeostasis in murine salivary gland after irradiation [48, 71]. Interestingly, only 300 CD117+ cells induced recovery to 70 % of the original saliva flow. Cells expressing CD24, CD29, CD49f and potentially combinations of these markers have also shown promising regeneration effects, albeit only after injection of higher cell numbers [72]. Importantly, the transplanted ASCs had functionally integrated within the secretory tissue of the recipient gland, expressed donor-derived markers and displayed ductal and acinar cell-type morphologies.
Cells derived from human salispheres also have shown to express multiple stem cell markers and have been shown to be able to self-renew and differentiate in vitro [70]. The most potent stem cell containing populations however still need to be determined.
The human salivary gland may harbour stem cells that are similar to the mouse; however, it is plausible that they will differ. Potentially it may be better to start with a trial in which all the cells from human salispheres are transplanted. This will contain a cocktail of stem and progenitor cells and may provide optimal gland recovery, otherwise inhibited by the relatively long tissue turnover time. As such, progenitor cells induce short-term recovery, whereas the stem cells take care of the long-term maintenance and homoeostasis. The exact number of stem cells necessary for salivary gland rescue will depend on the patient age, extent of irradiation and potentially on use of medication. Since the stem and progenitor cells seem to reside in the major ducts, this may be the best place to inject the stem cells.
But first the salivary gland culture should be further optimized and translated for compliance to current good manufacturing practice (GMP) regulations, e.g. using MACS- and cGMP-approved antibodies [73]. Furthermore, the culture period should be followed by cryopreservation until the radiotherapy is finished and the patient is ready for transplantation. HNC patients are mostly older, and old age has been suggested to lead to an even more dramatically response to radiation [74]. A reduced salisphere-forming capability of cells from salivary glands of old mice was observed [70] which when combined with a small salivary gland biopsy that may be obtained prior to the radiotherapy makes it probably necessary to increase the number of ASCs before transplantation. It is of eminent importance to find protocols that safely allow this. Recent in vitro culture, self-renewal and differentiation methods for ASCs may be used for screening of novel factors useful for amplification. Adult stem cell therapy in patients after radiotherapy may dramatically reduce the decline in quality of life.
Head and Neck Cancer and Radiotherapy Treatment
This sub-chapter partly contains quotations from the thesis of T.A. van de Water [75]. Radiotherapy, often applied in combination with surgery or chemotherapy, is an important treatment modality for the management of head and neck cancer. The main objective of radiotherapy is to optimize the dose to the tumour (sterilizing the tumour cells by administering a dose as high as possible) while avoiding the normal surrounding tissues as much as possible.
In head and neck cancer patients, target volumes are often complex shaped, large and surrounded by various critical and vital structures (e.g. the spinal cord, salivary glands, the hearing organ, the optic structures and structures involved in swallowing). Therefore, radiotherapy of the head and neck region is frequently associated with radiation-induced acute and late side effects that adversely affect quality of life [5, 6, 76, 77]. Hence, apart from eradication of the tumour, preservation of organ function is of major clinical importance as well.
More specifically, xerostomia or oral dryness is the most frequently reported side effect occurring after radiotherapy of the head and neck region [5, 78] and has a significant adverse effect on quality of life [5, 6]. The salivary glands that are responsible for a sufficient saliva production and composition are the parotid, submandibular and sublingual glands (the major salivary glands) and the minor salivary glands lining the oral cavity [79, 80]. Radiation results in a progressive loss of salivary gland function and therefore in a decrease in saliva output and a change in saliva composition, resulting in the sense of dry mouth and sticky saliva [10, 81]. Moreover, salivary dysfunction may result in considerable additional problems, including severe oral discomfort, impairment of oral functions (speech, chewing, swallowing) due to insufficient wetting and an increased incidence of caries and mucosal infections [10, 78].
It is important to note that the probability of radiation-induced complications markedly depends on the radiation dose to the organs at risk (OARs). Thus, by conforming the radiation dose to the target and simultaneously limiting the dose to the OARs, the severity and incidence of the radiation-induced side effects can be reduced.
Clinical Studies
Over the years, radiotherapy treatment techniques have been improved and allow better conformation of the high-dose region to the PTV, while OARs can be spared more adequately.
Clinical studies indicated that compared with more conventional radiotherapy techniques, intensity-modulated radiotherapy with photons (IMRT) significantly reduces the parotid gland dose, resulting in higher flow rates after treatment and/or lower rates of xerostomia contributing to an improved quality of life [82–84] (also see Chap. 10
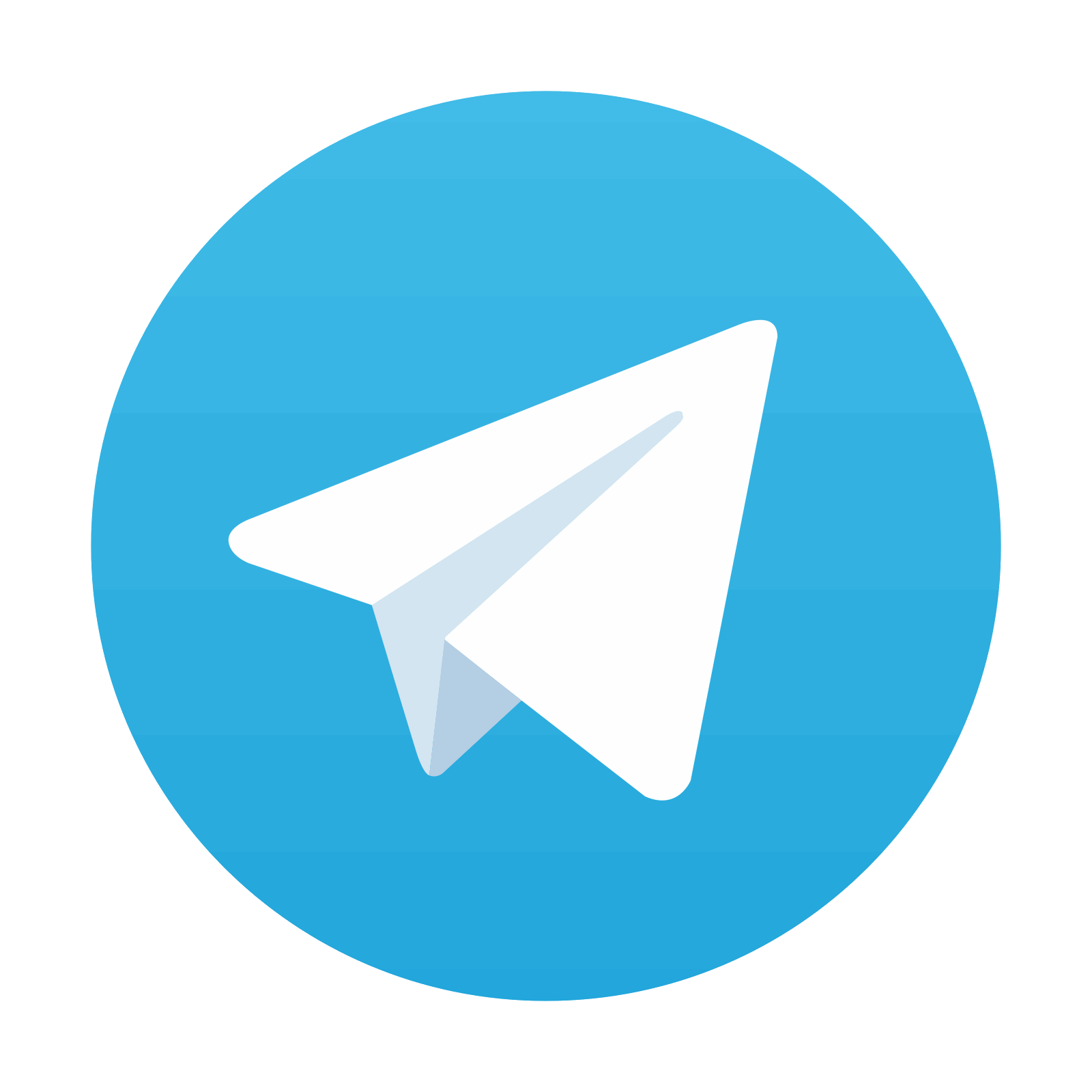
Stay updated, free dental videos. Join our Telegram channel

VIDEdental - Online dental courses
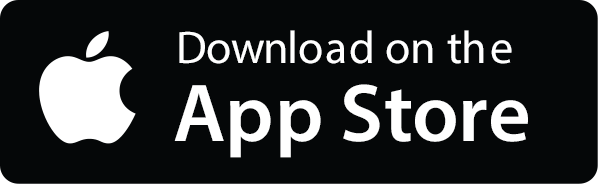
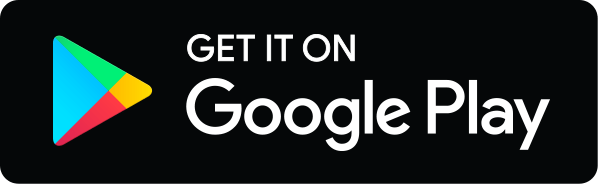