© Springer-Verlag Berlin Heidelberg 2009
Margaritis Z. PimenidisThe Neurobiology of Orthodontics10.1007/978-3-642-00396-7_2
2. Functional and Dysfunctional Aspects of the Cerebral Cortex
(1)
Marathonos Street 22, 152 33 Halandri, Athens, Greece
2.1 Introduction
Diagnosis of oral motor disorders is a professional skill. Most orthodontists and other specialist dentists are familiar with the many types of oral motor behaviors and their dysfunctions, such as normal chewing, speech, improper bites, malocclusions of the teeth, and oral–facial imbalances, but have perhaps not thought too much about the underlying processes or mechanisms that regulate these behaviors and which may eventually provide practitioners with a rationale for correcting dysfunction. Thus, special interest is focused on the information–input–processing function of the brain that is necessary for giving a conscious experience and how it may be related to oral motor behaviors that underlie the structure of the mouth.
In this context, the basic goals of oral practitioners are to discover the patient’s motor problem, understand the potential causes of the problem, the ways the central nervous system regulates motor behavior, and to recommend appropriate treatment. To accomplish these goals the diagnostician must have a basic knowledge of normal and disordered oral motor behavior. Since the clinicians must mediate between the patient and the dysfunction when providing corrective experience it is important that they can conceptualize the nature of oral motor behavior.
The information processing approach to motor control and learning provides a conceptual framework in which to study and understand oral motor behavior, for instance during chewing and swallowing, within the control of a mouth system with ever-changing behavior.
2.2 The Sensorimotor Cortex
The somesthetic cortex or neocortex (excluding the limbic cortex) integrates information received from the receptors throughout the body, including the mouth. The somesthetic cortex (postcentral gyrus, Brodmann’s areas 1, 2, and 3) is organized vertically. Columns of neurons (module), from one to several neurons, are oriented vertically to the cortical surface. Many vertical cylinders of neurons constitute this area. The column of neurons or module in the somesthetic cortex of the postcentral gyrus is the basic neuron circuit that in its elemental form comprises input channels (afferent fibers), complex neuronal interactions in the module, and output channels. The output channels are largely the axons of the pyramidal cells of the motor cortex of the precentral gyrus, which directly or indirectly through the pyramidal and extrapyramidal tracks, respectively, innervate the alpha motor neurons of skeletal muscles, including the trigeminal system. The postcentral gyrus, however, also has a role in motor activity, since the electrical stimulation of the gyrus may elicit movement at times. Similarly, the precentral gyrus is also a “primary sensory cortex” because it also receives input from the ventral posterior thalamic nucleus as does the postcentral gyrus [36].
The cerebral cortex is also organized into six layers or laminae of cells, arranged parallel to the surface of the brain, each of which contains different cell types that play different roles. Beginning at the cortical surface the layers are: (1) the plexiform layer or molecular layer, (2) the external granular layer or layer of small pyramidal cells, (3) the layer of medium-sized and large pyramidal cells or external pyramidal layer, (4) the internal granular layer or layer of small stellate and pyramidal cells, (5) the inner or deep layer of large pyramidal cells, and (6) the spindle cell layer or layers of fusiform cells or multiform layer [36].
Pyramidal cells of layer III project to other cortical regions (corticocortical connections), whereas pyramidal cells of layer V project to subcortical structures conveying motor commands to muscles. Layer V pyramidal cells are the largest. Layer III pyramidal cells are generally smaller. Nonpyramidal local circuit cells, which do not project out of the immediate cortical area, are smaller and more numerous than pyramidal cells. Some of these local circuit cells can be excitatory local relays, and others mediate local inhibition. One type of local circuit cell, the horizontal cell, interconnects cells across the module and is generally inhibitory. Minicolumns and modules are organized around the large pyramidal cells. The many excitatory local circuit cells relay information up and down the vertical column. In this manner, the large dendrite tree of pyramidal cells receives many inputs via local circuit cells [15].
Pyramidal cells receive many of their inputs onto dendrite spines. Spines are appendages of dendrites that increase their surface area, and compartmentalize the molecular machinery relevant to receiving inputs. As the cortical hierarchy increases, pyramidal cells receive more inputs. For example, pyramidal cells in the prefrontal cortex have 23 times more spines (and inputs) than the pyramidal cells in the primary visual cortex [15].
2.3 Oral Sensorimotor Integration
Oral sensory integration is a child’s ability to feel, understand, and organize sensory information from his/her mouth. Sensations flow into the brain through oral experiences, and the brain locates, sorts, and orders sensory stimuli into sensations. When the stimuli flow in a well-organized or integrated manner (patterned input) the brain can use those sensory data to integrate functions underlying sensation, perception, learning, memory, motor control of muscles and mentation. The connection between the capacity of an individual to integrate sensory data and the child’s social and emotional development should not be underestimated. How a child integrates information through the sensory system provides a basis of the child’s oral and body reality. When the flow of sensory stimuli is disorganized, life can be like a rush hour traffic jam. It is sensory integration that attempts to “put it all together” and that helps us make sense of who we are and understand the world around [78, 92].
The integration of oral sensory and motor functions or behavior depends on the development of reflex circuits connecting the sense organs through the central nervous system to muscles. The sensorimotor integration function of the mouth can then be analyzed in terms of three basic functions:
1.
An input transducer function. This includes all the sophisticated sensory receptors and their innervating sensory neurons, which are endowed with specificity, that is to say are able to transform definite kinds of external stimuli into complex electrical and chemical phenomena, depending on the nature and strength of the stimulus, and convey patterned action potentials (coded information) from the senses to the somesthetic cortex.
2.
A control function carried through the central processing unit, the cerebral cortex, namely the postcentral and precentral gyri, which represent the primary somesthetic and motor cortex. The capacity to distinguish one sensory experience from another in the cortex is known as discrimination. Specifically, the cortical neurons must be capable of decoding the frequency of the action potential of each nerve transmitted to the brain in order to interpret the sensory messages of the receptors, and to store in memory information of the learned particular sensorimotor reflex experience.
3.
An output transducer function. The human brain processes information in countless ways, most of which are poorly understood, if at all. When, however, there is stimulation of the oral motor areas in the cortex, movement of the mandible and tongue may result as a result of activation of trigeminal and hypoglossal motor nuclei, respectively [92].
By definition these three basic functions with relationships among them form a system, the oral sensorimotor integration system. There is no direct link between the oral input and output in the system. This means that the oral sensorimotor integration reflex function is an open loop. The motor output, however, has an influence on the sensory input. That is the movements of the mouth stimulate the sensory receptor input that guides oral function. Thus, the mouth’s sensory experiences are generated principally by its own actions, and its actions are responsive to its sensory experiences [43, 65]. This means that the oral sensory system is functionally integrated with the oral musculature.
Similarly the receptors of the skin of the oral–facial region are functionally integrated with the underlying muscles of facial expression. The contraction of muscles stimulates the receptors through deformation of the skin [78, 93]. Granit [44] suggested that adjacent portions of the stimulated skin may be inhibitory to muscle action. This function may be locally regulated without reaching conscious states in the cortex. Thus, the skin has also established “local signs” for reflex action, and not only for conscious perception. This may imply that in certain circumstances the movements of the mouth may be initiated locally without reaching perception in the cerebral cortex. In this view, the oral mucosa, like the skin, may be able to deliver both general and localized messages. The general piece of information may be based on very large receptive fields, in which the afferent fibers overlap. The localized messages may be based on smaller receptive fields down to punctiform, which are innervated by a single afferent fiber and from which a certain type of reflex response could be elicited. The central nervous system may correspondingly be organized to take care of large receptive fields, reaching the conscious level, and others of small receptive fields for local function at the brainstem level [44].
The strength of information processing performed by a cortical circuit depends on the number of interneuronal connections or synapses. Morphologically speaking, a typical neuron presents three functional domains. These are the cell body or “soma” containing the nucleus and all major cytoplasmic organelles, a single axon, which extends away from the soma and has cable-like properties, and a variable number of dendrites, varying in shape and complexity, which emanate and ramify from the soma. The axonal terminal region, which connects via synapses to other neurons, displays a wide range of morphological specializations depending on the target area [3].
Turning morphology into a functional event, the soma of neurons and the dendritic tree are the major domains of receptive (synaptic) inputs. Thus both the dendritic arborization and the axonal ramifications confer a high level of subcellular specificity in the localization of particular synaptic contacts on a given neuron. The extent to which a neuron may be interconnected largely depends on the three-dimensional spreading of the dendritic tree [3].
Dendrites are the principal element responsible both for synaptic integration and for the changes in synaptic strengths that take place as a function of neuronal activity [46, 47]. While dendrites are the principal sites for excitatory synaptic input, little is known about their function. The activity patterns inherent to the dendritic tree-like structures, such as in integration of synaptic inputs, are likely based on the wide diversity of shapes and sizes of the dendritic arborizations [48]. The size and complexity of dendritic trees increase during development [49], which has been, in turn, associated with the ability of the neural system to organize and process information, such as when animals are reared in a complex sensory environment [50]. Thus, dendritic size and branching patterns are important features of normal brain development and function.
Most synapses, either excitatory or inhibitory, terminate on dendrites, so it has long been assumed that dendrites somehow integrate the numerous inputs to produce single electrical outputs [3]. It is increasingly clear that the morphological functional properties of dendrites are central to their integrative function [51, 52]. Branching in this context is essential to the increasingly complex ability of the neuron to respond to developmental complexity [48]. The dendritic cytoskeleton plays a central role in the process of ramification, filopodia formation, and more specialized neuronal activity such as long-term potentiation circuit dynamics, including memory formation, and the response to pathological conditions [3].
Synaptic contacts take place in dendritic spines, which are small sac-like organelles projecting from the dendritic trunk. Dendritic spines are more abundant in highly arborized cells such as pyramidal neurons and scarcer in smaller dendritic tree interneurons with few interconnections. The number of excitatory inputs can be linearly correlated with the number of spines present in the dendrite [3]. How information is processed in a dendritic spine, is still a matter of current study [6]. Dendritic spines contain ribosomes and cytoskeletal structures, including actin, and a- and b-tubulin protein. As in other parts of the neuronal structure, cytoskeletal components are highly relevant in the structure and function of the neuron. The axonal hillock contains large “parallel” bundles of microtubules. Axons are also located with other cytoskeletal structures, such as neurofilaments, which are more abundant in axons than in dendrites. The axon hillock is the region where the action potential is generated. The dynamics in cytoskeletal structures are central to our contention that information can be processed and “delivered” to the synaptic function by changes in the cytoskeletal structures [3].
The recently advanced new learning and memory model of Penrose and Hameroff [113] suggests that information processing and storage of memory occurs in the microtubules and microtubule-associated proteins of the subsynaptic zone of dendrites, which gives rise to the spine. Microtubules in the subsynaptic zone are capable of storing important information concerning where spines were originally located, and of using that information to determine where synapses will reappear following learning or enriched experiences [39–41].
The primary receptive area of the cortex integrates the more complex aspects of touch, deep sensibility, pain, and temperature. These perceptions include the appreciation of the location and position of a body part (proprioception), the localization of the source of pain, temperature, and tactile stimuli, as well as the comparison of these sensed modalities with those formerly experienced through memory [36]. Ablation of the postcentral gyrus is followed by loss of the finer and more subtle aspects of sensory awareness. For example, when an object is handled with the eyes closed, the subject can feel it but cannot appreciate its texture, estimate its weight or temperature. Difficulty is also experienced in appreciating the position of the body or body parts in space [36].
The sense of taste is claimed to be located at the base of the precentral and postcentral gyri, slightly rostral to the primary somesthetic cortex or in the cortex immediately adjoining the insula area [42].
In sum, as we view the development and function of the mature mouth we are more aware that the mouth is essentially an information-processing system, in which the higher faculties of the brain, namely learning, memory, motor control of muscles and reasoning, are chiefly concerned with the choices involved in getting food, and the development of the computational activities of mastication, speech, taste, etc, through learning and memory of the oral sensorimotor integration function of the cerebral cortex. Any animal would starve to death if the effect of food was limited to its action on the surface of the mouth. Thus, the enormous significance of feeding acquired by experience and learning becomes evident.
2.4 Sensory Integration Dysfunction
All the basic five sensory systems need to be working simultaneously and cooperatively for acquisition and performance of higher skills. In fact, if any one system does not work properly either by itself or in conjunction with the others, a sensory dysfunction of some kind may result. The degree of sensory dysfunction, whether it manifests as merely a “sensory issue” or mild, moderate, or severe sensory dysfunction, is highly dependent on which senses and/or sensory systems are impaired and to what extent. Again, the sensory systems actually operate in conjunction and cooperation with one another, so the resultant behavior or sensation is probably the outcome of accessing more than one system [79].
Often a child with sensory integration dysfunction will be categorized as immature and possible spoilt. The new social, cognitive, and motor demands of the school setting often create even more confusion, anxiety, and chaos for the child who has sensory integration dysfunction [79]. Now, let us explore some of the systems that may indicate sensory integration dysfunction.
2.4.1 Dysfunction in the Proprioceptive System
This refers to an inefficiency in the proprioceptive system that may cause a child to have difficulties both at school and at home. Since the proprioceptive system gives feedback from muscles and joints, it supports holding a pen or a pencil correctly, staying properly seated in a chair, and learning to use a knife, fork and spoon correctly. This system also supports learning to walk, opening and closing a jar or a door, using playground equipment, and chewing [79].
2.4.2 Dysfunction in the Tactile System
A dysfunction in the tactile system may manifest in a variety of ways. A child may be actively defensive and not want to be touched, bumped, or hugged, or to touch certain textures or wear certain types of clothes. An atypical tactile system may also cause a child to crave certain tactile sensations and experiences, such as never being able to keep their hands to themselves, constantly touching and feeling the people and/or things around them. In other words, the tactile system greatly influences relationships, fashion sense (clothing choice), and school performance [79].
2.4.3 Dysfunction in the Vestibular System
A problem or inefficiency in the vestibular system may cause difficulty with balance, coordination and motor planning. This may manifest in the child as clumsiness and uncoordinated fine motor activities, such as eating, drinking, using writing utensils etc. The vestibular system will play a key role in the ability of the child to participate successfully in sport, in the gym class or on the playground, and in various activities at school and at home [79].
2.4.4 Possible Sensory Signs and Symptoms
Let us now explore a wide range of behaviors and symptoms that may indicate some level of sensory dysfunction. This list is not meant to be used for diagnostic purposes, but rather to create awareness of some of the sensory difficulties a child may experience over the course of development and for which specialist help might be needed.
-
Young children may be overly sensitive to touch. Touch is interpreted as a nociceptive stimulus and therefore working in their mouth is very difficult. For the same reason these children dislike bathing, brushing their teeth and other self-care activities. These children may be oversensitive to pain.
-
Young children may be underactive to touch. These children exhibit oral hyperactivity by constantly eating or chewing. These children may be “chewers”—they chew shirts, blankets, toys, toothpaste etc.
-
Children who do not tolerate the taste of certain foods, and therefore vomit or gag easily, and always have the same lunch; i.e., they are very picky eaters. This suggests gustatory sensory dysfunction.
-
Children who do not know when their mouth is full and so stuff their meal into the mouth. This behavior suggests proprioceptive, tactile sensory dysfunction.
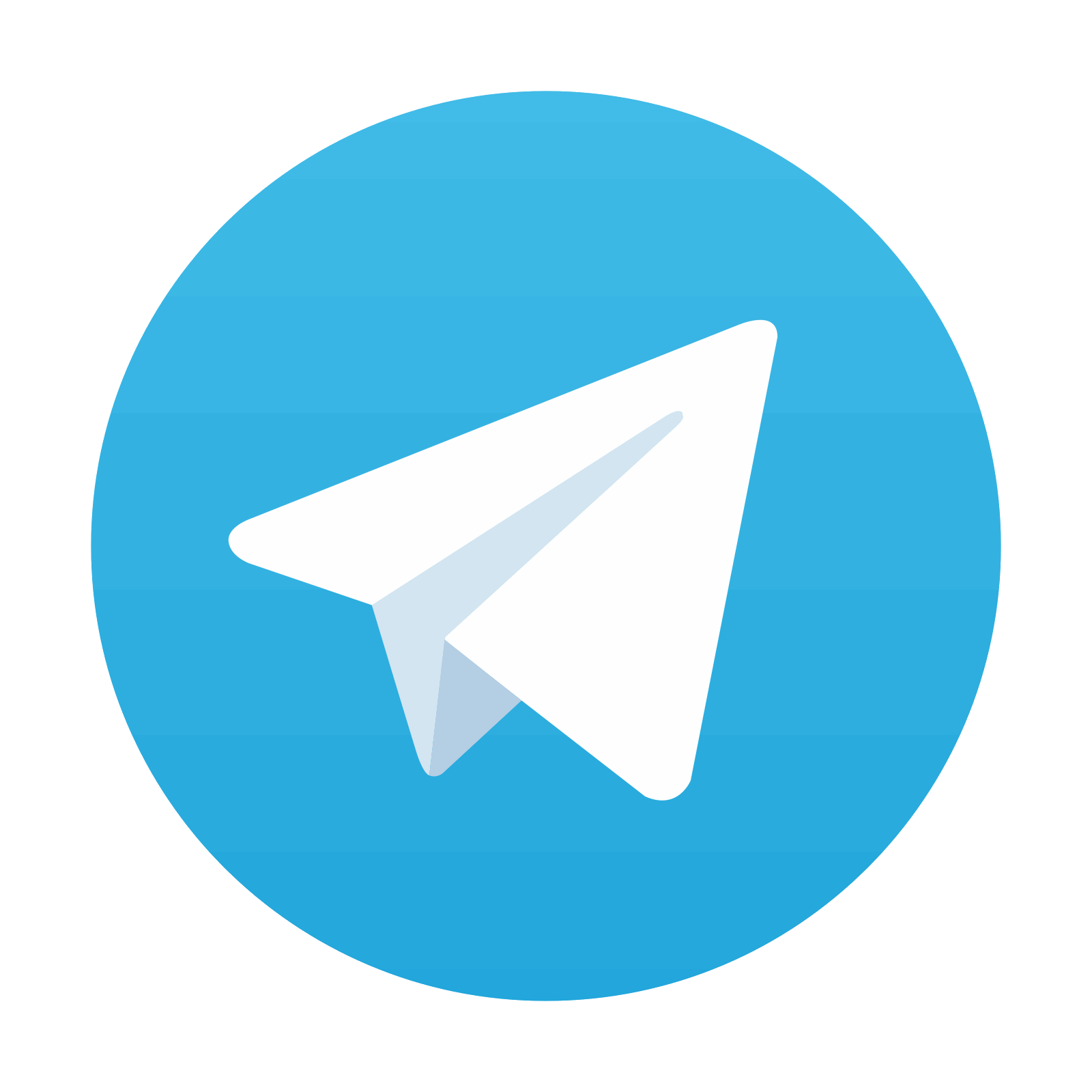
Stay updated, free dental videos. Join our Telegram channel

VIDEdental - Online dental courses
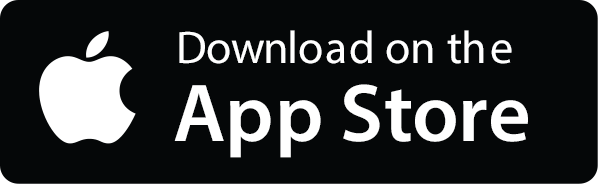
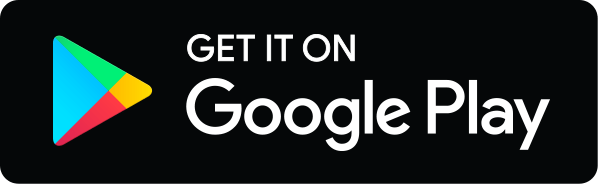