Abstract
Objective
The aim of this study was to investigate the influence of the photoinitiator system on the polymerization kinetics of methacrylamide-based monomers as alternatives to methacrylates in adhesives dental-based materials.
Methods
In total, 16 groups were tested. Monofunctional monomers (2-hydroxyethyl methacrylate) – HEMA; (2-hydroxy-1-ethyl methacrylate) −2EMATE, (2-hydroxyethyl methacrylamide) – HEMAM; and ( N -(1-hydroxybutan-2-yl) methacrylamide) −2EM; were combined with bifunctional monomers containing the same polymerizing moieties as the monofunctional counterparts (HEMA-BDI; 2EMATE-BDI; HEMAM-BDI; and 2EM-BDI) at 50/50 M ratios. BHT was used as inhibitor (0.1 wt%) and the photoinitiators used were: CQ + EDMAB (0.2/0.8), BAPO (0.2), IVOCERIN (0.2), and DMPA (0.2), in wt%. The polymerization kinetics were monitored using Near-IR spectroscopy (∼6165 cm −1 ) in real-time while the specimens were photoactivated with a mercury arc lamp (Acticure 2; 320−500 nm, 300 mW/cm 2 ) for 5 min, and maximum rate of polymerization (Rp max , in %.sˉ̄ 1 ), degree of conversion at Rp max (DC@Rp max , in %), and the final degree of conversion (Final DC, in %) were calculated (n = 3). Initial viscosity was measured with an oscillating rheometer (n = 3). Data were analyzed using Two-way ANOVA for the polymerization kinetics and one–way ANOVA for the viscosity. Multiple comparisons were made using the Tukey’s test (∝ = 0.05).
Results
There was statistically significant interaction between monomer and photoinitiator (p < 0.001). For the methacrylates groups, the highest Rp max was observed for HEMA + DMPA and 2EMATE + BAPO. For methacrylamides groups, the highest Rp max were observed for HEMAM and 2EM, both with DMPA. Final DC was higher for the methacrylate groups, in comparison with methacrylamide groups, independent of the photoinitiators. However, for the methacrylamide groups, the association with BAPO led to the lowest values of DC. In terms of DC@Rp max , methacrylate-based systems showed significantly higher values than methacrylamide formulations. DMPA and Ivocerin led to higher values than CQ/EDMAB and BAPO in methacrylamide-based compounds. BAPO systems showed de lowest values for both HEMA and HEMAM formulations. For the viscosity (Pa.s), only 2EM had higher values (1.60 ± 0.15) in comparison with all monomers. In conclusion, polymerization kinetics was affected by the photoinitiators for both monomers. Viscosity was significantly increased with the use of secondary methacrylamide.
Significance
this work demonstrated the feasibility of using newly-synthesized methacrylamide monomers in conjunction with a series of initiator systems already used in commercial materials.
1
Introduction
The composition of the vast majority of dental adhesive systems available on the market includes methacrylates, mainly because their chemical and mechanical properties have shown satisfactory results in this application [ ]. The mono-methacrylate 2-Hydroxyethyl (HEMA) was incorporated in the composition in the late 1960’s due to its “water-chasing” capabilities, which in turn allowed for wet bonding to the dentin substrate using total etch techniques [ ]. Until the introduction of more hydrophilic adhesive compositions, the bonding could only be reliably performed within enamel margins, so the introduction of bonding to dentin and the novel description of the hybrid layer were revolutionary [ ]. However, since that time, in spite of active research in the area [ , ], very few products with monomers different from HEMA have been introduced in the market. The clinical studies that followed this products have demonstrated acceptable performance [ , ], in spite of lower in vitro results compared to other commercial products [ ]. There is interest in pursuing alternatives to HEMA, since this monomer imparts significant water sorption and solubility to the adhesive, and contains ester bonds that make it prone to hydrolysis in the oral environment [ , , ]. In addition, studies have shown that the degradation of methacrylates is accelerated at low pH, a common condition in some adhesive compositions and also when caries-forming biofilm is present mainly in gingival margins at proximal surfaces [ ]. As a result, polymer properties can be compromised, resulting in premature bonding degradation, secondary decay or restoration de-bonding [ , ]. The degradation of the adhesive can also pose toxicity to pulp cells through the dentinal tubules or even to adjacent soft tissues in regions of the restoration close to gingival margins [ ].
Since the maintenance of the integrity of the bonded interface is crucial to the longevity of the restoration, novel monomer alternatives such as acrylamides and methacrylamides have been proposed [ , ]. Methacrylamides and acrylamides have an amide group instead of an ester group, which makes them less prone to degradation in the oral environment. This is due to the fact that the electronegativity of the nitrogen is greater than the oxygen present in the methacrylate, which stabilizes the bond via resonance with the lone pair [ ]. The energy required to break the bond between the carbon in the carbonyl and the nitrogen in the amide is much greater than the energy required to break the bond with the oxygen in the methacrylate [ ]. Therefore, it can be envisioned that these monomers will be less prone to the hydrolysis via acid-base reactions in aqueous environment, and even through the great advantage from the bond preservation standpoint. Also it means that the vinyl bond attached to the methacrylamide is more stable, which leads to concerns over the reactivity of acrylamides and methacrylamides [ ]. Previous study has demonstrated that steric constraints such as the ones observed in tertiary methacrylamides significantly reduce monomer reactivity [ ].
One approach to circumvent the lower reactivity of methacrylamides is to use initiator systems with greater quantum yields than what is observed for the conventional camphorquinone/amine pair [ ]. The efficiency of this Norrish-type photoinitiator system can be improved by the addition of electron acceptors such as diphenyl iodonium salts [ ]. Studies with methacrylate monomers have shown significantly improved rates of polymerization and conversion with the addition of iodonium salt concentrations of around 1 mol% [ ]. In addition, the use of cleavage-type photoinitiators such as phenylbis(2,4,6-trimethylbenzoyl) phosphine oxide (BAPO) and 2,2-dimethoxy-2-phenylacetophenone (DMPA) can also improve reactivity, since they rely on a single electron transfer event to generate twice the amount of radicals compared to CQ/amine [ ]. In fact, their quantum yields of conversion are two to four-fold that of the CQ/amine pair [ ]. More recently, photoinitiators based on germanium chemistry have also been described [ ]. These are also cleavage-type initiators, and have been shown to be suitable for the polymerization of methacrylates and methacrylamides [ ]. Bis-(4-methoxybenzoyl)diethyl germane (brand name Ivocerin, Ivoclar-Vivadent, Liechtenstein) and BAPO can be activated by visible light, at a slightly shorter wavelengths compared to CQ (405 and 470 nm, respectively), which also improves light penetration through the bulk of the material [ ].
The present study had two distinct aims: firstly was to develop alternative monomers to BisGMA and HEMA, based on secondary methacrylamides, for future potential use as adhesive monomers. The monomers were prepared with systematically varied structures to allow for the assessment of the effect of steric hindrance on the kinetics of polymerization. Methacrylate analogs with similar steric constraints were also synthesized to allow for direct comparisons with the novel methacrylamides. Secondly was to evaluate the polymerization kinetics of these monomers when in combination with different types of initiators, including the conventional CQ/amine and three other examples of cleavage-type initiators. The null hypotheses of this study were: (1) the methacrylamide-based monomers would have the same polymerization kinetics behavior as the methacrylate-based monomers, and (2) the polymerization kinetics would not be influenced by the type of initiator used.
2
Material and methods
2.1
Monomer synthesis, material composition, and specimen preparation
Unless otherwise noted, all commercially-available materials were obtained from Sigma-Aldrich (Milwaukee, WI, USA). The synthesis procedures for the materials produced de novo are described in the supplemental materials. Four different monofunctional monomers were used: two commercially available (2-hydroxyethyl methacrylate (HEMA); and N -hydroxyethyl methacrylamide (HEMAM)) and two synthesized de novo (α-substituted methacrylate 2-hydroxy-1-ethyl methacrylate (2EMATE); and 2-hydroxy-1-ethyl methacrylamide (2EM)). The OH-bearing monofunctional monomers were then reacted with a difunctional isocyanate (1,3-bis (1-isocyanato-1-methylethylbenzene) – BDI) at 2:1 mol ratio, resulting in difunctional urethanes. The structures of all monomers used in this study are shown in Fig. 1 .

Each monofunctional monomer was combined at 50:50 mass ratio with the corresponding difunctional urethane, resulting in four different monomer systems – HEMA+HEMA-BDI, 2EMATE+2EMATE-BDI, HEMAM+HEMAM-BDI and 2EM+2EM-BDI. For simplicity’s sake, throughout the manuscript, the groups containing a pair of monofunctional monomer plus its analogous difunctional monomer with the BDI core will be referred to by the name of the monofunctional monomer. For example, HEMA+HEMA-BDI will be referred to as HEMA. These were made photopolymerizable by the addition of one of the following four photoinitiator systems: 0.2/0.8 wt% dl-camphoroquinone/ethyl 4-dimethylaminobenzoate (CQ/EDMAB – λ max = 470 nm), 0.2 wt% phenylbis(2,4,6-trimethylbenzoyl) phosphine oxide (BAPO – λ max = 380 nm); 0.2 wt% 2,2-dimethoxyphenoxy acetophenone (DMPA – λ max = 365 nm); or 0.2 wt% bis-(4-methoxybenzoyl)diethyl germane (IVOCERIN™, donated by Ivoclar-Vivadent, Schaan, Liechstein – λ max = 405 nm). 0.1 wt% 2,6-di-tert-butyl-4-methylphenol (BHT) was used as inhibitor. Materials were formulated under filtered yellow lights. Molecular structures for photoinitiator systems are shown in Fig. 2 .

2.2
Viscosity
The initial viscosity in Pa.s n = 3 was measured with an oscillating rheometer DH-R1, TA Instruments, New Castle, DE, USA. Approximately 20 mg of each monomer mixture were placed between 20-mm diameter plates separated by a 300 μm gap, and tested at room temperature with strain rate ranging from 10 to 1000 Hz flow sweep mode.
2.3
Photopolymerization reaction kinetics and degree of conversion
Specimens were made using a rubber mold 10 mm in diameter and 0.8 mm thick, laminated between two glass slides. Polymerization kinetics (n = 3) was monitored using near–infrared (NIR) spectroscopy in real time. The vinyl overtones for the methacrylate and methacrylamide (6165 and 6135 cm −1 , respectively) were followed with 2 scans per spectrum at 4 cm −1 resolution, resulting in 2 Hz data acquisition rate. The test was recorded continuously during 300 s of irradiation with a mercury arc lamp filtered to 320−500 nm (Acticure 4000, EXFO, Mississauga, ON, Canada). The tip of the light guide was positioned 1.5 cm away from the surface of the specimen, so to deliver approximately 300 mW/cm 2 . Selected groups (HEMA and HEMAM-containing materials) were also evaluated using a mono-wave LED light source (Elipar, 3M-ESPE, St. Paul, MN, USA), centered at 470 nm and delivering an irradiance of 840 mW/cm 2 to the surface of the specimen for 60 s. Polymer conversion was calculated based on the area of the overtone peak before and during polymerization [ ], and the rate of polymerization was calculated as the first derivative of the conversion vs. time curve.
2.4
Characterization of the light curing units
The emission spectra and irradiance of all light curing units (LCU) used in this study were obtained using a UV–vis detector (MARC Resin Calibrator, Blue Light Analytics Inc., Halifax, NS, Canada). The tip of the LCU was positioned parallel to the surface of the top detector, separated by 1.5 cm to mimic the conditions of the polymerization kinetics on the IR chamber. Spectra were recorded after light stabilization, 5 s after power up. The irradiance for each individual wavelength band was calculated in mW/cm 2 .
2.5
Characterization of the absorption spectra of each photosensitizer
Each initiator (0.008 g of EDMAB and 0.002 g of the others) was dissolved in 1 ml of ethanol. These concentrations were selected to match the mass ratio of initiators in the materials as tested. Therefore, the results are reported in arbitrary units, rather than in molar absorptivity units. The solutions were placed in UV-transparent cuvettes and UV–vis spectra (300−540 nm) were obtained using a UV–vis spectrophotometer (Evolution 201, Thermo Fisher Scientific, Waltham, MA), with 1 nm sampling interval.
2.6
Statistical analysis
The data was tested for normality (Anderson-Darling) and homoscedasticity (Bartlett/Levene). Degree of conversion, maximum rate of polymerization and conversion at rate maximum data were analyzed with two-way ANOVA (monomer type and photoinitiator system). The data for initial monomer viscosity failed the normality and homocedasticity tests, so one-way ANOVA was performed on ranks (Kruskall-Wallis). Multiple comparisons were done using Tukey’s test. The significance level of 95% was used for all tests.
3
Results
3.1
Viscosity
Results for viscosity are presented in Table 1 . Both methacrylate-based formulations were statistically similar to each other (HEMA = 0.067 and 2 EMATE = 0.05 Pa.s), and showed statistically lower viscosity than the methacrylamide systems (HEMAM = 3.567 and 2 EM =40.0 Pa.s). The methacrylamide 2 EM monomer system presented the highest viscosity of all materials (p = 0.001; F = 874.7).
Monomer system | Viscosity (Pa s) |
---|---|
HEMA | 0.067 ± 0.006 c |
2EMATE | 0.050 ± 0.007 c |
HEMAM | 3.567 ± 0.303 b |
2EM | 40.0 ± 12.578 a |
3.2
Light source and photoinitiator spectra
The emission spectra of the two light curing units used in this study are shown in Fig. 3 . The Acticure presents three distinct emission peaks, centered at 365, 405 and 435 nm, with approximately 157, 53 and 90 mW/cm 2 absolute irradiance, respectively. These values were calculated by integrating the area under those peaks. The Elipar is a mono-wave LED, with emission spectra centered at 450 nm and irradiance of 840 mW/cm 2 . Irradiance for each individual peak is shown in Table 2 .

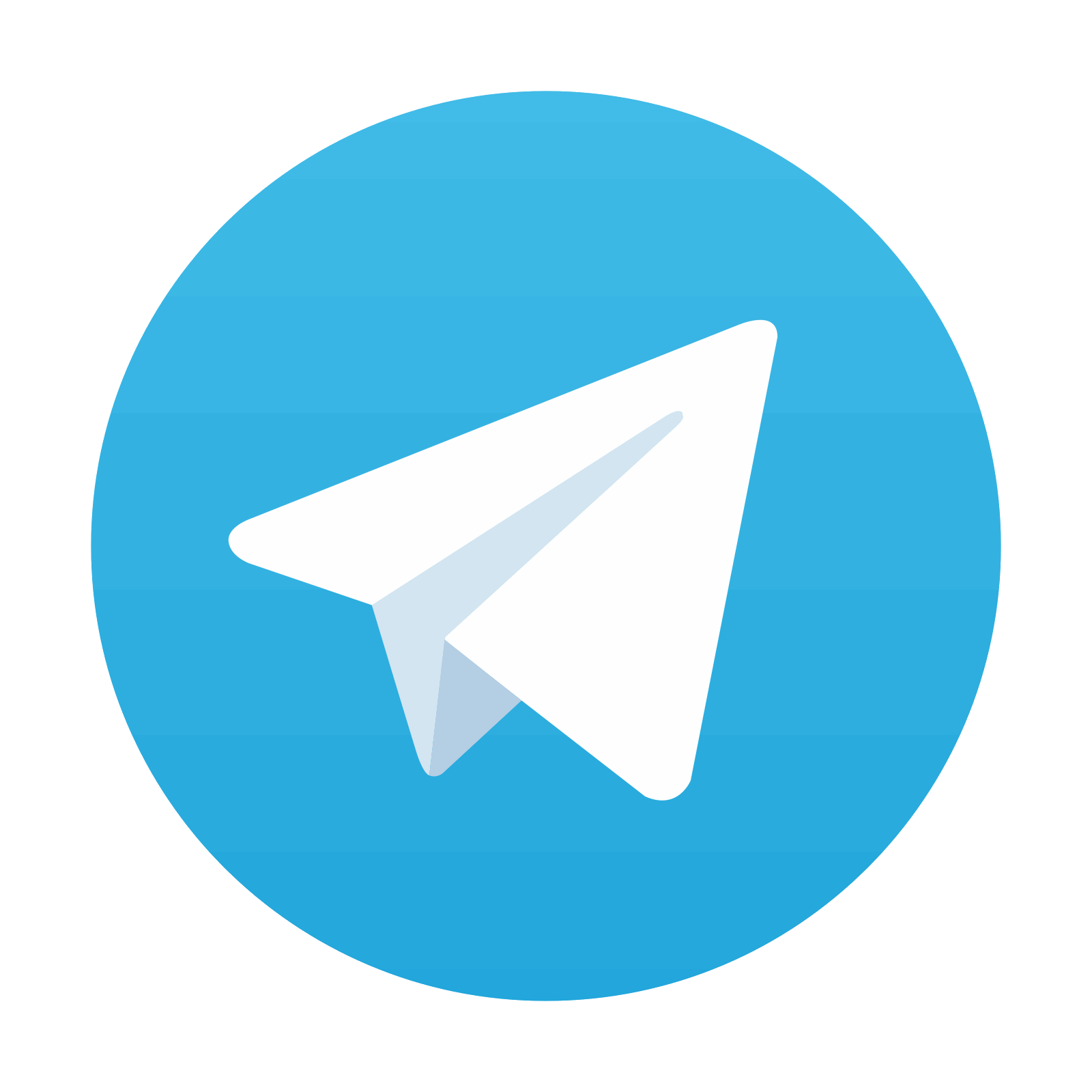
Stay updated, free dental videos. Join our Telegram channel

VIDEdental - Online dental courses
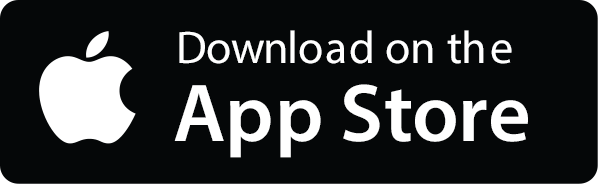
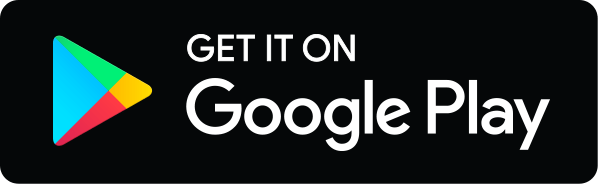
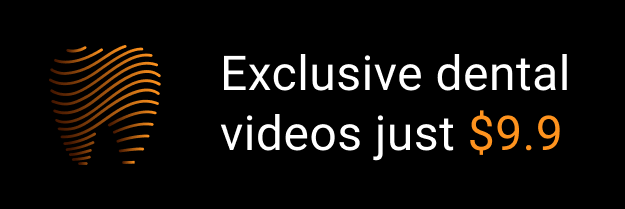