Graphical abstract

Highlights
- •
Zirconia dental pieces were produced by robocasting (AM) and subtractive manufacturing (SM).
- •
AM samples revealed to be biologically safe and present adequate properties.
- •
AM samples are more porous, less hard and tough and more hydrophilic than SM samples.
- •
Although both samples do not suffer wear on chewing simulation, cusps wear less against AM.
- •
Glazing of both samples led to a dramatic increase of the antagonist cusps wear.
Abstract
Objectives
The main goal of this work is to evaluate the suitability of nanostructured zirconia pieces obtained by robocasting additive manufacturing (AM), for dental applications.
Methods
The density, crystalline structure, morphology/porosity, surface roughness, hardness, toughness, wettability and biocompatibility of the produced samples were compared with those of samples obtained by conventional subtractive manufacturing (SM) of a similar commercial zirconia material. Chewing simulation studies were carried out against dental human cusps in artificial saliva. The wear of the material was quantified and the wear mechanisms investigated, as well as the influence of glaze coating.
Results
AM samples, that revealed to be biocompatible, are slightly less dense and more porous than SM samples, showing lower hardness, toughness and wettability than SM samples. After chewing tests, no wear was found both on AM and SM samples. However, the dental wear was significantly lower when AM samples were used as counterbody. Concerning the glazed samples, both coated surfaces and dental cusps suffered wear, being the cusps’ wear higher than that found for unglazed samples. More, cusps tested against AM coated samples suffered less wear comparatively to those opposed to SM coated samples.
Significance
Overall, the results presented in this paper show that AM processed nanostructured zirconia can be used in dental restorations, with important advantages from the point of view of processing and tribological performance. Moreover, the option for glaze finishing should be carefully considered both in SM and AM processed specimens.
1
Introduction
Synthetic dental materials are used to repair/replace missing tooth tissue, allowing re-establishing masticatory, phonetic and aesthetic functions. Among them, ceramics play an important role, since they are highly compatible with oral environment, present good chemical resistance and suitable mechanical and optical properties to mimic the natural teeth [ ]. Zirconia is the most used ceramic for dental crown replacement [ , ]. It presents high strength, toughness, hardness, and resistance to wear and corrosion, being easily machinable in the pre-sintered state [ ].
Nowadays, the processing of zirconia dental structures is generally based on CAD/CAM of compact or pre-sintered core materials. Subtractive manufacturing (SM) is currently used to produce these types of structures by removing surplus material from ceramic blocs in milling units. However, this methodology impairs the production of parts with intricate internal details, because the millings units have severe constraints to the angulations [ ]. More, the production of complex pieces leads to a more time-consuming and expensive cost of fabrication [ ]. Additionally, this method involves a large amount of wasted raw material. To overcome the drawbacks of SM. To overcome the drawbacks of SM, a new type of technologies based on additive manufacturing (AM) (also referred as 3D printing) is emerging as a tool with great potential to build up long term dental structures [ ]. In the dental field, AM techniques are considered promising and a valuable tool to ensure mass customization, with higher efficiency, repeatability and reproducibility [ ]. They also allow lower production costs comparatively to SM, a faster production of customized dental pieces with complex details (e.g. intricate internal geometries and arbitrary angles [ ]), since they involve less manufacturing steps. Moreover, they reduce energy consumption and material waste since the amount of material needed is only what is required to build the part, and eliminates the use of conventional manufacturing tools [ ]. AM also allows the production of structured composite materials by combining different materials to improve the mechanical and tribological properties [ ].
Recently, some studies focused on the production of zirconia pieces using different AM techniques (e.g. Vat polymerization/stereolithography [ ] and material extrusion/jetting [ ]) and investigated properties as density, porosity, surface roughness, microstructure, dimensional accuracy, morphology and mechanical behaviour. Such properties depend on the ceramic paste composition (e.g. solid content, solid particles size, addition of dispersants and binders) and printing conditions (e.g. nozzle size, deposition velocity and temperature). It was found that it is possible to produce dense 3D components (relative densities 96.9–99.3 % [ , ]) with mechanical properties close to milled zirconia (flexural strength 943−1154 MPa [ , ], fracture toughness 6.4–6.7 MPa·m 1/2 [ , ] and hardness 13.9 GPa [ ]) and high dimensional stability [ , , ]. However, none of the above-mentioned works studied the tribological behaviour of the produced materials. To be used in dental crown replacement/restoration, zirconia must be resistant to the stress induced during mastication without suffering significant wear. Additionally, they also shall prevent the abnormal wear of the antagonist natural tooth surfaces [ ], since the loss of dental material may affect negatively the chewing ability, dental sensitivity and aesthetics.
In order to improve restorations aesthetic properties (e.g. colour, brightness and translucency), zirconia dental pieces are usually manually coated with feldspathic ceramics [ , , ] which differ in composition and microstructure, but fulfil stablished standards concerning mechanical properties (e.g. minimum flexural strength 50 MPa [ ]). However, both clinical practice and in-vitro testing show a typical failure pattern of such coatings, which involves chipping, delamination, wear and fracture [ , ].
The aim of the present work is to evaluate the potential of the additive manufacturing (AM) method robocasting to produce nanostructured zirconia pieces suitable for dental applications. This technique involves the extrusion and layer-by-layer deposition of a ceramic paste according to a digital 3D model [ ]. As far as the authors know this is the first time that a in-depth characterization of robocasting AM processed zirconia pieces for dental applications is made. The benchmark are zirconia samples processed by classical subtractive manufacturing processes (SM) typically used in dental applications. Microstructural and surface characterization of both type of samples, as well as of their wettability and mechanical and tribological properties was performed. The influence of glazing was also accessed. Finally, the biocompatibility of both types of pieces relatively to fibroblast cells was evaluated.
2
Materials and methods
2.1
Materials
Yttria (3% mol) -stabilized zirconia powder Zpex from Tosoh was used to produce the paste to manufacture AM samples. Besides, organic corn syrup (La Finestra Sul Cielo), d (-)-fructose EPR Ph. Eur. (Labkem) and Zusoplast C92 (Zschimmer and Schwarz) which were used as plasticizers and stabilizers, and Dolapix CE 64 (Zschimmer and Schwarz) as dispersant. Samples washing solution was prepared with Extran (Merck). In the case of SM samples, yttria (3% mol) -stabilized zirconia blocks Ice Zirkon Translucent from Zirkonzahn were used.
A glaze ceramic paste (IPS e.max Ceram Glaze Paste 3 g) from Ivoclar Vivadent [ ] was used to coat the zirconia samples.
Dental cusps were isolated from 8 human permanent healthy molars which were visually inspected to ensure the absence of caries, damages or fillers/sealants. Informed consents were obtained prior to teeth extraction. Chloramine-T trihydrate (VWR, ≥98%) and a fluoride free toothpaste (Colgate) were used for teeth cleaning. A thermosetting phenol formaldehyde resin (Bakelite, Vertex Castapress) was used to immobilize the cusps for wear simulation tests.
Artificial saliva (pH 6.9–7.0), which was used as lubricant in the chewing simulation tests, was prepared using the following reagents (all purchased from Sigma-Aldrich) [ ]: sodium sulphide nonahydrate (Na 2 S•9H 2 O, 0.005 g/L), sodium chloride (NaCl, 0.4 g/L), potassium chloride (KCl, 0.4 g/L), sodium phosphate monobasic dihydrate (NaH 2 PO 4 •2H 2 O, 0.69 g/L), urea (NH 2 CONH 2 , 1 g/L), sodium phosphate dibasic (Na 2 HPO 4 , 0.8 g/L), calcium chloride (CaCl 2 , 0.2 g/L). Distilled and deionized (DD) water, obtained from a Millipore system, was used to prepare both artificial saliva and the ceramic paste for AM.
All chemicals and reagents used in cytotoxicity assays were of highest purity grade and sterile. Dulbecco’s Modified Eagle Medium (DMEM), bovine calf serum, amphotericin B, sodium pyruvate, l -glutamine, phosphate-buffered saline (PBS; 10 mM, pH 7.4), trypsin from bovine pancreas, thiazolyl blue tetrazolium bromide (MTT) were purchased from Sigma Aldrich. Penicillin–streptomycin solution (10,000 U penicillin and 10 mg streptomycin per millilitre in 0.9% NaCl) was obtained from Gibco. The dimethylsulfoxide (DMSO) and Triton-X100 were obtained from Merck. Glutaraldehyde (Merck), cacodylate buffer (AppliChem Panreac), t -butanol (Merck) and ethanol (Chem-lab) were used to prepare the samples with cells for scanning electron microscopy (SEM) analysis.
2.2
Methods
2.2.1
Dental samples preparation
The extracted teeth were washed with water and disinfected by immersion in 1% chloramine -T trihydrate solution, at 4 °C, for one week. Then, the specimens were brushed with toothpaste to remove the bacterial plaque and food scraps. Each tooth was cut in 4 parts, so that 4 cusps could be isolated and used for the wear tests in the chewing simulator. Cusps were stored in DD water at 4 °C, until use, to avoid dehydration.
2.2.2
Zirconia samples production
Two methods were used to obtain the zirconia samples: subtractive manufacturing (SM) and additive manufacturing (AM).
Commercial yttria-stabilized zirconia blocks were milled to produce SM samples (15 × 15 × 4 mm 3 , except for biocompatibility tests where cubes with 4 × 4 × 4 mm 3 were used), using a Zirkonzahn M5 milling unit. After the milling process, the samples were cleaned using dry compressed air and were sintered in an oven (Zirkonofen 700 Ultra-Vakuum). The sintering cycle consisted of a heating step of 3 h, a plateau of 2 h at 1500 °C and finally a cooling step of 3 h.
AM samples were produced by robocasting using a paste with the following composition: 350 g of ZrO 2 , 75.6 g of water, 8.75 g of corn syrup, 10.5 g of fructose, 0.84 g of Zusoplast C92 and 0.105 g of Dolapix CE 64. 3D CAD models of plates (15 × 15 × 4 mm 3 , except for biocompatibility tests where, as for SM, cubes with 4 × 4 × 4 mm 3 were used) were obtained through the 123D Design software. Cura software was used to generate the GCODE code to set the printer (Delta WASP 2040 with Ceramic Extruder kit). The samples were printed at room temperature using the following parameters: 60 mm/s, nozzle size 0.4 mm, printed layer height 0.2 mm. After printing, the green samples were air dried overnight and then grinded with a P-600 SiC sandpaper to remove surface features associated to layer-by-layer deposition. Samples were submitted to a sintering cycle which included a pre-heating till 400 °C at a rate of ≈0.6 °C/min, a debinding plateau at 400 °C for 1 h, a second heating step till 1500 °C at a rate of ≈0.9 °C/min, a plateau at 1500 °C during 2 h and finally a cooling step at a rate of ≈5 °C/min. After sintering, AM samples were polished until diamond paste 3 μm in order to obtain an average surface roughness (R a ) similar to SM samples.
Both AM and SM samples were washed for 5 min in an ultrasonic bath with a 15% Extran aqueous solution and then with DD water for 10 min, dried with nitrogen gas, submitted to vacuum overnight and finally stored in a desiccator.
Part of the AM and SM samples were manually coated with a ceramic glaze paste. After glazing, the samples were ovened at 930 °C during 30 min.
2.2.3
Samples characterization
2.2.3.1
Microstructure and topography
Grazing incidence X-ray diffraction (GIXRD) was performed using a Bruker D8 Discover diffractometer with Kα 1,2 radiation (wavelength 1.54 A°), a Göbel mirror and a 0.6 mm slit in the primary beam and a Soller slit and scintillation detector in the secondary beam, at 40 kV and 40 mA. The scanning was performed with a step size of 0.02°, a fixed incidence angle (α) of 1° and dwell time of 15 s in the range between 2θ = 25–70°. Zirconia samples (SM and AM) were analysed before and after glazing. The software Match! was used to visualize the diffractogram of each sample. The crystallites size was estimated using the Debye-Scherrer equation [ ]:
t=KλBcosθB
where <SPAN role=presentation tabIndex=0 id=MathJax-Element-2-Frame class=MathJax style="POSITION: relative" data-mathml='t’>?t
t
is the size of the crystalline domains, <SPAN role=presentation tabIndex=0 id=MathJax-Element-3-Frame class=MathJax style="POSITION: relative" data-mathml='K’>?K
K
is a dimensionless shape factor (default value of 0.9), <SPAN role=presentation tabIndex=0 id=MathJax-Element-4-Frame class=MathJax style="POSITION: relative" data-mathml='λ’>?λ
λ
is the X-ray wavelength, <SPAN role=presentation tabIndex=0 id=MathJax-Element-5-Frame class=MathJax style="POSITION: relative" data-mathml='B’>?B
B
is the line broadening at half of the maximum intensity, and <SPAN role=presentation tabIndex=0 id=MathJax-Element-6-Frame class=MathJax style="POSITION: relative" data-mathml='θB’>θ?θB
θ B
is the Bragg angle.
Topographical images of AM and SM samples were obtained using an atomic force microscope (AFM) Nanosurf Easyscan 2. Images of 10 × 10 μm 2 were obtained in contact mode, at room temperature, using a silicon probe with an applied force of 25 nN and at a scan rate of 1 Hz. The average surface roughness of the samples was determined at different scales using two methods: S a , based on the whole area of the previously referred AFM images; and R a , based on surface 2D profiles obtained using a surface roughness tester (SRT, Mitutoyo 178-923-2A/SJ-201 SurfTest model). In the last, sample lengths of 1.75 mm, with a cut-off of 0.25 mm were scanned. Four samples of each type were analysed (three scans/sample).
Samples surface and cross-cut morphology was analysed by scanning electron microscopy (SEM). To obtain the cross-cut images, samples were cut with a diamond disc and polished up to 3 μm. Images were obtained using the JEOL scanning electron microscope (JSM-7001F) with a voltage of 15 kV and a vacuum of ∼ 8 × 10 −4 Pa. Energy dispersive spectroscopy (EDS) was used to identify the chemical elements present in the samples. The samples were previously sputter-coated with a thin film of Au/Pd.
2.2.3.2
Density and porosity
Density measurements were performed through the Archimedes method. The measurements were done on a AND GR-200 balance, which includes the proper density determination kit. Five samples of each type were used.
The porosity of samples surface and cross-cut sections was estimated from the analysis of five SEM images for each sample using the ImageJ software.
2.2.3.3
Wettability
Water contact angles were determined through the sessile drop method. Measurements were performed at room temperature on previously dried samples (vacuum, 24 h), using a goniometer Ramé-Hart 100-07-00 with a video camera (JAI CV-A50) coupled to a microscope (WildM3Z). Microdrops of DD water were generated on the surface samples with a micrometric syringe inside a testing chamber saturated with water, to avoid evaporation. For each drop, images were taken at pre-established times during 10 min. The acquisition and analysis of the images was performed using the ADSA-P software (Axisymmetric Drop Shape Analysis-Profile [ ]. At least ten drops were done on each material.
2.2.3.4
Hardness and toughness
The microdurometer HSV-30 Shimadzu was used to measure Vickers hardness on the surface and on the cross-cut section of AM and SM samples. For comparison purposes, measurements were also performed on glaze surface. A load of 294.21 N with a dwell time of 15 s was applied on the samples surface. Four samples of each type were analysed. For each sample, five indentations were performed in different locations. The indentations on the samples induced the formation of cracks whose length was measured from SEM images using the ImageJ software. Fracture toughness was estimated through the equation [ , ]:
KIC=0.0726×Pc3/2
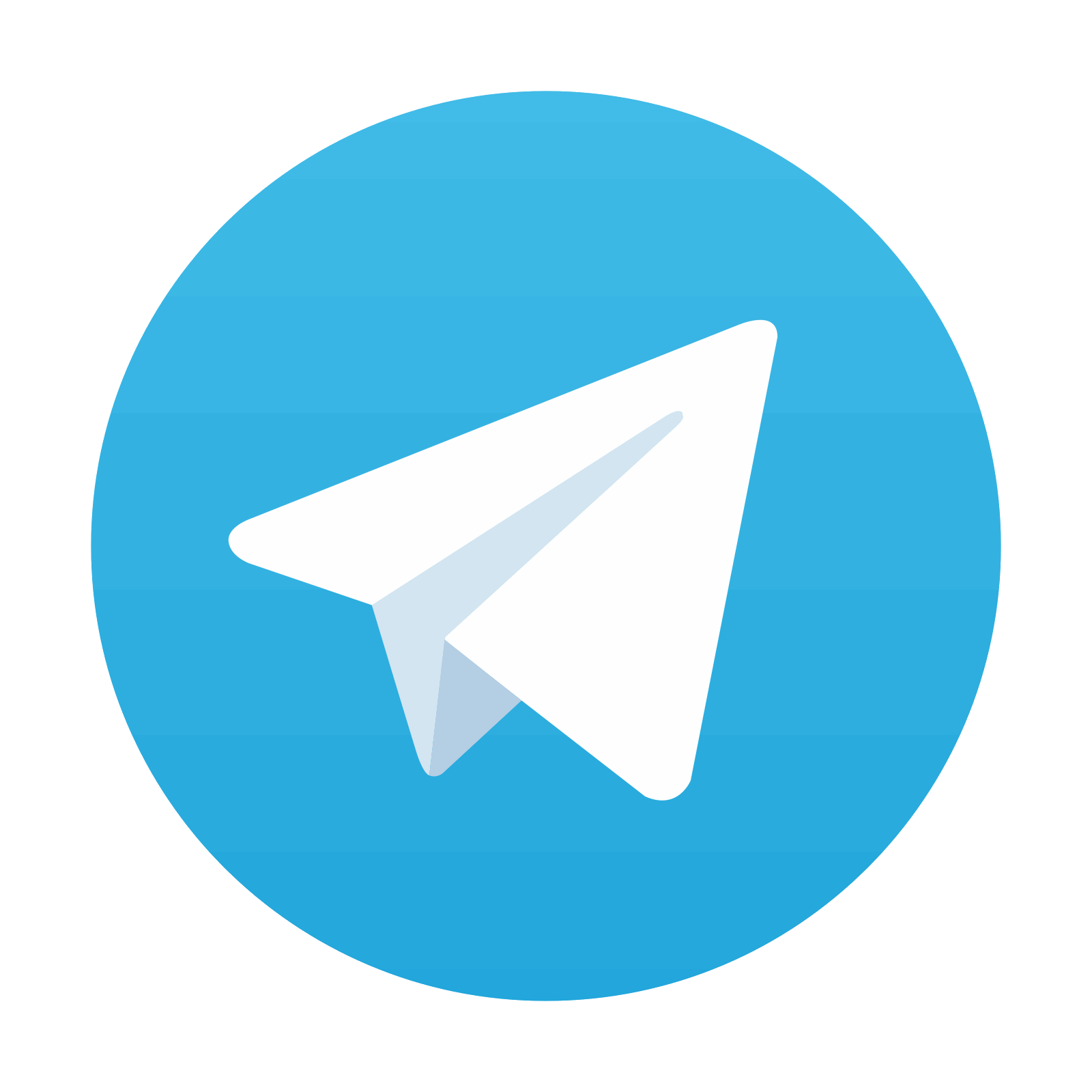
Stay updated, free dental videos. Join our Telegram channel

VIDEdental - Online dental courses
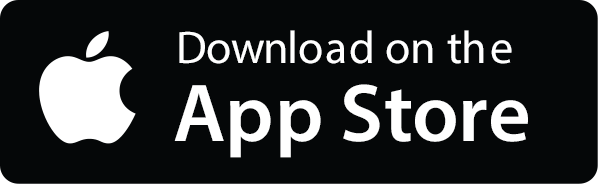
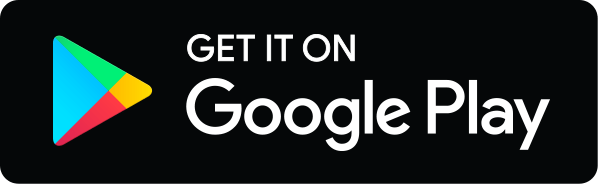
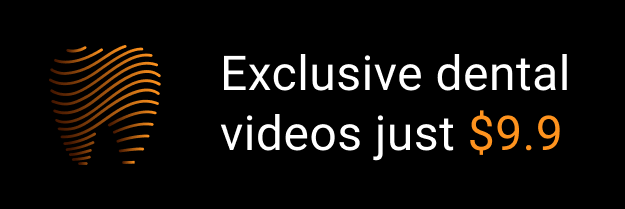