Parameter measured
Result
Reference
SEM characterization
Fluoride treatment reduces roughness at the micrometer scale but produces nanostructures
Roughness (average surface roughness/Sa, μm)
1.32–1.82
XPS (F atomic %)
0.3–1.0
Contact angle (°)
138
[136]
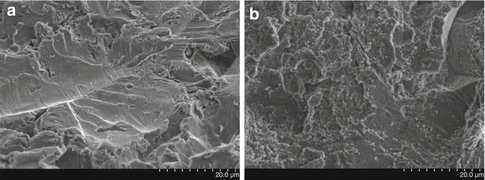
Fig. 6.1
SEM images of blasted titanium implant surfaces before (a) and after (b) fluoride treatment, obtained at 10 kV and ×2,000 magnification
Chemical analyses of these surfaces by the use of X-ray photoelectron spectroscopy (XPS) spectra has revealed binding energies corresponding to oxygen, titanium, carbon, fluoride, and traces of nitrogen [42]. Fluoride was in this study present at a level of 1.0 %, a similar level also reported by Petersson et al. [44], but lower fluoride values of the OsseoSpeedTM surface, 0.4 %, are reported in the literature also using XPS analysis [45]. Exact analysis of fluoride on this level is technique sensitive, and these differences may be due to variations between the analytical protocols. By treating titanium with fluoride, the fluoride ions will exchange with hydroxyl groups at the surface. The fluoride content that remain on the TiO2 surface of dental implants after a rinsing process are calculated to occupy approximately 5 % of all active sites equaling that every 20th site is occupied by an exchanged fluoride ion. This change in the surface TiO2 composition increases the conductivity and lowers the surface charge of the implant that may have biological consequences [44]. It is further hypothesized that the fluoride ion as such may contribute directly to the calcification process through ion exchange and binding to phosphate molecules in the bone structure [46, 47]. The nanoscale roughness created by the fluoride modification may add further bone-promoting effect to the already seen by the microstructure due to the blasting. A unique nucleating effect is demonstrated by fluoride-modified Ti in that when the implant is immersed into a liquid saturated with respect to calcium and phosphate, it attracts these ions to the surface and crystals of calcium phosphate start to grow [48]. Based on physicochemical evaluation, this designed implant surface had potential for improving bone integration and regeneration.
Molecular and Cellular In Vitro Response to Fluoride-Modified Titanium Implants
The formation of mineralized bone at the bone-to-implant interface requires the recruitment of osteoprogenitor cells, its proliferation, and differentiation to mature osteoblasts followed by the production and mineralization of extracellular matrix at the surface [49]. Osteoblast cell culture in vitro models provide a useful tool to study the cellular and molecular events of osteoblasts in response to different implant surfaces [50].
Osteoblast maturation in vitro has been divided into three major developmental stages, a period of growth and proliferation, a period of extracellular matrix development and maturation, and a mineralization stage (Fig. 6.2), with characteristic changes in gene expression at each stage [51–54]. The analysis of cell attachment, proliferation, gene expression, and mineralization in cells cultured onto different implant surfaces are valuable data to define the effect of the different surfaces at the implant interface.
The main sources of osteoprogenitor cells are MSC, multipotent cells that can differentiate along a variety of cell lineages [55]. During the commitment towards a specific lineage, a master gene, such as a transcription factor, is induced and starts a cascade that leads to the sequential expression of other transcription factors and of phenotype-specific genes [56]. Runt-related transcription factor 2 (RUNX2 or formerly called Cbfa1), a member of the runt homology domain transcription factor family, is the master gene necessary for the osteoblast lineage commitment [57], also influences on the signaling pathways and transcriptional factors which regulate osteoblastogenesis [58], and modulates the expression of bone extracellular matrix protein genes [59]. Downstream of RUNX2 is Osterix (OSX), a specific osteogenic zinc finger transcription factor that is required for the ongoing differentiation within the osteogenic pathway [60], and is involved in the differentiation step from preosteoblast to fully functional osteoblast [61].
During osteoblast differentiation, type I collagen (COLL-I) is expressed in high levels in the early synthetic stage, supporting cell proliferation [54], and its expression is gradually decreased as the cell matures. Alkaline phosphatase (ALP), a membrane-bound enzyme contained in matrix vesicles that contributes to rendering the extracellular matrix competent for mineralization [54], is considered an early marker of osteoblast differentiation; hence, its expression increases during extracellular matrix maturation and decreases when mineralization is well progressed [62]. Bone sialoprotein (BSP) is transiently expressed very early and then upregulated again in differentiated osteoblasts at the onset of mineralization. Osteopontin (OPN) is a sialoprotein that mediates hydroxyapatite binding, being produced early in the differentiation of bone cells with higher expression levels after mineralization has been initiated [63, 64]. Finally, osteocalcin (OC) appears with mineralization, being only expressed by fully differentiated osteoblasts [51, 54].
The cellular response to fluoride-modified Ti implants has been assessed in different osteoblast cellular models (Table 6.2) using MSCs from different origin [65–69], primary cultures of osteoblasts [70–73], nontransformed clonal cell lines (MC3T3-E1) [43, 74, 75], or osteosarcoma cell lines (MG63) [76]. The different cellular models, time-point of the analysis, or implant production might explain the differences in the reported results. Thus, while some studies have reported increased proliferation on fluoride-modified titanium implants [43, 65, 66], others failed [67, 75]. In solution, fluoride has been proved to stimulate bone cell proliferation [77], but its effect varies according to the stage of differentiation of the cells; thus, the fluoride ion acts primarily on osteoprogenitor cells or undifferentiated osteoblasts rather on more differentiated osteoblasts [78]. In addition, some studies find higher cell adhesion [71] in fluoride-modified titanium implants compared to control; other studies found no differences [65, 66, 68, 73]. In this regard, it is important to include the importance of the surface topography when discussing the number of cells attached on the surfaces since the modification of titanium surface with HF is influenced by HF concentration, the exposure time, and the initial surface topography [43]. In the same line, differences in the results of gene expression analysis (Table 6.2) might also be explained by differences in the roughness or chemical composition of the surfaces used in the different studies. However, fluoride-modified Ti implants (Fig. 6.2) stimulate osteoblast differentiation and expression of bone-specific mRNA [43, 74], as does fluoride in monolayer bone cell cultures [78]. Thus, fluoride-modified Ti implants increase the expression of RUNX2 [67–69, 74], OSX [68, 74, 75], COLL-I [66], and BSP [65, 75] and increases ALP activity [73]. In addition, fluoride modification augments the thrombogenic properties of titanium, promoting fibrinogen activation and rapid coagulation, resulting in a less dense fibrin clot that could promote osteoblast migration to the implant surface in vivo [79].
Table 6.2
Summary of in vitro studies investigating the cellular response to fluoride-modified titanium implants
Reference
|
Cell type
|
Cell culture time
|
Type of surface
|
Type of analysis
|
Cell response
|
---|---|---|---|---|---|
[76]
|
Human osteoblast-like cells (MG-63)
|
<45 min
|
TiOblast/OsseoSpeed/machined Ti
|
Cytodetachment
|
Higher cytodetachment in OsseoSpeed than in TiOblast, but not statistically significant. Machined Ti significantly lower cytodetachment than rough surfaces
|
[65]
|
hMSC
|
1 h–28 days
|
GB/GB-HF
|
Cell adhesion, proliferation, and gene expression analysis
|
No differences in cell adhesion
|
Greater proliferation and increased BSP and BMP-2 expression in GB-HF than GB
|
|||||
[70]
|
Primary human mandibular bone cells
|
12 h–10 days
|
Fluoride etched/Alkali-head treated/magnesium ion incorporated anodized/nano-HA coated and heat treated/GB
|
CaP formation in SBF
|
Earlier CaP formation in all modified surfaces than GB, but after 72 h, GB showed higher CaP formation than the modified surfaces
|
Cell attachment, OC, and protein production
|
The fluoride-etched surface treated for 72 h with SB before cell culture showed similar or lower bone cell response than GB
|
||||
[66]
|
Human bone marrow MSCs
|
3 h–14 days
|
GB/GB-HF
|
Cell adhesion, proliferation, and protein production and activity
|
No differences in cell adhesion
|
Higher proliferation and increased COLL-I and OPG expression levels on GB-HF than GB
|
|||||
[74]
|
Mouse pre-osteoblasts (MC3T3-E1)
|
1–14 days
|
GB/GB-HF
|
Gene expression analysis
|
Higher levels of Runx-2 and Osterix in GB-HF than GB
|
[67]
|
Human embryonic palatal MSC
|
1, 3, 7 days
|
GB/GB-HF
|
Cell proliferation, ALP activity, gene expression analysis
|
No differences in proliferation
|
Higher expression levels of Runx-2 in GB-HF
|
|||||
[43]
|
Mouse pre-osteoblasts (MC3T3-E1)
|
1–7 days
|
P/P-HF (treated for 40, 90, 120, and 150 s)
|
Cytotoxicity, cell number and gene expression analysis
|
Lower cytotoxicity in P-HF, being significant in Ti treated with HF for longer times (120 and 150 s)
|
Higher cell number after 7 days in P-HF treated for 120 and 150 s
|
|||||
No significant differences on gene expression
|
|||||
[71]
|
Primary mouse alveolar bone cells
|
48 h
|
Nanotite/OsseoSpeed/TiUnite/SLActive
|
Cell adhesion analysis
|
Higher cell adhesion rate in OsseoSpeed and SLActive than the other surfaces (being as well the two surfaces with higher roughness)
|
[68]
|
Human mesenchymal pre-osteoblasts (HEPM)
|
72 h
|
TiOblast/OsseoSpeed/SLA-1/SLA-2
|
Cell attachment and gene expression analysis
|
No differences in cell attachment
|
Increased Runx-2 and Osterix expression levels in TiOblast and OsseoSpeed
|
|||||
[75]
|
Mouse pre-osteoblasts (MC3T3-E1)
|
2–14 days
|
TiOblast/OsseoSpeed
|
Cytotoxicity, cell morphology and proliferation, ALP activity, gene expression, and release of osteoblast markers
|
No differences on cell viability and cell proliferation
|
Increased secretion of BMP-2 secretion after 2 days of culture in OsseoSpeed
|
|||||
Increased IGF-I, BSP, and Osterix gene expression levels in OsseoSpeed after 14 days
|
|||||
Increased mineralization in OsseoSpeed after 14 days
|
|||||
[72]
|
Human osteoblasts
|
1 day
|
P/P-HF/GB/GB-HF
|
Gene expression analysis by microarray and RT-PCR
|
Identification of novel genes involved in the early response of osteoblasts to rough and to fluoride-modified titanium implants
|
[73]
|
Primary mouse osteoblastic cells
|
21 days
|
sulfuric-hydrochloric acid/anodic oxidation with phosphoric acid/chemical attack plus thermal oxidation immersion NaF
|
Cell attachment, proliferation, and differentiation
|
No differences on cell attachment
|
Higher ALP activity in the sulfuric-hydrochloric surface and in the chemical attack plus thermal oxidation immersion NaF
|
|||||
[79]
|
No cells: in vitro slide chamber model furnished with heparin in contact with blood, PRP, or PPP
|
Hydroxyapatite surface/machined Ti/TiOblast/OsseoSpeed
|
Generation of thrombin-antithrombin complexes
|
Higher thrombin-antithrombin complexes formation in OsseoSpeed compared to the other surfaces
|
|
[69]
|
Human MSCs
|
1–28 days
|
GB/GB-HF
|
Gene expression analysis
|
Osteoblast differentiation more rapid and to a greater extent in GB-HF
Higher expression of Runx-2, Smads, insulin-like growth factor 2, BMPs, and bone matrix proteins
|
Bone Healing Around Fluoride-Modified Titanium Implants in In Vivo Models
In general, Ti implants are classified as bioinert [80], characterized by contact osteogenesis, in which the osteogenic cells migrate directly to the surface where they will establish de novo bone formation [81]. In the very first animal study performed with fluoride-modified Ti, it was observed that non-threaded conically shaped Ti implants with a machined surface increased the retention three to four times as measured by a push-out test when the surface had been modified by fluoride; the push-out values were depending on the fluoride solution used [46]. It was further reported from this study that the test implants had bone firmly attached to the surface after the push-out as observed by SEM. This first observation clearly indicated that the bone had reacted differently to the fluoride-modified implants compared to the control Ti implants with a natural TiO2 layer. The observation of an attachment between the implant and bone with increased implant retention was further evaluated by Ellingsen and co-workers who placed 80 threaded grit-blasted implants with or without fluoride-modified surface into the rabbit tibiae [82]. The functional attachment of the threaded implants to bone was evaluated by a removal torque test after 1 and 3 months healing time. In this study, there were no significant differences in removal torque values after 1 month healing time, but significant higher values were recorded in the fluoride-modified group compared to the blasted group, 85 Ncm versus 54 Ncm, after 3 months healing. In the histomorphometric analysis, significantly higher BIC for the fluoride-modified implants was observed already after 1 month healing time, and the difference between the groups was even more pronounced after 3 months healing. The lack of significance between the groups when evaluating functional attachment after 1 month healing time could be due to that the bone that was formed, with significant higher BIC, was not yet fully mature and calcified and thus not able to resist the removal torque forces. Later, Cooper and co-workers placed grit-blasted Ti implants into rat tibiae and compared these to implants with similar surface treatment, but with an additional fluoride modification. At day 21 after surgery, the rats were euthanized and the implants were prepared for histology and histomorphometric analysis [65]. In this study, the grit-blasted implant group had a mean direct BIC of 34.2 %, whereas the fluoride-modified group had a significantly higher direct BIC of 55.5 %. In the same study, significantly increased expression of genes related to bone regeneration was recorded in the fluoride-modified group as discussed in the previous section. Then, the early stage of osseointegration was investigated by placing grit-blasted implants with or without fluoride modification in the alveolar process of mongrel dogs [83]. The bone regeneration and growth in a chamber created between the cut surface of the old bone was studied, and the authors reported that the amount of new bone that formed in the chambers within the first 2 weeks of healing was larger at sites where the internal walls of the wound chamber were designed with a fluoride-modified surface (test) than with a control surface (TiOblast®). The BIC that had been established in the macro-threaded part of the implant was significantly larger at the fluoride-modified implants than the grit-blasted-only control implants. In another interesting study from the same group, the focus was on the healing of marginal bone when implants were placed without direct contact to the bone, imitating the clinical situation of implant placement into extraction sockets [84]. The marginal 50 % of the prepared canal for the implant was widened to give a free space of 1 mm around the implant, and the bone regeneration in this gap was evaluated. Abrahamsson and co-workers found that the quality of the contact between the implant and the newly formed bone differed between test and control implants. The % of BIC within the defect area was in the 2-week specimens 55.7 % at test sites and 33.7 % at control sites. After 6 weeks of healing, the % of BIC had increased to 63.7 and 45.2 % at test and control sites, respectively. The histological analysis in this study thus revealed a significantly larger area of osseointegration within the defect at fluoride-modified (OsseoSpeedTM) implants than at implants with a grit-blasted-only (TiOblastTM) surface after 6 weeks of healing. Another interesting fact of the fluoride-modified Ti implants was found by Thor and co-workers who demonstrated that Ti in whole blood activates the thrombogenic response to a higher degree than platelet-rich plasma (PRP) and that a fluoridated Ti surface augmented the effect compared with the control. This property was further evaluated by the same group in an implant defect model in dogs [79, 85]. The defects of 1.25 mm were made around the implant and the regeneration of bone was evaluated regarding the influence of PRP, whole blood, grit-basted surface and grit-blasted surface, and fluoride modification. The authors confirmed the findings from the previous in vitro study that PRP does not improve bone regeneration in peri-implant tissue compared to whole blood and a statistically significant higher bone fill was recorded in the defects with OsseoSpeedTM implants compared to TiOblastTM implants. The most superior point of the bone envelope in marginal bone was attached at a shorter distance from a perpendicular point on the OsseoSpeedTM implants compared with the control, irrespective of defect-fill PRP or whole blood.
An even more detailed information about the regeneration process around the OsseoSpeedTM implants after their installation in vivo in rabbits was given by Monjo and co-workers who analyzed bone-implant retention in combination with gene expression of several inflammatory and osteogenic markers of the peri-implant bone after the tensile test was completed. In addition, enzymatic analyses of the wound fluid and micro-computed tomography (micro-CT) analysis of the intact bone were performed [86]. Implant placement elicits a sequence of healing events leading to osseointegration including bleeding, inflammation, and subsequent resorption of traumatized bone around the titanium implant concomitant with new bone formation and mineralization (Fig. 6.3). The present investigation demonstrated that in this sequence of events, necrosis and gene expression of inflammatory and resorption markers were reduced at the surface of fluoride-modified Ti implants after an early healing period of 4 weeks. This was followed after a longer healing period of 8 weeks by a significant increased bone-to-implant retention, gene expression of bone formation markers, and bone mineral density, reflecting an improved bone remodeling activity.
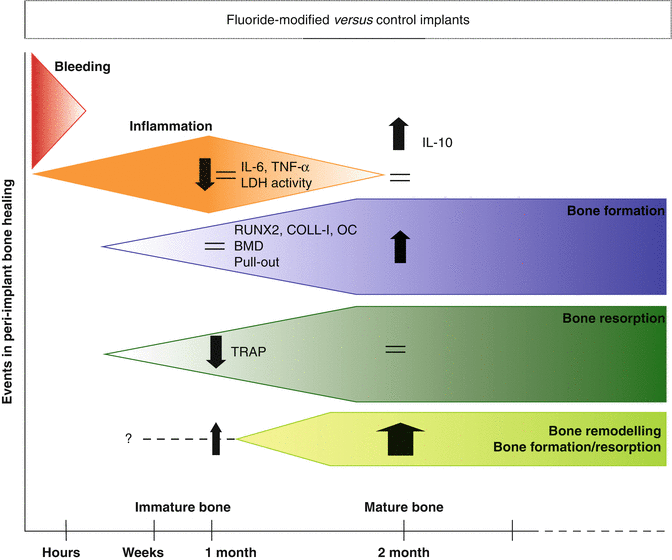
Fig. 6.3
Events in the peri-implant bone healing in fluoride versus control modified implants. Schematic representation of the events in the peri-implant bone healing and the reported effects of the fluoride-modified implants analyzed by enzymatic analyses, gene expression, biomechanical test, and micro-computed tomography (Results extracted from Monjo et al. [86])
In conclusion, all these investigations using different animal models (Table 6.3) represented and demonstrated clearly that this new surface gave an improved bone response after implantation with a firmer attachment and higher degree of contact between bone and implant. Although most of the studies focused on histology [42, 46, 65, 82–85, 87–95] and biomechanical tests [42, 46, 82, 86
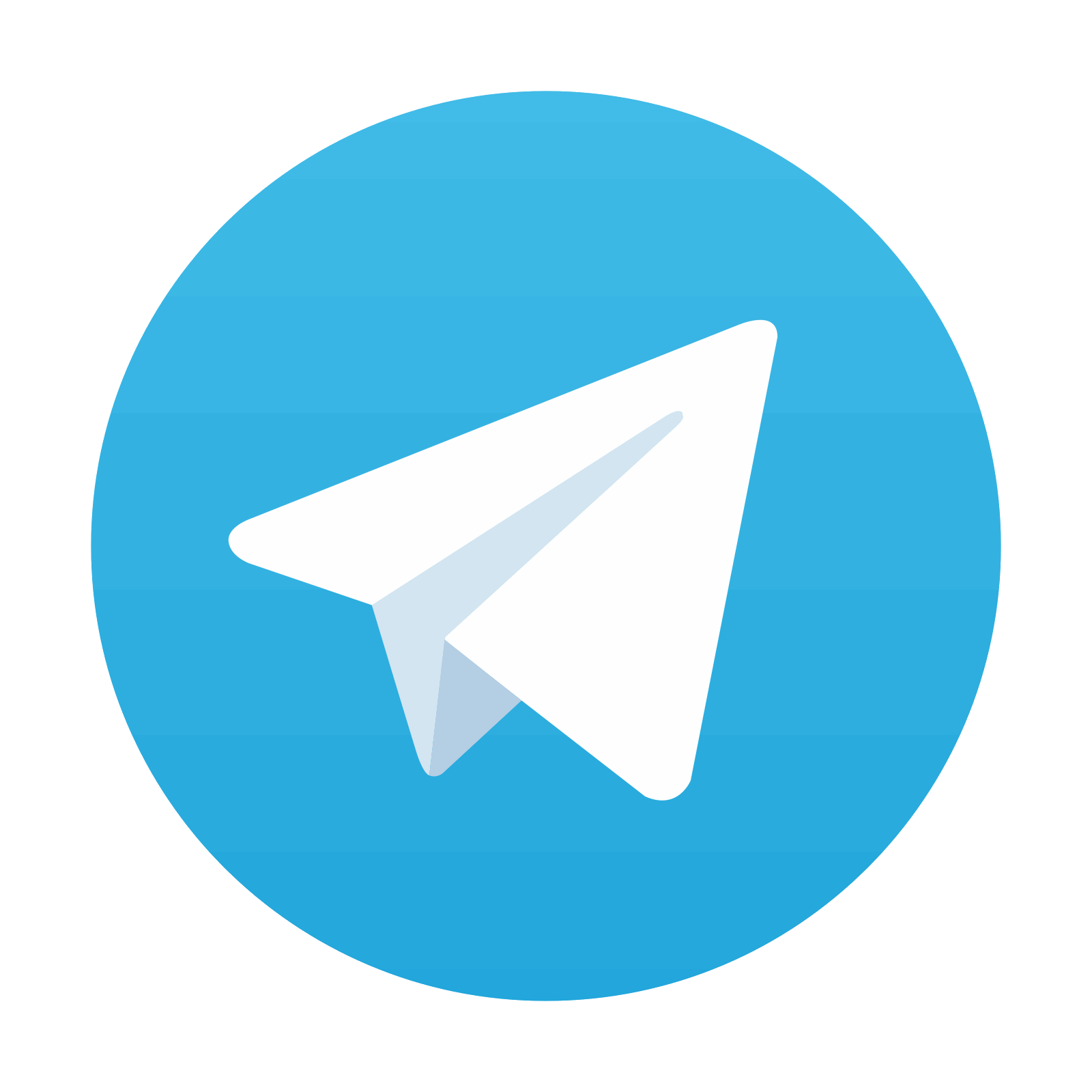
Stay updated, free dental videos. Join our Telegram channel

VIDEdental - Online dental courses
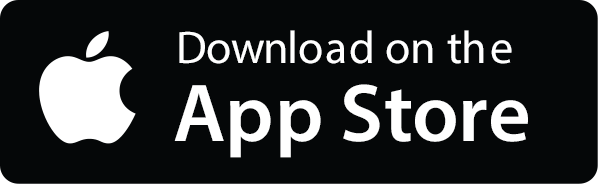
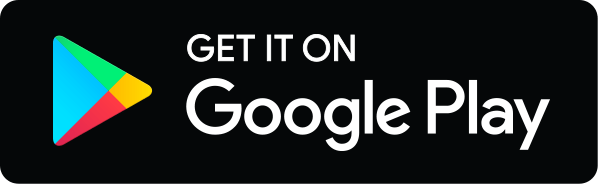