Highlights
- •
Manual mixing with water results in microstructural changes, with lower setting time and lower calcium ion release.
- •
Manual mixing required more liquid added to the mixture to obtain a similar consistency and flow to the control.
- •
Increasing the amount of Biodentine liquid increases the calcium ion release but also increases the setting time.
- •
Following the manufacturer’s instruction for the mixing of Biodentine guarantees the materials characteristics and clinical performance.
Abstract
Objective
The water to powder ratio and method of mixing is important for the properties of hydraulic cements. For this purpose a number of clinicians prefer premixed materials. Dental manufacturing companies provide predosed materials, however the manufacturer instructions are not always adhered to. The aim of this research is to investigate physical and chemical alterations of the tricalcium silicate‐based cement Biodentine when manipulated according to the manufacturer’s instructions (control) or changing the doses and mixing of the material components.
Methods
6 groups were constituted according to different mixing and dosing of powder and liquid. The hydrated cements were characterized using scanning electron microscopy (SEM), X-ray diffraction (XRD) and Fourier transform infrared spectroscopy (FT-IR). Calcium ion concentration of the leachate was also investigated. Assessment of the physical characteristics included setting time and microhardness.
Results
Microstructural differences were visible only in the Biodentine mixed manually with water, in which early hydration rate was also affected, with lower calcium ion release. Increase of Biodentine liquid increased the calcium ion release, but also increased the setting time. Manual manipulation required more liquid (both water and Biodentine liquid) added to the mixture to guarantee a similar consistency to the control. A decrease in setting time was also noted. All groups showed higher values of microhardness at 24 h compared to the freshly set materials. In the freshly set materials, there was an overall decrease in microhardness in all groups when compared to group control, particularly significant when increasing the dosage of Biodentine liquid.
Significance
When mixing Biodentine, altering the mixing procedure in terms of type and amount of liquid added to the powder and mixing device chosen has an effect on the physical, chemical and mechanical characteristics and surface topography of the material, when compared to Biodentine mixed according to the manufacturer’s recommendations. Hence, the manufacturer’s instructions should be strictly followed.
1
Introduction
There are numerous hydraulic calcium silicate cements (HCSCs) currently in use in endodontics, with mineral trioxide aggregate (MTA) having been the first to be introduced and the most extensively studied. MTA is composed of Portland cement and bismuth oxide in an 4/1 ratio [ , ]. In 2009, Septodont (Saint-Maur-des-Fossés, France) introduced Biodentine (BD), an optimized tricalcium silicate-based dental material to be used as a bioactive dentin substitute [ ]. Biodentine is a two component material; powder and liquid. The powder, presented in a capsule, is mainly composed of tricalcium silicate, with a smaller percentage of calcium carbonate, and zirconium oxide as a radiopacifier [ ].The liquid is mostly composed of water but also contains calcium chloride as a setting accelerator and a water reducing agent [ , ].
The material is claimed to possess better physical and biological properties compared to other tricalcium silicate-based cements [ ]. The advantages over MTA include the elimination of trace elements and of aluminum-based phases by using pure tricalcium silicate in its composition [ , ], absence of tooth discoloration by including zirconium oxide instead of bismuth oxide [ ] and a shorter setting time (approximately 10−12 min), as a result of the calcium chloride in the aqueous solution [ , ]. It also shows higher strength when compared to other similar materials thanks to the lower water to cement ratio possible due to the incorporation of the polycarboxylate in the aqueous solution [ , ] and a higher initial rate of calcium ion release [ ].
The mixing and handling characteristics of the powder/liquid system is very technique sensitive and leads to considerable waste [ ]. The manufacturer’s recommended mixing instructions for BD include placing five drops of the liquid in the ampoule (which does not correspond to the total amount of liquid) in the open capsule, and placing the closed capsule in a mixing device available from Septodont (Saint-Maur-des-Fossés, France) at 4000–4200 rotations per minute for thirty seconds [ ].
In an effort to reduce waste, clinicians and dental assistants may be tempted to manipulate BD with some alterations to the recommendations by the manufacturer [ ]. Such alterations may have an impact on the characteristics of the final hydrated material and, thus, its clinical performance [ ].
The aim of this research was to investigate whether altering the manufacturer’s recommendations for the mixing of Biodentine (Septodont, Saint-Maur-des-fosses, France) would affect the material’s physical and chemical properties.
2
Materials and methods
This study evaluated Biodentine (Septodont, Saint-Maur-des-fossés, France) (BD) manipulated in different ways. The six study groups included the following:
-
Group 1 (BD): 1 capsule of BD and 5 drops of liquid, vibrated for 30 s (s) in the BD vibrator (BDV), according to manufacturer’s instructions;
-
Group 2 (BD-A): 1 capsule of BD and 5 drops of BD liquid vibrated for 30 s in the amalgamator (Amg) (Vibracap RS, Paris, France);
-
Group 3 (BD-F): 1 capsule of BD and full amount of BD liquid provided, vibrated for 30 s in the BDV.
-
Group 4 (BD-AF): 1 capsule of BD and full amount of BD liquid, vibrated for 30 s in the Amg.
-
Group 5 (BD-MW): 1 capsule of BD and 6 drops of water manually mixed on a glass plate until a homogeneous consistency (similar to group 1) was obtained.
-
Group 6 (BD-ML): 1 capsule of BD and 6 drops of BD liquid manually mixed on a glass plate until a homogeneous consistency (similar to group control) was obtained.
Cylindrical specimens 6 mm in diameter and 2 mm high were prepared in stainless-steel molds. The BD was mixed in the different ways defined in Groups 1–6 and tested immediately after setting and again after 24 hours (h) kept at 37 °C in Hank’s balanced salt solution (HBSS; Sigma Aldrich, Gillingham, UK). All mixing was done by a single operator (JC). The specimens were characterized to assess whether the changes in dosing and mixing affected the material chemistry and microstructure. This was done by scanning electron microscopy (SEM), X-ray diffraction (XRD) analysis, and Fourier transform infrared (FT-IR) spectroscopy. Leachate analysis was carried out by inductively coupled plasma optical emission spectroscopy (ICP-OES). The effect of modified mixing and dosing on the physical properties was also assessed, including setting time and microhardness.
2.1
Material characterization
The materials were tested immediately after setting and also after 1 day immersion in Hank’s balanced salt solution (HBSS). The characterization by SEM, ATR and one batch for microhardness were tested immeditealy after the materials had achieved their final setting time testing using an indenter. The XRD, calcium ion release and remaining batch for microhardness were assessed after 24 h immersed in 5 mL HBSS and placed into a cabinet at 37 °C.
2.1.1
Scanning electron microscopy
The ultrastructure was assessed by SEM. Immediately after setting, the specimens were vacuum desiccated and progressively polished. The polishing was achieved using silicon carbide grinding paper of grit sizes 1000 (P2500, CarbiMet, Buehler, Esslingen, Germany) and 280 (P320, CarbiMet, Buehler, Esslingen, Germany) under tap water. The specimens were attached to aluminum stubs, gold coated (K550X Sputter coater, Emitech, Sussex, UK) and viewed under the SEM (EVO MA10, Zeiss, Oberkochen, Germany). Scanning electron micrographs were captured of the different material microstructural components at different magnifications — 500, 1000 and 2000 — in backscatter electron mode.
2.1.2
X-ray diffraction (XRD) analysis
Phase analysis was carried out on the set materials using X-ray diffraction analysis. The diffractometer (D8 ADVANCE, Bruker, Massachusetts, USA) used Cu Kα radiation at 40 mA and 40 kV. After 24 h immersion in HBSS the materials were desiccated for 30 min, crushed using a mortar and pestle prior to testing. Samples were presented in powder form and the detector was set to rotate between 5 and 50°, a sampling width of 0.058° and scan speed of 1°/min. Phase identification was accomplished using a search-match software utilizing ICDD database (International Centre for Diffraction Data, Newtown Square, PA, USA).
2.1.3
ATR-Fourier transform infrared (FT-IR) spectroscopy
After final setting the specimens were desiccated for 30 min and afterwards crushed to powder with a mortar and pestle. The resulting powder was analyzed using a Fourier transform infrared spectrophotometer (Nicolet 6700, Thermo Scientific, Tewksbury, USA) using transmitted infrared spectroscopy with frequencies between 4000 and 400 cm −1 (wavenumbers).
2.1.4
Calcium ion release
After setting the specimens were immersed in 5 mL Hank’s balanced salt solution (HBSS, Sigma Aldrich, Gillingham, UK) for 24 h. Calcium ion release was assessed by ICP-OES (Optima 8000, PerkinElmer, Waltham, USA) and determined as the average of three measurements per sample in parts per million (ppm).
2.2
Assessment of physical characteristics
2.2.1
Setting time
The cements were mixed as previously described and placed in a stainless-steel mold of 10 mm inner diameter and 5 mm height and pressed between two glass plates. The assembly was placed into a cabinet (Shake ‘n’ Stack, Hybaid, ThermoFisher Scientific, Massachussets, USA) at 37 °C and relative humidity of 95 ± 5 % for 5 min. An indenter with 100 ± 5 g in weight using a round needle with a flat end, 1 ± 0.1 mm in diameter was used to assess the initial setting time. This indenter was lowered vertically onto the surface of the test cement. This process was repeated every 60 s until the indenter failed to make a visible imprint on the sample surface. At this point, the 100 g indenter was replaced by the 400 g indenter to test for final setting time. The previous procedure was repeated until the indenter failed to make a visible imprint on the sample surface. The initial setting time was defined as the time elapsed between the end of mixing and the time when the 100 g needle failed to make a visible indentation on the test cement; and final setting time was defined as the time elapsed between the end of mixing and the time when the 400 g needle failed to make a visible indentation on the test cement.
2.2.2
Microhardness
Six specimens of each group were mixed according to the previous description. Three were tested after setting and the remaining three were placed in plastic containers with 5 mL HBSS and placed into a cabinet at 37 °C and relative humidity of 95 ± 5 % for 24 h. Microhardness testing (Mitutoyo, Mitutoyo Asia Pacific td. Singapore) was performed using a diamond shaped indenter on polished surfaces. A load of 4,903 N was applied for ten seconds. Vickers Hardness Number (VHN) was recorded from an average of three indentions per specimen.
2.3
Statistical analysis
The data obtained for calcium ion release and microhardness were subjected to statistical analysis using SPSS version 25.0.0 (SPSS Inc, Chicago, IL, USA). The Kolmogorov–Smirnov showed that the data were normally distributed and, therefore, parametric statistical tests were performed (one-way analysis of variance – ANOVA- followed by Tukey’s post-hoc test for multiple comparisons). The level of statistical significance was set at p < 0.05.
Only one specimen for group was tested for setting time, which conveyed insufficient data to perform a statistical analysis other than descriptive.
3
Results
3.1
Material characterization
The scanning electron micrographs ( Fig. 1 ) of all the groups were similar. Microstructural differences were visible only in the Biodentine mixed manually with water (BD-MW). While all the groups exhibited cement particles with the hydration halo, in the BD-MW group no hydration product around the cement particles was visible, indicating a slower hydration process compared to the other groups.

X-ray diffraction analysis is presented in Fig. 2 , where the different phases, namely tricalcium silicate (ICDD: 31-0301), zirconium oxide (ICDD: 37-1484) and calcium carbonate (ICDD: 005-0686) are shown. The materials were identical except for the BD-MW group, which showed a calcium hydroxide peak, visible at 18°2θ. No other group showed this peak.

The FT-IR plots of the six groups are shown in Fig. 3 . FT-IR spectroscopy spectrum of BD was analyzed between 4000–400 cm −1 . Broad peaks at the region 3400 (water or hydroxide), 2500 and sharper peaks at 1420 and close to 700 cm −1 (carbonate) could be identified. The band in the 3400 cm −1 region is typical of free OH, which can be present due to water or calcium hydroxide present in the material. This band was modified for all the groups except BD-AF when compared with the control group (BD). At 700 cm −1 , typical of carbonate, there was also changes for the BD-AF group, with less absorbance measured.
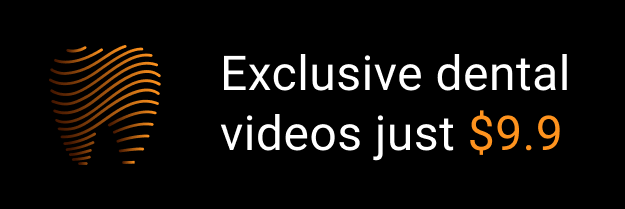