Fig. 7.1
Crystalline structure of alumina
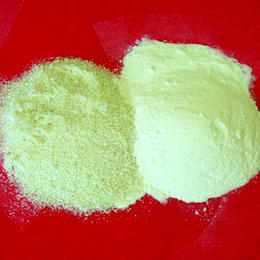
Fig. 7.2
Typical alumina powder: raw material for alumina ceramic bodies
7.1 Mechanical Behavior and Microstructure of Alumina Dental Ceramics
The mechanical behavior of alumina is strongly affected by its microstructure because of the propagation of cracks and secondary microcracks. The improvement of mechanical properties of alumina for use in unfavorable service situations is one of the major challenges facing researchers in recent decades.
Piconi and Maccauro (1999) state that alumina used as a biomaterial must have high purity (99.9 %), low porosity (<0.1 %), high flexural (>500 MPa) and compression strength (>4,100 MPa), a K IC superior to 4 MPa.m1/2 and hardness greater than 2,200 HV0,1 (Figs. 7.3 and 7.4).
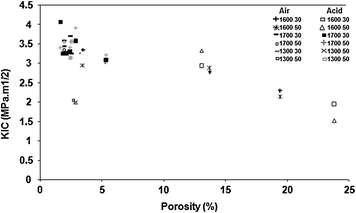
Fig. 7.3
Relationship between porosity and K IC in alumina ceramic bodies exposed or not to acetic acid
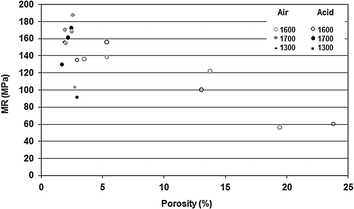
Fig. 7.4
Relationship between porosity and mechanical resistance in alumina ceramic bodies exposed or not to acetic acid
The U.S. National Institute of Standards and Technology predicts that at a temperature of 20 °C the properties of high purity (99.5 %) Al2O3-α with a grain size of 5 μm and a density of more than 98 % should be the following: melting point of 2,050 °C, compressive strength of 3 GPa, modulus of elasticity of 450 GPa, flexural strength of 380 MPa, fracture toughness (with crack of 300 μm in length) of 3.5 MPa.m1/2, Vickers hardness of 15 GPa, Weibull modulus of 11 and tensile strength of 267 MPa. According to Kelly (2004), the K IC of alumina prostheses is around 4.5 MPa.m1/2, while for conventional metal-ceramic prostheses this indicator is around 1 MPa.m1/2 and for zirconia prostheses between 8 and 12 MPa.m1/2.
Some additives may be added during the production of alumina, such as magnesium oxide (MgO), zirconium oxide (ZrO2) and chromium oxide (Cr2O3) in order to improve certain characteristics and mechanical properties. Furthermore, the microstructure of polycrystalline alumina as well as some of its properties depends on the additives and the residual presence of silicon and calcium oxides. These oxides are quite common and their control is critical. Many of them have low solubility in alumina and concentrate in the grain boundary. The segregation rate of these oxides varies with the orientation of the crystal planes of the alumina. At high concentrations, these oxides may favor the formation of the glassy phase.
The introduction of sintering additives during the processing of alumina can control grain growth and thus influence the crack growth process and consequently improve the mechanical properties of ceramic materials.
The presence of second phase or elongated grains can increase the fracture toughness of alumina, allowing the deflection of cracks. Barinov et al. (1998) state that the presence of glassy phase in alumina increases its sensitivity to crack growth, leaving the alumina less resistant to fatigue.
Teng et al. (2007) report that one of the problems with using alumina is its low flexural strength. These same authors state, however, that the mechanical properties can be improved with finer grains and by strengthening the grain boundary.
Alumina has a tendency of presenting abnormal grain growth and often the addition of dopants can avoid this. The addition of MgO and/or ZrO2 can increase flexural strength of alumina between 17–27 % in three-point bending tests. The addition of these components increases the density of alumina and decreases its grain size. These researchers obtained alumina with a 40 % smaller grain size with the addition of MgO, 50 % lower with the addition of ZrO2 and 80 % lower with the addition of MgO and ZrO2.
Grain boundary has a strong influence on important phenomena of the sintering process such as densification and grain growth, deformation, segregation mechanical properties and optical properties. The influence of grain boundary on the ceramic properties depends on factors such as its density and the chemical composition of its interface.
Differences in the thermal expansion coefficient between the crystalline and glassy phase may create stresses at the grain boundaries during cooling of the ceramics. These stresses can affect the mechanical properties of alumina for dental applications. The compressive forces arising from the glassy phase formed around the grains can promote crack growth. The effect of this phenomenon on fracture toughness, however, is still not well understood. Still, the authors have found that alumina with small grains is more resistant to fracture, which restates the importance of microstructure for the properties of ceramics.
Guazzato et al. (2004) state that the microstructure, strengthening mechanisms and the inter-relationships between the ceramics an these strengthening elements, as well as the mechanical properties, should be more thoroughly researched. Quinn et al. (2003) state that although microstructure is important in determining the properties of ceramics, the chemical composition should also be considered.
One of the characteristics of alumina is the R curve. The R curve represents an increase of fracture energy with the extension of the crack, indicating lower sensitivity in relation to the size of the defect in the microstructure and greater reliability of the material. This behavior can be explained by the energy consumed when crack branching, plastic deformation, and encapsulation of the crack by the damaged area during crack growth, occurs. The effect of the R curve is well observed at the beginning of the macrocrack growth and tends to saturate. However, some studies have not shown any reductions in the variability of resistance with the R curve.
Indentation tests shows that alumina containing elongated and crude grains, has an upward R curve, indicating stable crack propagation, less variability in mechanical strength. The greatest resistance is presented by alumina with a microstructure of fine and equiaxial grains and a small or even absent R curve. However, that R curve behavior and tolerance to defects are not exclusively controlled by average grain size, but by grain morphology and other factors such as grain distribution and also the mechanical strength of its boundaries.
Hotta et al. (2005) report that not only the presence, but also the size and distribution of coarse grains can be correlated with the differences in mechanical resistance between aluminas. Alumina with elongated grains can present higher fracture toughness in indentation tests of up to 80 %.
Armstrong and Cazacu (2006) have assessed the fracture toughness of alumina in indentation tests with respect to grain size and crack size. They concluded that the intensity of fracture toughness (K) in the presence of small defects (even if these are larger than the grain size) increases with decreasing grain size. In large defects, K increases with increasing grain size.
Muchtar and Lim (1998) have studied the fracture toughness in indentation tests of high purity (99.99 %), fine-grained alumina and found that the intensity of fracture toughness (K IC ) increases with decreasing grain size. The same authors assessed fracture type and concluded that in fine-grained alumina, fracture occurred intergranularly, while in alumina with dispersed grains, the fracture was a combination of intergranular and transgranular fracture. Authors such as Armstrong (2001) and Njiwa et al. (2005) also report that the mechanical resistance of alumina increases with decreasing grain size.
In recent years, different microstructure concepts have been developed to overcome the inherent brittleness of ceramics. Present studies focus on the possibility of reinforcing alumina with carbon nanotubes, improving its mechanical properties, especially fracture toughness.
7.2 Optical Properties of Alumina Dental Ceramics
Patients throughout the world are showing a growing interest in restorative dental materials, which enable natural teeth to be faithfully recreated with regard to their function and aesthetic appearance.
Tooth color is caused by volume reflection: the passage of incident light through the translucent enamel and dentine followed by backward emergence. This passage is concurrent with the sideward displacement of photons that, in effect, influences the result of spectrophotometric measurement of tooth color. This phenomenon would be applied similarly to shade guide tabs which were made to mimic the color and structure of natural tooth.
Optical properties of human teeth are influenced by their external configuration. Tooth dimension, shape and surface structure generate light reflection patterns, which influence the overall color. Knowing that the amount of reflected and absorbed light depends on the thickness and translucency of dentine and enamel, it is evident that the thickness of the dentine and enamel affect tooth color.
Color assessment is regarded as a complex psychophysiologic process subject to numerous variables. Dentin is considered to be the primary source of color for teeth, which is modified by the thickness and translucency of overlying enamel. The perceived color of natural teeth is a result of light reflected from the enamel surface, in addition to the effect of light scattering within enamel and dentin before it is ultimately reflected back. Clinically, it is important that ceramic restorations reproduce the translucency and color of the natural teeth.
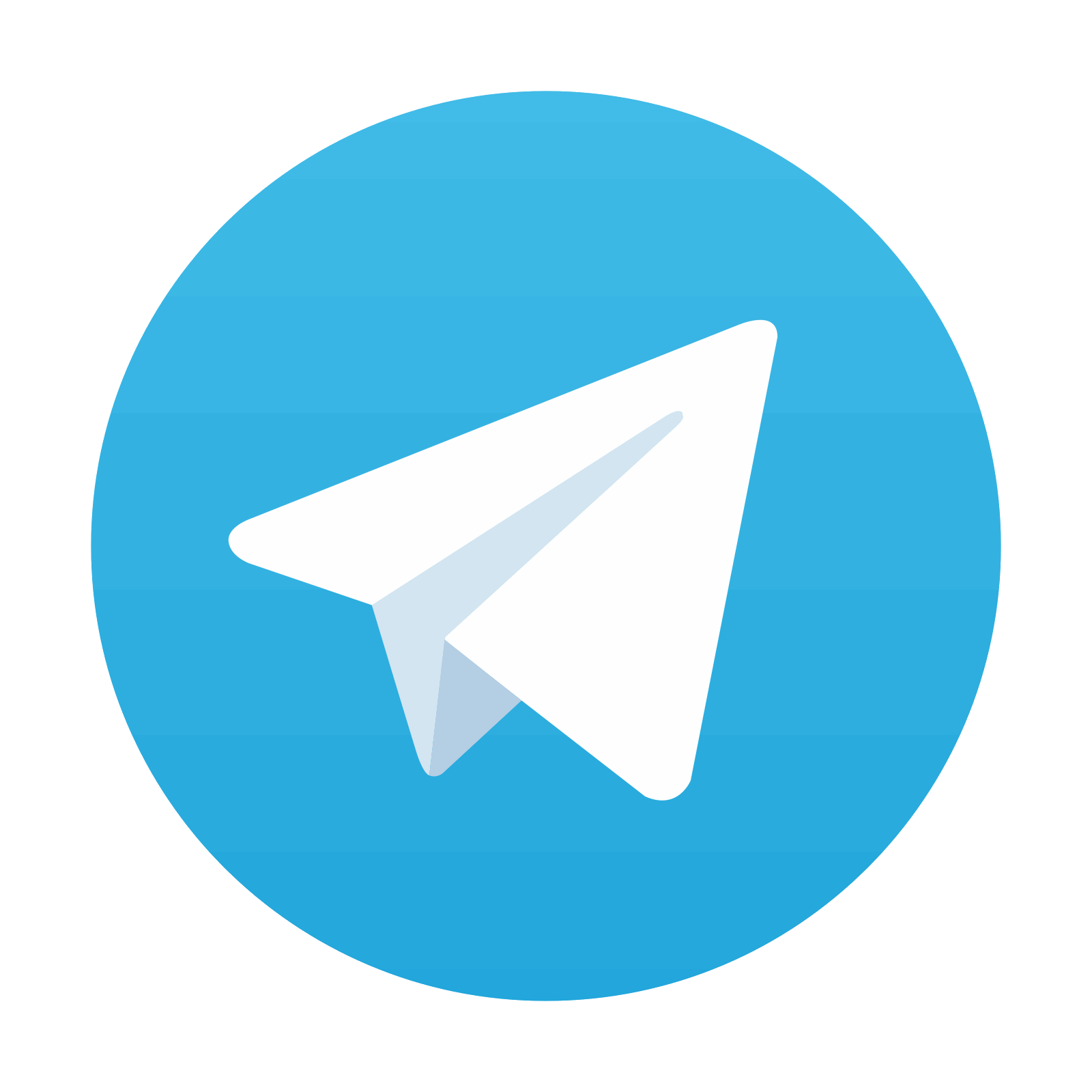
Stay updated, free dental videos. Join our Telegram channel

VIDEdental - Online dental courses
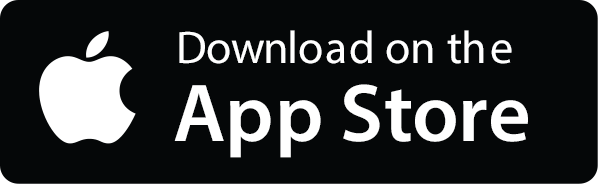
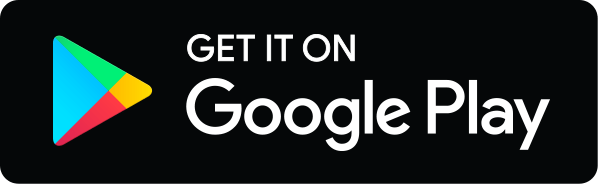