Graphical abstract

Abstract
Objective
This work focuses on the influence of poly(acrylic acid) (PAA) architecture (linear or branched) on setting behavior and compressive strength of glass ionomer cements (GICs).
Methods
Branched and linear poly(acrylic acid)s were synthesized according to the Strathclyde methodology or by free radical polymerization. They were characterized by 1 H-NMR spectroscopy and size exclusion chromatography to determine their molecular weight and size distribution. GIC setting was characterized by oscillating rheometry and time-dependent FTIR spectroscopy. In addition, compressive strength was tested on cylindrical samples (6 × 4 mm; n = 8/cement composition) after storage in deionized water at 37 °C for one day.
Results
We used two different routes to prepare PAA. One direct route in order to provide straightforward access to branched PAA and a two-step approach in order to get more control about the PAA molecular weight using tert- butyl acrylate ( t BA) for polymerization with subsequent deprotection. Using the second approach we obtained several linear PAA of which a mixture was used in order to mimic the molecular weight and size distribution of branched PAA. This allowed the direct comparison of properties relying only on the polymer architecture. Comparing linear PAA to branched samples in general led to faster setting but at the same time decreased the compressive strength. Increasing molecular weight of branched PAA resulted in even faster GIC setting while increasing compressive strength and this correlates well with the trends reported for linear PAA in literature. Mixing of branched and linear PAA, however, turned out to be an effective way of tailoring GIC properties. Significance: our results suggest that both molecular weight and dispersity need to be considered when choosing suitable PAA architecture for obtaining specific GIC properties.
1
Introduction
Glass ionomer cements (GICs) were discovered by Wilson and Kent in the late 1960s, and they are clinically used in restorative dentistry for example as liners, bases or fillers [ , ]. These cements are formed by the reaction of a polymeric acid, such as poly(acrylic acid) (PAA), and an acid-degradable aluminosilicate glass. Protons of PAA react with the aluminosilicate network, leading to the release of cations (e.g. Ca 2+ , Al 3+ ) owing to the breakdown of the glass network by hydrolysis of Si OA l bonds and possibly also an ion-exchange reaction. Setting of the cement occurs owing to the formation of ionic bridges between the released cations and the resulting carboxylate (COO − ) groups [ , ]. Numerous studies were performed to investigate how polymer properties affect GIC setting and properties, and an increase in molecular weight (M w ) typically improves GIC mechanical properties [ ]. However, increasing M w also results in a dramatic increase in the viscosity of the initial mixtures and may therefore negatively affect GIC mixing. Increasing the acid concentration up to 50% leads to improved mechanical properties [ , ]. Alternatives to PAA as polymeric acid have been investigated for the use in GICs (setting and mechanical properties) such as poly(vinylphosphonic acid) where a decrease in working and setting times but an increase in compressive strength has been reported [ ].
The load regimes of GICs used intraorally are more complex than simple compression and, hence, standards were developed to evaluate material properties under laboratory conditions but these do not necessarily mimic service conditions [ ]. The ISO standard for water-based cements such as GICs requires a compressive strength of at least 70 MPa when used as a liner or bases and 150 MPa for the use as direct restoration material [ ]. Commercially available GICs exceed the minimal values and typically show compressive strength between 220 and 300 MPa with short setting times [ ].
Up to now, the effect of PAA architecture on GIC formation and mechanical properties has only been studied to a limited extent. It was shown that the use of dendrimers improved the mechanical properties of commercial GICs [ ], but these are often synthetically demanding. By comparison, access to branched polymers is more straightforward and such materials have been first described by Flory in 1941 [ ]. They typically show lower glass transition temperatures [ ], a larger amount of functional endgroups [ ], lower solution and melt viscosity [ ], and improved solubility [ ] compared to linear polymers with comparable molecular weight. All these properties depend on the degree of branching. With regard to a future clinical application of GICs the effect of branched polymeric acids on setting time due to lower solution viscosity is probably most interesting, as an increase in working time and therefore an improvement of the cement handling can be anticipated.
As already stated above, the synthesis of (hyper)branched polymers is typically straightforward [ ] and easily scalable, rendering such materials interesting candidates for potential applications in biomedicine [ ], gene delivery [ ], or as viscosity modifiers [ ]. Synthetic access to branched vinyl polymers was enabled by self-condensing vinyl polymerisation (SCVP), first reported in 1995 by Frechet et al. [ ]. Here, inimers, i.e. initiator containing monomers, were used to form branched polymers by controlled radical polymerization techniques such as NMP [ ], RAFT [ ], ATRP [ ], cationic [ ] or anionic polymerization [ ]. This also resulted in the first synthetic preparation of well-defined branched poly(acrylic acids) [ ]. In 2000, Sherrington et al. [ ] described a facile and flexible procedure to form long-chain branched vinyl-containing polymers by a simple free-radical copolymerisation of a vinyl monomer and a multifunctional crosslinker, also known as Strathclyde methodology. To prevent macroscopic gelation, a defined amount of chain transfer agent (CTA), such as alkane thiols or cobalt complexes, was added [ ]. Besides the formation of branched poly(methacrylic acid) (PMAA) [ ] also acrylic acid (AA) [ ], methyl methacrylate (MMA) [ ], styrene [ ], vinyl acetate (VAc) [ ], dimethylacrylamide (DMA) [ ], butyl acrylate (n/tBA) [ ] or even vinyl-functionalized glycomonomers could be used in a one-pot reaction to form branched polymers [ ].
Here we present two different synthetic routes to branched PAA based on the Strathclyde methodology. The polymers are investigated by SEC and 1 H-NMR-spectroscopy and designed in a way that the apparent molecular weight and especially the molecular weight distribution of both linear and branched PAA was comparable. With these polymers on hand we were aiming to study the effect of PAA architecture (linear vs. branched, as well as variation in molecular weight and disperstiy) on the setting and compressive strength of experimental GICs. Well known and already commercially used glass LG26 was used as glass component for cement preparation.
2
Materials and methods
2.1
Materials
All chemicals were used as received if not otherwise stated. The monomers acrylic acid (AA, Alfa Aesar, 99.5%), tert -butyl acrylate ( t BA, Acros, 99%) and tri(propylene glycol) diacrylate (TPGDA, Sigma Aldrich, mixture of isomers) contained monomethyl ether hydroquinone or methoxyphenol as inhibitor, which was removed by column chromatography over aluminium oxide prior to use.
Aluminosilicate glass LG26 [ ] (4.5 SiO 2 –3 Al 2 O 3 –1.5 P 2 O 5 –3 CaO–2 CaF 2 , in mol% d50 = 18.9 μm) was kindly donated by First Scientific Dental GmbH, Elmshorn, Germany, and used without further purification.
2.2
Synthesis of linear and branched poly( tert -butyl acrylate) (P t BA)
A reaction vial was charged with t BA and a magnetic stirrer. A stock solution of TPO, TPGDA and DDT in tetrahydrofuran (THF) was prepared and added. Additional THF was added so that the total volume was 45 mL. The vial was sealed and degassed by bubbling argon through the solution for 30 min, before being exposed to intense UV irradiation for 15 min. THF (3 mL) was added to the solution directly afterwards. The polymer was precipitated in into a 10-fold excess of 50 vol% methanol/H 2 O and re-dissolved in acetone, transferred and dried under reduced pressure.
1 H NMR (300 MHz, acetone D 6 (Deutero)) δ [ppm] = 2.34 (m, 2H, CH 2 ), 1.9 (m, 1H, backbone), 1.5 (s, 9H, tert- butyl).
SEC (DMAc + 0.21% LiCl; PMMA-calibration): P t BA L 20 : M n =11.7 kg mol −1 , M w = 20.0 kg mol −1 , Đ = 1.7; P t BA L 31 : M n =18.1 kg mol −1 , M w = 31 kg mol −1 , Đ = 1.7 P t BA B 23 : M n = 6.4 kg mol −1 , M w = 22.9 kg mol −1 , Đ = 3.6; P t BA B 36 : M n = 14 kg mol −1 , M w = 35.5 kg mol −1 , Đ = 2.5.
2.3
Deprotection of poly( tert -butyl acrylate)
The respective P t BA was dissolved in dichloromethane (DCM) (m(P t BA)[g]:v(DCM)[ml] = 1:6) and trifluoroacetic acid (TFA) (m(P t BA):m(TFA) = 0.6:1) is added. The solution was stirred over night at 60 °C, during which time deprotected PAA precipitates. The supernatant was decanted and the obtained product was washed 3 times with DCM to remove TFA and afterwards dissolved in dioxane and freeze dried.
1 H NMR (300 MHz, acetone D 6 (Deutero)) δ [ppm] = 2.34 (m, 2H, CH 2 ), 1.9 (m, 1H, backbone).
SEC (0.08 M Na 2 HPO 4 /0.05% NaN 3 pH = 9; PAA-calibration): PAA L 23 : M n =5.5 kg mol −1 , M w = 22.8 kg mol −1 , Đ = 4.2; PAA L 38 : M n = 8.2 kg mol −1 , M w = 38.2 kg mol −1 , Đ = 4.7 PAA B 25 : M n = 4.2 kg mol −1 , M w = 25.3 kg mol −1 , Đ = 6.0; PAA B 41 : M n = 9.0 kg mol −1 , M w = 41.5 kg mol −1 , Đ = 4.6.
2.4
Synthesis of branched poly(acrylic acid) (PAA B )
TPGDA, DDT, 80 mg TPO and 50 g AA were dissolved in 150 mL of THF. To remove oxygen, dry argon was bubbled through the solution. After 30 min, the reaction mixture was exposed to UV light for 20 min, to start polymerization. After cooling to room temperature, the polymer was precipitated in cold diethyl ether, filtered and dried.
1 H NMR (300 MHz, DMSO D 6 (Deutero)) δ [ppm] = 2.3 (m, 1H, CH), 1.83 – 1.53 (m, 2H, CH 2 ).
SEC (0.08 M Na 2 HPO 4 /0.05% NaN 3 pH = 9; PAA-calibration): PAA B 3 : M n = 0.83 kg mol −1 , M w = 3.3 kg mol −1 , Đ = 4.0; PAA B 12 : M n = 1.9 kg mol −1 , M w = 12.2 kg mol −1 , Đ = 6.3 PAA B 22 : M n = 1.5 kg mol −1 , M w = 22 kg mol −1 , Đ = 15; PAA B 47 : M n = 1.9 kg mol −1 , M w = 47 kg mol −1 , Đ = 24.8.
2.5
Nuclear magnetic resonance (NMR) spectroscopy
Proton nuclear magnetic resonance ( 1 H NMR) spectra were recorded on a 300 MHz Bruker Fourier300 Avance spectrometer at 298 K. Chemical shifts are reported in parts per million (ppm) relative to the NMR solvent signals. As deuterated solvents, d 6 -Acetone, CDCl 3 and d 6 -DMSO (Deutero) were used.
2.6
Size exclusion chromatography (SEC)
Size-exclusion chromatography was performed on two different systems. Poly( tert -butyl acrylate) samples were measured in N,N -dimethylacetamide (DMAc) using an Agilent1200 system combined with a G1310A pump, a G1362A refractive index detector and both a PSS Gram30 and a PSS Gram1000 column in series (separation range 374–1,040,000 Da). N,N -dimethylacetamide with 5 mmol lithium chloride was used as eluent at a flow rate of 1 mL min −1 at 40 °C. The SEC system was calibrated against low dispersity poly(methyl methacrylate) (PMMA).
Water-soluble PAA samples were measured on a JASCO system (JASCO Corporation, Tokyo, Japan) equipped with a PU-980 pump, an AS-2051 Plus autosampler, and a RI-2031 Plus refractive index detector. Separation was performed on SUPREMA 30 Å and 1000 Å columns (PSS, Mainz, Germany) at 30 °C in water containing 0.08 M Na 2 HPO 4 and 0.05% NaN 3 at a flow rate of 1 mL min −1 . Here, the SEC system was calibrated using PAA standards (1,250–782,200 g mol −1 ), which were purchased from PSS.
2.7
Glass ionomer cement preparation
GICs were prepared by hand mixing glass LG26 powder with PAA (linear or branched) powder and de-ionised water in a weight ratio of 4:1:1 on a plastic plate using a plastic spatula. GICs were prepared using linear or branched PAAs with different molecular weights ( Table 1 ) or a mixture of linear PAAs (PAA L 23 and PAA L 38 ). In addition, mixtures of PAA L 58 with branched PAA (PAA B 41 or PAA B 47 ) were prepared to investigate dispersity effects ( Đ ).
Sample | Composition (molar ratio) | Before deprotection | After deprotection | ||||||
---|---|---|---|---|---|---|---|---|---|
t BA | TPGDA | CTA | TPO | M w [a] [kg mol −1 ] | Ð [a] | M w [b] [kg mol −1 ] | Ð [b] | Residual tert-butyl groups [%] | |
Linear | |||||||||
PAA L 23 | 60 | – | 0.1 | 0.06 | 20 | 1.7 | 22.8 | 4.2 | 11 |
PAA L 38 | 60 | – | 0.1 | 0.01 | 31 | 1.7 | 38.2 | 4.7 | 3 |
PAA L 60 | – | – | – | – | – | – | 59.9 | 3.8 | |
PAA L Mix | – | – | – | – | – | – | 40.6 | 5.0 | |
Branched | |||||||||
PAA B 25 | 60 | 1 | 5 | 0.01 | 22.9 | 3.6 | 25.3 | 6.0 | 19 |
PAA B 41 | 60 | 1 | 0.1 | 0.09 | 35.5 | 2.5 | 41.5 | 4.6 | 29 |
AA | |||||||||
PAA B 3 | 60 | 1 | 3 | 0.02 | – | – | 3.3 | 4.0 | – |
PAA B 12 | 60 | 1 | 1 | 0.02 | – | – | 12.2 | 6.3 | – |
PAA B 22 | 60 | 1 | 0.8 | 0.02 | – | – | 22 | 15.0 | – |
PAA B 47 | 60 | 1 | 0.6 | 0.02 | – | – | 47 | 24.8 | – |
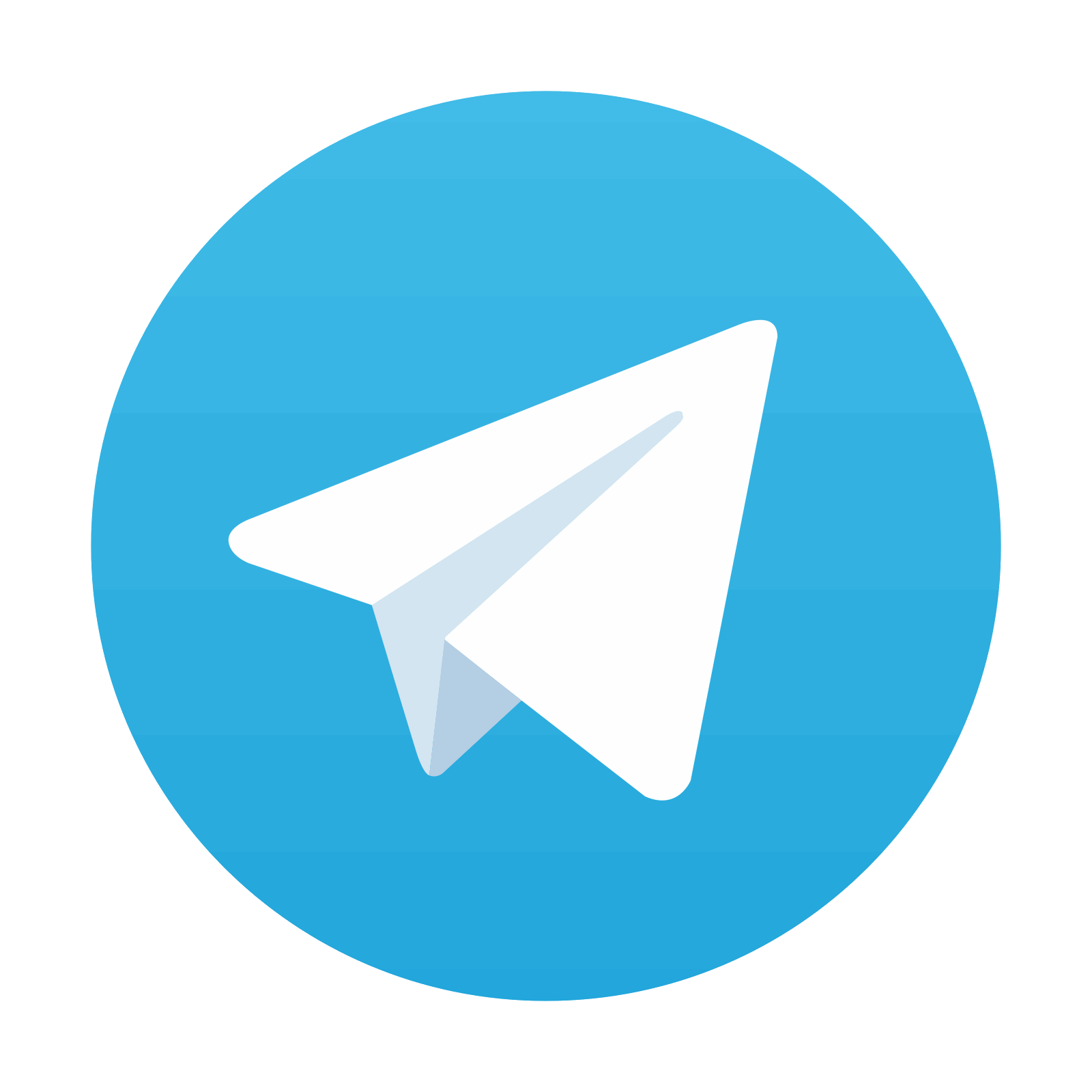
Stay updated, free dental videos. Join our Telegram channel

VIDEdental - Online dental courses
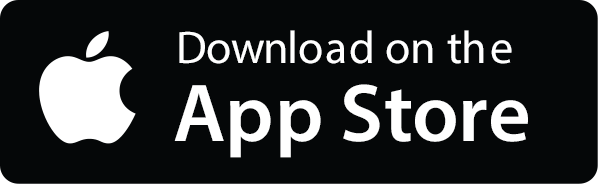
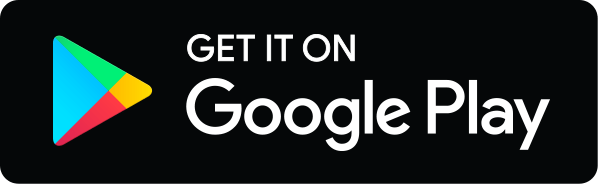
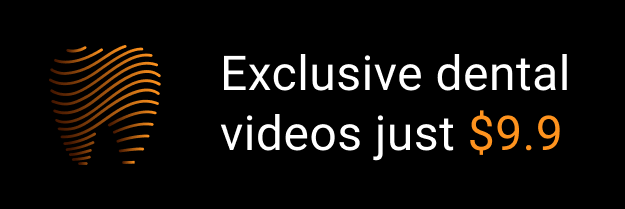