Introduction
Orthodontic tooth movement causes pain to a patient. Glial cells are nonneuronal cells in the central nervous system and are implicated in various types of pain. In this study, we assessed glial activation responses after experimental tooth movement using immunocytochemical detection of anti-CD11b (OX42) and glial fibrillary acidic protein immunoreactivity to illustrate the microglial and astrocytes response, respectively. In addition, the effect of minocycline in reducing pain during tooth movement was also investigated.
Methods
Fifty-five Sprague Dawley rats with and without administration of minocycline after 1, 3, 5, 7, and 14 days (n = 5, for each) of tooth movement were used. Immunohistochemistry for microglia (OX42) and astrocyte (glial fibrillary acidic protein) were performed at the medullary dorsal horn (trigeminal subnucleus caudalis). Three-dimensional quantitative analysis was performed with a confocal fluorescence microscope and a software program.
Results
There was a significant increase in the OX42 and glial fibrillary acidic protein immunoreactivity in response to tooth movement in the medullary dorsal horn. Furthermore, systematic administration of minocycline, a selective inhibitor of microglial activation, significantly attenuated the nociceptive c-Fos expression in the medullary dorsal horn that was induced by experimental tooth movement.
Conclusions
These data indicate the possible importance of microglial activation in the development of orthodontic pain. This is also the first report on the systematic application of minocycline.
Highlights
- •
Microglia and astrocytes may play roles in pain transmission during tooth movement.
- •
Minocycline administration selectively inhibited microglial and c-Fos expression.
- •
Minocycline has the potential to control pain during orthodontic treatment.
Orthodontic force causes continuous pressure on the teeth and supporting structures that induces pain. Significant pain tends to appear normally about half a day later and then lasts for a few days, indicating that orthodontic pain is different from immediate acute pain. Orthodontic patients feel discomfort, and 95% of them experience varying degrees of discomfort during treatment.
Glial cells are nonneuronal cells in the central nervous system (CNS) that have a role in the initiation and maintenance of persistent pain states. Microglia and astrocytes have been implicated in various types of pain such as subcutaneous inflammation and peripheral nerve injury. Microglia are the early responding glial cells in the CNS after injury, and microglia release products that activate astroglia. Furthermore, both microglia and astrocytes have the ability to release proinflammatory cytokines. These proinflammatory cytokines and prostaglandins released after microglial activation play a role in central sensitization and are implicated in exaggerated pain states.
Because orthodontic pain is initiated by an inflammatory-like response in the periodontal ligament, nonsteroidal anti-inflammatory drugs are the preferred over-the-counter medications for orthodontic pain. The major concern regarding these drugs is the interference produced on inflammation associated with tooth movement. Since nonsteroidal anti-inflammatory drugs cannot cross the blood-brain barrier, they directly react with the peripheral tissues. The current trend is directed toward use of preemptive or preoperative analgesics, which are administrated at least 1 hour before every orthodontic procedure. Preemptive analgesia blocks the afferent nerve impulses before they reach the CNS, abolishing the process of central sensitization. There are only a few suggested preemptive drugs, most of which inhibit bone resorption and interfere with tooth movement. Orthodontic pain characteristically is long lasting but is not persistently acute. Thus, preemptive analgesia targeting the brain or brainstem might relieve the orthodontic pain efficiently. It may be productive to develop a new preemptive analgesia that blocks neuronal hyperactivity input to the CNS that would reduce pain or discomfort without tooth movement side effects.
Minocycline is a semisynthetic tetracycline derivative that exerts anti-inflammatory effects completely distinct from its antimicrobial action. Minocycline is a lipophilic molecule and readily crosses the blood-brain barrier and selectively disrupts the activation of microglia without directly affecting neurons or astroglia. Minocycline’s antihyperalgesic and antiallodynic effects have been demonstrated in models of arthritis, spinal nerve transection, and sciatic inflammatory neuritis. Interestingly, minocycline has no effect on acute inflammation and attenuates and delays the development of neuropathic pain and formalin-induced inflammatory pain responses. Lasting nociception induced by experimental tooth movement may lead to glial activation at the medulla, and glial inactivation by minocycline medication should show antineuronal activity.
In this study, anti-CD11b (OX42) and glial fibrillary acidic protein (GFAP) were used as glial cell markers and for microglial and astrocytic responses, respectively. Proto-oncogene c-Fos, known as a marker for nociceptive pain, was also used for double immunostaining with glia markers.
Our hypothesis was that there would be no significant difference in the OX42 immunoreactivity, GFAP immunoreactivity, and c-Fos immunoreactivity with and without the application of minocycline after experimental tooth movement in rats.
Material and methods
The animal committee of the Graduate School of Medicine and Dentistry at Okayama University in Japan approved the experimental protocol.
Fifty-five adult Sprague Dawley rats (weight, 150-200 g; CLEA Japan Inc., Tokyo, Japan) (5 control; 25 with minocycline; 25 without minocycline) were used for the study. For the tooth movement, the rats were anesthetized with a mixture of pentobarbital sodium (20 mg/kg) and ethylcarbamate (650 mg/kg), and a piece of an orthodontic elastic module was inserted between the maxillary first and second molars on the right side ( Fig 1 , A ). After 1, 3, 5, 7, and 14 days (each group, n = 10) after tooth movement, the rats were anesthetized by inhalation to a depth at which respiration was markedly suppressed and then transvascularly perfused with 50 mL of saline solution followed by 500 mL of 4% formaldehyde in 0.1 mol per liter of sodium phosphate buffer (pH, 7.4). The lower brain stem was dissected out, immersed in the same fresh fixative overnight, and then cryoprotected by immersion in 20% sucrose in 0.02 mol per liter of phosphate-buffered saline solution at 4°C. The frozen sections were embedded in an optimal cutting temperature compound and cut frontally (50 μm thick).
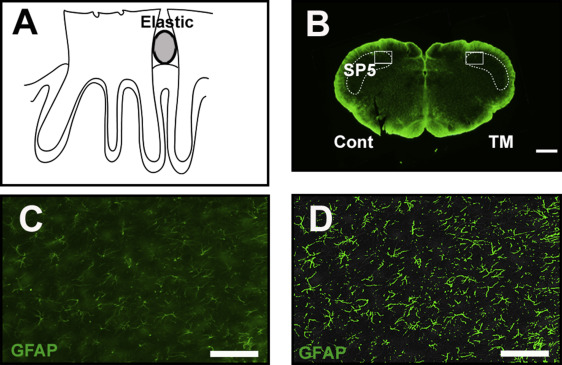
In 25 rats, minocycline was administrated during tooth movement. Minocycline (Sigma-Aldrich, St Louis, Mo) was dissolved in sterile water and sonicated to ensure complete solubilization. The rats received an intraperitoneal injection of vehicle or minocycline (50 mg/kg) once a day during the experimental period. They were transvascularly perfused followed by dissection 1, 3, 5, 7, and 14 days after tooth movement.
For the immunohistochemistry, microglia were identified by OX42 (mouse anti-rabbit, 1:1000; Sigma-Aldrich), which recognizes cell surface complement receptor 3. Astrocytes were identified by anti-GFAP (mouse monoclonal, 1:5000; Dako, Glostrup, Denmark), which is an intermediate filament protein predominantly expressed in cells of astroglial origin. The synthesis of this protein is activated by injury or stimuli. c-Fos immunoreactivity was also analyzed to evaluate the orthodontic nociception at the medulla (1:10,000; Oncogene Science, Cambridge, Mass). The avidin-biotin-horseradish peroxidase complex method was used, and all sections were incubated with 1% bovine serum albumin (Amersham International, Amersham, United Kingdom) and 0.3% Triton-X 100 (Sigma-Aldrich) in phosphate-buffered saline solution for 1 hour at room temperature. Sections were then incubated overnight at 4°C with an OX42, GFAP, or c-Fos antibody. The sections were developed using a mixture of Alexa Fluor 594-conjugated goat anti-rabbit IgG (Invitrogen, Carlsbad, Calif) and Alexa 488-conjugated goat anti-mouse IgG (Invitrogen). Some sections were exposed to avidin-biotin-horseradish peroxidase complex in 0.1 mol per liter of PBST for c-Fos immunostaining for quantitative analysis. The antigens were visualized by reaction with 0.05% 3,3-diaminobenzidine tetrahydrochloride in phosphate-buffered saline solution and 0.003% hydrogen peroxide for 5 minutes. The immunoreactions were enhanced with nickel ammonium sulfate. The immunostained sections were mounted on gelatin-subbed glass slides, dehydrated through an ascending series of ethanol, cleared with xylene, and covered with coverslips. The specificity of antibodies used in this study has been described elsewhere.
We evaluated quantitatively the immunoreactivities of OX42, GFAP, and c-Fos. The sections were examined with a fluorescence microscope (Bx-51; Olympus, Tokyo, Japan). The photographs were taken with a charge-coupled device camera (DP72; Olympus). The images were saved in TIFF format and managed by a DP manager (Olympus). OX42 and GFAP immunoreactivities were evaluated in the rostralmost sections through the medullary dorsal horn (trigeminal subnucleus caudalis). The outlines of the medullary dorsal horn were first determined with dark-field illumination ( Fig 1 , B ). These regions were also evaluated with calcitonin gene related peptide immunoreactivity to confirm that these regions were involved in pain processing. The size distribution of the OX42 and GFAP immunoreactivities in the medullary dorsal horn was measured with WinROOF software (Mitani, Fukui, Japan) in each region of interest of 400 × 300 × 50 μm ( Fig 1 , B – D ). The measured cross-sectional areas were given as the means and standard deviations.
The numbers of c-Fos immunoreactivity neurons were counted in the rostralmost 3 sections through the medullary dorsal horn. The images were processed, and the immunoreactive cells were also quantified with the WinROOF software program. All measurements were performed 3 times and were blinded, and we used the average value for the results.
Statistical analysis
The statistical analysis was performed using the SigmaStat software package (StatView, version 3.1; SPSS, Chicago, Ill). Individual means of OX42 immunoreactivity were determined by averaging intensities from 10 to 12 sections for each animal before the means and standard errors were calculated for each group (n = 5 per group). The difference between groups for OX42, GFAP intensity, and c-Fos immunoreactivity counting were compared using 2-way analysis of variance followed by the Tukey post hoc test. The alpha level was set at 0.05 in all cases.
Results
For the glial activation by experimental tooth movement, the controls (without the elastic module or tooth movement) showed low bilateral expressions of OX42 and GFAP immunoreactivity in the medullary dorsal horn. The OX42 and GFAP immunoreactivities did not appear to change over the postmanipulation intervals. The experimental group with the elastic module and tooth movement had significantly increased OX42 and GFAP immunoreactivities on the ipsilateral side of the medullary dorsal horn at 3 to 7 days after the experimental tooth movement began ( Figs 2 and 3 ) with a peak at 5 days ( Figs 2 , B and E , and 3 , B and E ). However, there was no significant change on the contralateral side of the medullary dorsal horn in the OX42 and GFAP immunoreactivities throughout the entire experimental period.
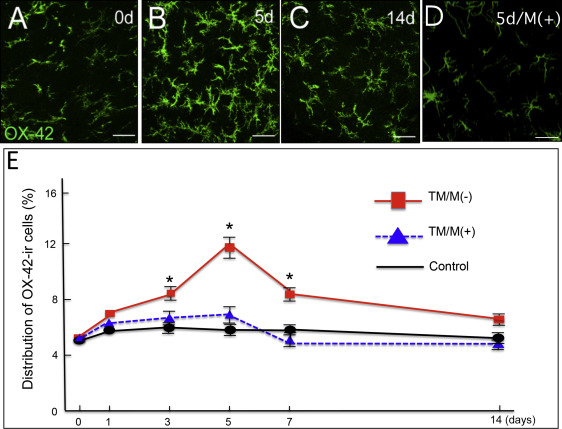
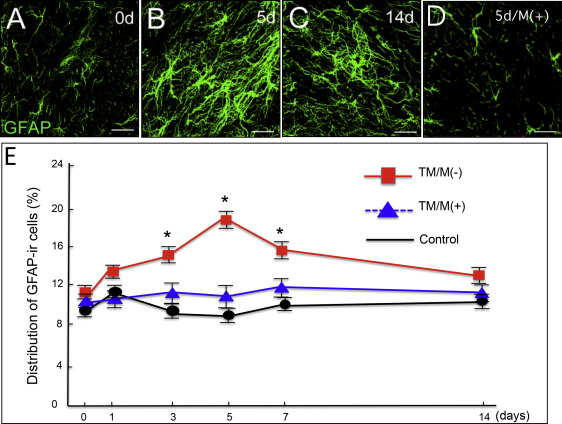
For the attenuation of the orthodontic nociception by minocycline application, only a few c-Fos immunoreactivity neurons were observed in the controls ( Fig 4 , A ). Experimental tooth movement increased c-Fos immunoreactivity in the medullary dorsal horn at 1 day after tooth movement ( Fig 4 , C , and G ). This induction was decreased after 3 days ( Fig 4 , E , and G ) and further decreased after 5 days ( Fig 4 , G ). Administration of minocycline ( Fig 4 , B ) significantly attenuated the tooth movement-induced c-Fos immunoreactivity in the medullary dorsal horn at 1 day ( Fig 4 , D and G ). Such significant attenuation by minocycline was also observed on day 3 ( Fig 4 , F and G ) and day 5 after the start of the tooth movement ( Fig 4 , G ). Furthermore, minocycline application also significantly decreased the numbers of OX42 immunoreactivity cells ( Fig 2 , D , and E ) and GFAP immunoreactivity cells ( Fig 3 , D and E ).
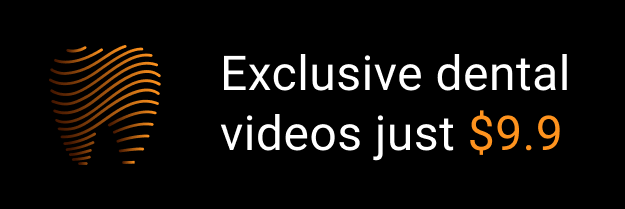