Fig. 14.1
Micrograph of dentin from a severely fluorosed tooth showing distinct layering of hypomineralized lines (Used with permission of John Wiley and Sons from Fejerskov et al. [4])
Moreover, numbers of studies have found that the severity of dentin fluorosis is related to fluoride levels in the drinking water, dentin fluoride concentration, and dentin microhardness [13–15]. Studies by Vieira et al. [13] measured microhardness and dentin mineralization in tooth samples from Montreal and Toronto, Canada, and Fortaleza, Brazil, where water fluoride levels were 0.2, 1.0, and 0.7 ppm, respectively. They found that dentin fluoride concentrations correlated with dental fluorosis severity. Dental fluorosis severity correlated with dentin microhardness, while enamel fluoride concentrations correlated with both dentin microhardness and dentin mineralization. Interestingly, the teeth from Brazil exposed to 0.7 ppm fluoride in the drinking water presented with the highest dentin fluoride concentration values and were also harder and less mineralized than the Montreal (0.2 ppm) and Toronto (1.0 ppm) teeth. The Montreal (0.2 ppm) teeth had lower levels of dental fluorosis when compared with teeth from Toronto (1 ppm) and Fortaleza (0.7 ppm).
Severely fluorosed deer [15] have a disrupted dentin crystal structure. The disrupted crystal formation may relate to the findings in a recent in vitro study by Waidyasekera and co-workers [16], who described crystallites in fluorosed human dentin as larger, but not homogenously arranged, and found a lower density of crystallites in fluorosed dentin.
14.2.2 Alteration in Extracellular Matrix Properties
Similar to the enamel matrix, the physicochemical effect of fluoride incorporated into the crystal lattice could directly regulate the growth of hydroxyapatite crystals in dentin. However, the molecular mechanism of hypomineralization in fluorotic dentin is not yet fully elucidated. Several studies have suggested the changes in the inorganic phase of mineralization in dentin are due to structural alteration in the extracellular matrix resulting from changes in synthesis or modification of collagenous and noncollagenous extracellular matrix proteins.
Type I collagen is the major organic component of dentin extracellular matrix constructing structural basis of the dentin matrix. Moseley et al. reported that 6 mM sodium fluoride supplemented in dentin organ culture inhibited collagen synthesis [17]. A further in vivo study by Maciejewska et al. showed that type I collagen expression was downregulated in the early stage of tooth germs from pulps (P1 or P5) from dams that ingested 110 ppm fluoride [18].
Proteoglycans are a class of glycosylated proteins consists of sulfated glycosaminoglycans (i.e., chondroitin sulfate, dermatan sulfate, heparan sulfate, heparin, keratan sulfate) covalently linked to core proteins. In mineralized tissues like dentin, proteoglycans are essential for maintenance of the stabilization of collagen fibers and act as a framework for mineralization in association with type I collagen. The proteoglycan extracted from rat teeth received 20 ppm fluoride for 10 weeks exhibited a more anionic profile due to changes in glycosaminoglycan characteristics and the presence of additional dermatan sulfate and heparin sulfate [19]. Changes in production of proteoglycans have also been demonstrated in vitro. Tooth organs cultured for 14 days in media supplemented with higher levels of fluoride than would be found in serum in vivo (1–6 mM) showed a reduction in biglycan, but no change in decorin or versican. In this same study, the levels of dermatan sulfate and sulfation of glycosaminoglycans in predentin and pulp extract were reduced by supplementation with 6 mM fluoride [20].
It is known that the each proteoglycan has different roles in matrix formation and mineralization. Chondroitin sulfate-substituted small leucine-rich proteoglycan/SLRP (including decorin and biglycan) within dentin may function to regulate the mineralization process, while dermatan sulfate-substituted SLRPs inhibit collagen fibril formation [21]. The functions of these different proteoglycans are partially explained by the different binding abilities of these molecules to hydroxyapatite [22, 23]. Septier et al. have demonstrated that the expression of glycosaminoglycan and dermatan sulfate/chondroitin sulfate in predentin decreases toward the predentin (unmineralized)/dentine (mineralized) interface, indicating regulation of the mineralization status of dentin as one of the roles of proteoglycans [6]. If fluoride at physiological levels (micromolar) can alter proteoglycans in the dentin matrix, it would certainly alter dentin mineralization.
14.2.3 Alteration in Mineralization Front
Dentin phosphoprotein (DPP, a protein hydrolysis product of dentin sialophosphoprotein/DSPP) is the most abundant noncollagenous protein in the dentin matrix [24, 25] and is reported to have diverse effects on mineralization [26–30]. Because it is highly phosphorylated [31, 32], DPP has very high binding capacity for calcium ions and an affinity for crystalline hydroxyapatite surfaces, suggesting its role in nucleating mineral initiation at the mineralization front [33]. The phosphate content of DPP has been reported to be significantly reduced in dentin of rats receiving 20 ppm fluoride in drinking water for 17 weeks [34]. The possible mechanism of this finding was suggested by an in vitro study [35] showing that physiological concentration of fluoride (0.04–4 μM) inhibits casein kinase II, a potent kinase that phosphorylates DPP [24, 25].
Appositional mineralization of circumpulpal dentin has been shown to progress rapidly at the mineralization front. A lightly mineralized layer (LL) is observed in predentin immediately adjacent to the mineralization front of dentin [36, 37]. Crystal alignment in LL is within narrow spaces between the individual non-mineralized collagen fibers, thereby making LL distinct from the bulk of fully mineralized dentin where crystals are deposited in and around collagen fibers. This indicates that LL is where dentin mineralization initiates before more rapid mineralization of the matrix occurs (Figs. 14.2a–c and 14.3a–c). The LL along the mineralization front is clearly recognized at the crown-analogue dentin of the continuously erupting mouse and rat incisors as compared to root-analogue dentin. This is likely due to the differences in both organic and inorganic components between the crown- and root-analogue dentin matrix [36, 38–42].
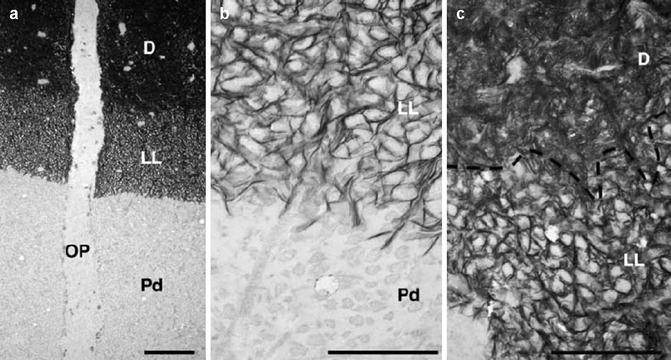
Fig. 14.2
Lightly mineralized layer (LL). (a) LL along the mineralization front of normal rat dentin. (b, c) LL at high magnification (Used with permission of Oxford University Press from Ahmad et al. [36] OP odontoblast process, PD predentin)
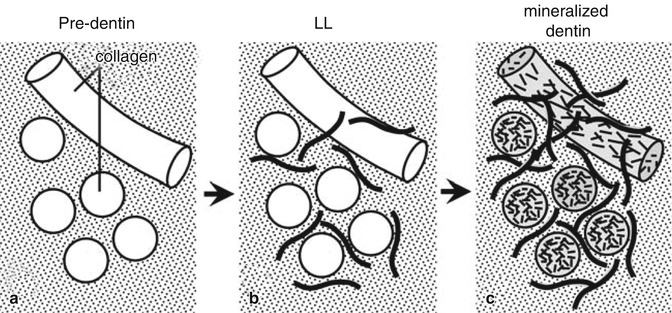
Fig. 14.3
Diagrammatic model of stepwise mineralization at the mineralization front of dentin. (a) Predentin without mineral deposits. (b) LL where mineral crystals deposited in noncollagenous matrix between collagen fibers. (c) Mineralized dentin after massive mineral deposition in and around collagen fibers (Used with permission of Oxford University Press from Ahmad et al. [36])
DPP is present in the crown-analogue dentin at about four times greater levels than in the root-analogue dentin [39]. DPP has been reported to delay the mineralization of collagen fibers in the rodent incisor crown-analogue dentin, though at lower concentrations DPP promotes the nucleation of hydroxyapatite [36, 42–44]. These findings are consistent with those of Boskey et al., who showed that high concentrations of DPP can inhibit the growth of nucleated crystals.
Mice ingesting 50 ppm fluoride in drinking water appear to lose LL in dentin from mice as compared to dentin from control mice (our unpublished data, Takano Y. 2014), with an abrupt change in the mineralization status of collagen fibers at the border between predentin and mineralized dentin under the influence of fluoride. These results, combined with our findings of reduced immunostaining for DSP (another hydrolysis product of DSPP to create DPP) in odontoblasts of the fluoride treated mice, further support the possibility that the loss of LL is caused by a reduction in the synthesis of DPP (Fig. 14.4a, b) (unpublished data, Nakano Y. 2014), allowing more rapid mineralization at the dentin mineral initiation front. These results support the possibility that the fluoride can have a direct effect on odontoblasts to reduce either the synthesis of DSPP or the hydrolysis of DSPP to form DPP.
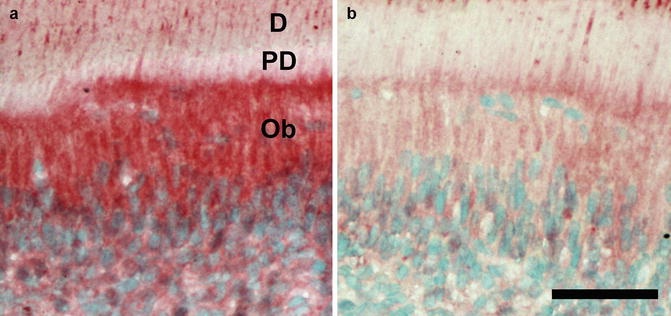
Fig. 14.4
(a, b) Immunostaining of DSPP/DSP. DSPP/DSP is expressed in cytoplasm of odontoblasts and adjacent pulp cells. DSPP/DSP expression in cytoplasm of odontoblasts is downregulated in fluoride (50 ppm)-exposed A/J mice. Ob odontoblast, D dentin, PD predentin, bar 100 μm
14.3 Effects of Fluoride on the Dental Pulp
Few studies have specifically looked at the effects of fluoride on dental pulp. However, the changes that have been identified are consistent with those found in odontoblasts. In vitro studies of human dental pulp cells exposed to fluoride show that below 5 ppm fluoride, cell proliferation and alkaline phosphatase synthesis increase [45, 46]. However, at higher fluoride concentrations, alkaline phosphatase and type 1 collagen synthesis decrease, with no similar effects on fibronectin synthesis [47] or change in cell morphology [17]. Fluoride incorporation into pulp capping agents has been shown to reduce thermal conductivity and dentin acid solubility, with no irritating effects on the pulp [48]. At high concentrations, fluoride can also inhibit lysosomal enzymes found in the pulp, with a possible application of limiting cellular destruction in damaged pulp tissue [49].
14.4 Clinical Significance of Dentin Fluorosis
These relatively few studies showing that fluoride can alter dentin and pulp formation suggest that more attention should be given to possible changes in fluorosed dentin. Possible effects of fluorosed dentin include alterations in caries susceptibility, alterations in the dentin structure to affect bonding of composite based dental materials, the capability of dentin to remineralize, secondary dentin formation, and dentin bridge formation. One aspect of fluoride-related effects on dentin formation has been explored in analyzing the effects of fluoride in drinking water on the so-called hidden caries.
14.4.1 Fluorosis and Dentin Caries
In the 1980s the term “hidden caries” was introduced to dentistry. This term is used to describe occlusal pit and fissure-type carious lesions seen in the dentin on a bitewing radiograph, where clinically the occlusal enamel appears intact or is minimally demineralized [50] (Fig. 14.5). These hidden caries create the risk that after cariogenic bacteria penetrate into the enamel through a minimal opening in the enamel surface, when they reach the less mineralized dentin, caries progresses at an increased rate. Meanwhile the fluoridated enamel undergoes remineralization, while caries progresses at an increased rate in the dentin. Hidden carious lesions present a challenge to dentists in diagnosis, treatment planning, and research, due to their “hidden” nature, resulting in misdiagnosis and treatment of dental caries [51].
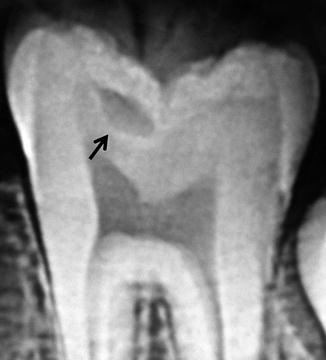
Fig. 14.5
Hidden caries. Radiolucency in the dentin (arrow) under an intact enamel surface suggests hidden caries
In a clinical study to investigate the possible role of fluoride in the formation of hidden caries, Weerheijm et al. showed that children ingesting “optimally” fluoridated water at a level of 1.1 ppm from birth until the day of data collection had significantly fewer hidden caries than the group of children drinking water containing 0.1 ppm. Hashizume et al. examined the role of public water fluoridation and fluoride dentifrice on the prevalence of hidden caries in 8–10-year-old children and, similar to the study by Weerheijm et al., found a statistically significant reduction in the prevalence of hidden caries by water fluoridation at currently recommended level (0.7 ppm) [52]. These results suggest that the ingestion of fluoride at optimal levels can reduce the presence of radiographically detectable hidden caries.
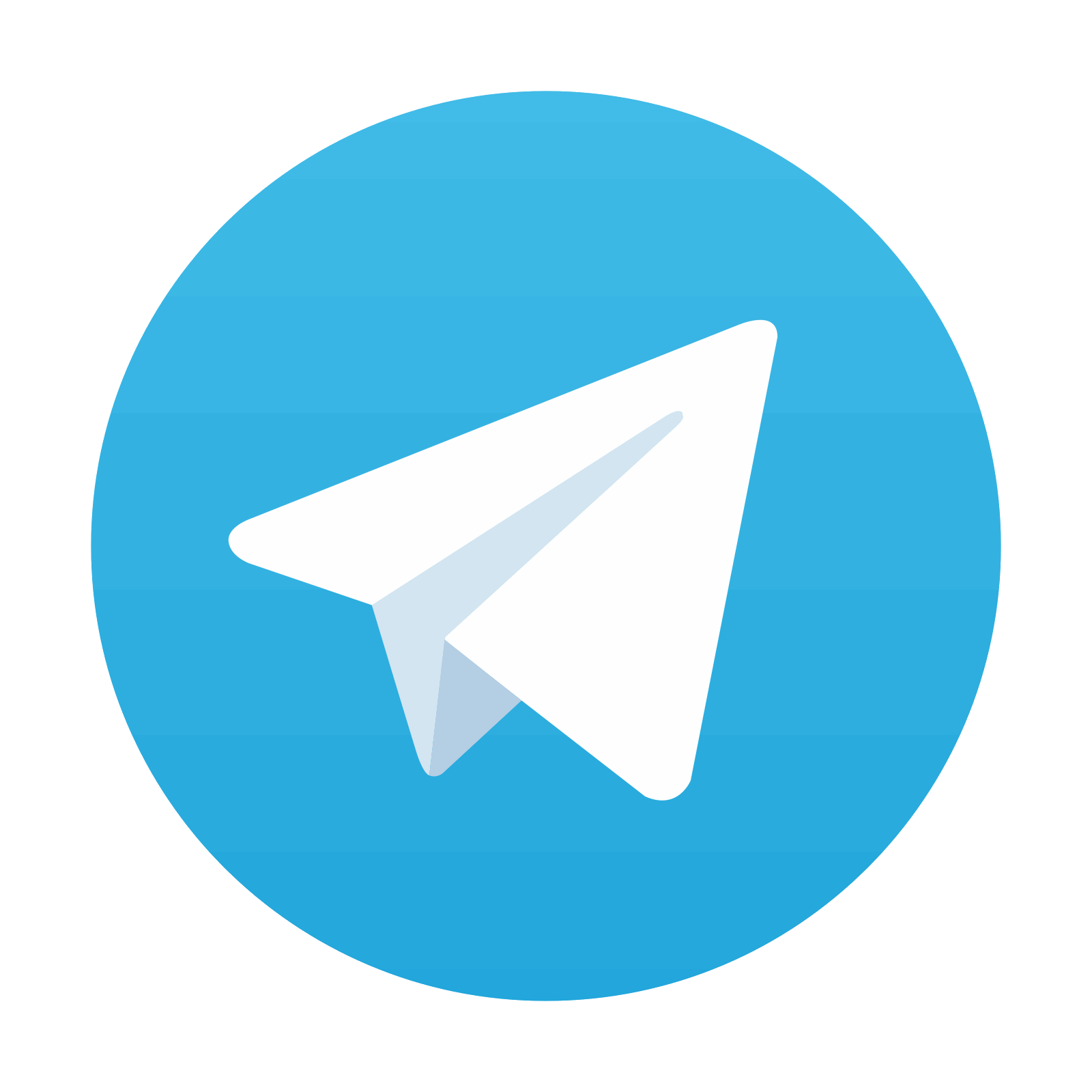
Stay updated, free dental videos. Join our Telegram channel

VIDEdental - Online dental courses
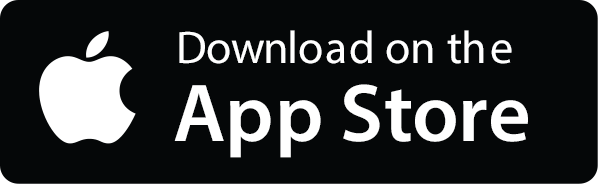
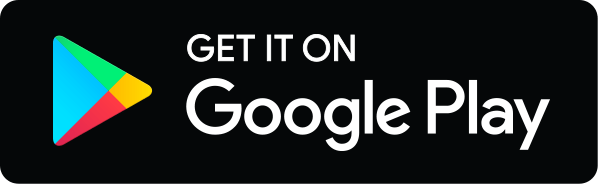