Highlights
- •
Buccal chamfer does not improve retention of reattached incisors for lingual load.
- •
Chamfers strengthen incisor reattachment not by increasing bond area.
- •
Lingual chamfer may play a key role in retention for upper incisor reattachment.
Abstract
Objective
This study aimed to evaluate the effect of different chamfer preparations on the load capacity of reattached fractured incisors under lingual loading.
Methods
Eighty #8 typodonts were randomly assigned to four groups (n = 20 each). They were sectioned to simulate crown fracture, and reattached with a self-etch adhesive and a resin composite. The preparation for each group was: (1) no chamfer; (2) buccal chamfer; (3) lingual chamfer; and (4) circumferential chamfer. Forty-eight human lower incisors were grouped and prepared similarly (n = 12 each). These teeth were tested for their load capacity under a lingual load on a universal testing machine. Finite element models were used to examine the stresses on the reattached surfaces to help interpret the experimental results.
Results
The buccal chamfer did not increase the load capacity when compared with the no-chamfer group. Lingual and circumferential chamfers respectively increased the fracture load by 36.9% and 32.3% in typodonts, and 78.5% and 33.3% in human incisors. The increase was statistically significant (p < 0.05). A higher fracture load tended to be accompanied by a larger area of deflected cohesive fracture. Finite element analysis showed that lingual and circumferential chamfers reduced the fracture-causing tensile stress at the lingual margin of the reattachment interface by approximately 70% and 60%, respectively, in human upper incisors.
Significance
It was the joint design, and not the size of the bond area, that affected the load capacity of reattached incisors. Among the preparations considered, only those with a lingual chamfer could increase the load capacity of reattached incisors under a lingual load.
1
Introduction
Incisor crown fracture is a common trauma to children and adolescents. It mostly happens to the upper incisors. When the fragment can be retrieved and is of a significant size, reattachment is recommended for several reasons: shorter chair time, better esthetics, better wear resistance, etc. From an earlier review [ ] to a contemporary textbook [ ], a buccal chamfer is recommended for concealing the fracture line. It is therefore common to see the use of buccal chamfers after reattachment in case reports [ , ].
As it is weak, dentists would usually instruct their patients to avoid heavy function or even any loading of the reattached tooth [ ]. Therefore, apart from better esthetics, it is also important to consider the chamfer’s effect on the load capacity of the reattached incisor. Early workers did not think that a chamfer would improve retention [ ]. However, later studies showed otherwise [ ]. The increased load capacity was attributed to an increased bond area [ , ]. Henceforth, it is believed that a buccal chamfer would improve both the esthetics and load capacity of a reattached incisor [ , ].
Most of the studies, including the one showing that a buccal chamfer helped retention [ ], applied loading to the buccal surface of the reattached incisor fragment [ , , ]. But, clinically, the upper incisors are mainly loaded from the lingual side [ ]. Since a lingual load would induce fracture-causing tensile stresses on the lingual side only, it is doubtful that a chamfer on the buccal surface, which would be under compression, could increase the load capacity of a reattached incisor.
In general, there can be three types of chamfers: buccal, lingual and circumferential. In this study, the effect of these different chamfer preparations on the load capacity of reattached incisors under a lingual load was investigated. The aim was to provide a better understanding of the mechanics and a better outcome of this clinical procedure.
2
Materials and methods
2.1
Sample preparation
2.1.1
Typodont samples
To avoid the material and dimensional variations of human teeth, typodonts were used in the first part of the experiment. A total of 80 typodonts (#8, Kilgore international, Coldwater, MI, USA) were randomly assigned to 4 groups ( n = 20 each) according to the chamfer design for reattachment. All of the typodonts were sectioned 4 mm from the incisal edge, perpendicularly to the tooth axis. Cutting was done with a slow speed saw (Buehler, Lake Bluff, IL, USA) under water cooling.
Prior to reattachment, the cut surfaces were washed with distilled water spray, dried, treated with a universal adhesive (Scotchbond™ Universal Adhesive, 3 M OCSD, St Paul, MN, USA) for 20 s, and then air-dried. Then the adhesive on the two surfaces was light cured for 15 s. A thin layer of resin composite (Filtek™ Supreme Ultra Universal Restorative, 3 M OCSD) was placed on the cut surface of the main tooth portion, and the fragment was reattached. Excessive materials were removed, and the buccal and lingual fracture lines were light cured for 40 s each.
Group 1 used direct reattachment without further preparation. In Group 2, a 1-mm-deep chamfer was prepared after reattachment with a round bur along the buccal fracture line. Then, the chamfer was treated with the same adhesive for 20 s, air-dried, light-cured, and restored with the same resin composite. In Group 3, a 1-mm-deep chamfer was made and restored on the lingual fracture line using the same steps as those for Group 2. In Group 4, a 1-mm-deep chamfer was made circumferentially along the entire fracture line, and restored using the same steps as those for Groups 2 and 3. The surface of the restored chamfers was polished with a high-speed bur.
2.1.2
Human lower incisors
For their ease of collection, human lower incisors were used as a substitute for upper incisors in the second part of the experiment. The teeth had been extracted for periodontal reasons, and informed consent was obtained for experimentation. The teeth were cleaned and stored in thymol solution until use. A total of 48 intact human lower incisors of similar size were selected. These incisors were randomly assigned into 4 groups (n = 12 each). The incisal one-thirds of their crowns were sectioned off, perpendicular to the tooth axis, and then reattached as for the typodonts. However, before applying the adhesive, the cut enamel was acid-etched for 15 s with Scotchbond™ Universal Etchant (3 M OCSD), rinsed with distilled water for 15 s, and air-dried. Grouping and chamfer preparation were the same as those for the typodonts. Restoration of the chamfers also followed the same procedure, except that the prepared surface in these real teeth was acid-etched for 15 s, rinsed for 15 s, and air-dried before applying the adhesive.
The typodont and human incisor samples were then tested for their load capacity under a lingual load.
2.2
Fracture test
All specimens were individually mounted and subjected to mechanical loading in a universal testing machine (MTS 858 Min. Bionix II, MN, USA). The root was fixed on a clamping device placed on the load cell, and a steel head was used to load the reattached fragment on the lingual surface at a point 1.5 mm away from the incisal edge until fracture. The loading was perpendicular to the long axis and the mesiodistal direction of the incisor. The speed of loading was 0.5 mm/min. The load capacity of the specimens was recorded and compared statistically using one-way ANOVA on SPSS 21 (IBM, Armonk, NY, USA). Post-hoc tests performed were Dunnett T3 with heterogeneous variance, and LSD (Least Significance Difference) with homogeneous variance. The significance level was set to α = 0.05.
The fracture loads (L f ) in each group were also analyzed using the Weibull statistical model [ ]. The probability of survival (P s ) was calculated using the estimator [ i /( n +1)], where i is the rank of a specimen based on its L f , and n is the sample size [ ]. The Weibull modulus, a measure of reliability, for each group was determined from the slope of the graph of lnln(1/P s ) against ln(L f ).
2.3
Fracture mode analysis
The fracture surface of both the typodonts and human incisors was inspected visually. Their fracture modes were classified according to the area of cohesive failure.
2.4
Finite element analysis (FEA)
A human upper central incisor was scanned using a micro-computed tomography machine (HMX-XT 225, Nikon, Brighton, MI, USA) with the following parameters: accelerating voltage of 100 kV, beam current of 70 μA, 720 projections and 4 frames per projection, with 708-ms exposure time per frame. CT 3D Pro 3.1.11 (Nikon Metrology, Inc., Brighton, MI, USA) was used to reconstruct the images in 3D, and VGStudio MAX 3.1 (Volume Graphics GmbH, Heidelberg, Germany) was used to process the images and export them as DICOM files.
The images were then processed with Mimics (Materialise NV, Leuven, Belgium) to separate the different regions. 3-Matic Research (Materialise NV, Leuven, Belgium) was used to virtually cut the incisal 1/3 of the tooth model to simulate the crown sectioning. Buccal, lingual and circumferential chamfers were introduced to form models for the different groups. The majority of the root was removed for simplicity. Element sizes of 0.4, 0.3 and 0.2 mm were used for the tooth model to check for convergence. After assembling the different parts and meshing, the resultant models were imported into Abaqus (SIMULIA, Providence, Rhode Island, USA) to set up material properties, boundary conditions and loading conditions. A steel sphere of 4-mm diameter was constructed to load the lingual surface of the reattached fragment with prescribed displacement. As in the experiments, the movement of the sphere was perpendicular to the long axis and the mesiodistal direction of the tooth.
All materials were assumed to be isotropic, homogeneous and linearly elastic. The material properties used are listed in Table 1 . The different parts of the tooth were perfectly bonded to each other, except the fracture surfaces and resin-tooth interfaces, for which surface-to-surface interaction was defined for outputting interfacial stresses. As an example, the no-chamfer model had a uniform mesh of 151,630 tetrahedral elements (0.3 mm) and 33,518 nodes ( Fig. 1 ). The cervical end of the model was fixed in all directions, and the displacement of the steel ball was adjusted such that the reaction force at the cervical base (hence, the load) had the same value in all the groups. The contact pressure on the interface of reattachment, which was equal and opposite to the interfacial normal stress, was used to evaluate and compare the tendency to failure among the groups.
Young’s modulus (GPa) | Poisson’s ratio | |
---|---|---|
Enamel | 40 | 0.3 |
Dentin | 18.6 | 0.3 |
Composite | 12 | 0.3 |
Steel | 200 | 0.3 |

3
Results
3.1
Fracture loads
The fracture loads of the specimens are shown as box plots in Fig. 2 . The typodonts had similar fracture loads as the human incisors. The buccal-chamfer group did not have a higher load capacity than the no-chamfer group for both the typodonts and human incisors. In fact, it significantly lowered the load capacity for the typodonts (p < 0.05). Lingual and circumferential chamfers, on the other hand, increased the load capacity (p < 0.05). While the increase was similar between these two chamfer designs for the typodonts, the increase by the circumferential chamfer was not as great as that by the lingual chamfer in the human incisors.
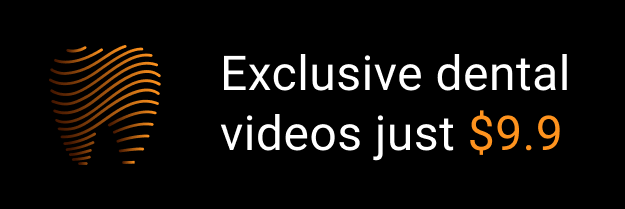