Fig 1.1
Photomicrographs showing osseointegration around stable MSIs (a–c) and fibrous tissues around mobile MSIs (d–f) MSIs (Adapted from Woods et al. [12])
1.2 Primary and Secondary Stability
Long-term MSI stability depends on both primary and secondary stability, two closely related phenomena. Immediately after the MSI has been inserted, its retention is entirely mechanical, due primarily to the characteristics and amount of the bone contacting the MSI, particularly the cortical bone [13, 14]. This mechanical type of MSI retention is called primary stability. For orthodontists, primary stability is important because it allows MSIs to be immediately loaded. More importantly, primary stability is critical for the development of secondary stability. The endosseous literature has shown that primary stability is essential for the long-term success of implants [15]. MSI placement generates stresses and strains along the length of the screw that damage the surrounding bone. Too much damage can lead to micromotion of the implant and early loss due to lack of osseointegration. If MSIs are mobile after 5–6 weeks, they are probably surrounded by fibrous tissues and exhibit no bone-to-implant contact (Fig. 1.1) [12].
Both insertion torque and pullout force need to be measured when evaluating primary stability because their effects should not be expected to be equal. Increasing the shear forces along the screw increases the strain and microfractures, which might be expected to affect the healing process and lead to degeneration or necrosis at the interface. Bone damaged during MSI insertion requires repair, too much of which can result in screw loosening. Theoretically, primary stability can be optimized by minimizing insertion torque and maximizing pullout forces.
During the first few weeks after implant placement, primary stability decreases as osteoclasts remove older, damaged bone, and osteoblasts form new bone. As new bone is formed around the MSI, its stability increases. This second phase of increasing stability is referred to as secondary stability. Secondary stability is due to the osseointegration that occurs around MSIs. As healing and greater amounts of osseointegration occur, secondary stability increases.
Primary and secondary stability each exhibit characteristic curves (Fig. 1.2). The stability that the orthodontist observes clinically is the net or overall stability, composed of both primary and secondary stability. Initially, overall stability is due entirely to primary stability (i.e., there is no secondary stability). Overall stability decreases rapidly at first and then slows down as secondary stability takes over. The point at which the primary and secondary stability curves cross is when MSIs are least stable; it can be identified by the dip in the overall stability curve. At that time, primary stability is increasing rapidly. The rate at which secondary stability increases begins to slow down after 4–5 weeks of healing. After healing has occurred and the bone has remodeled, overall MSI stability is primarily due to secondary stability.
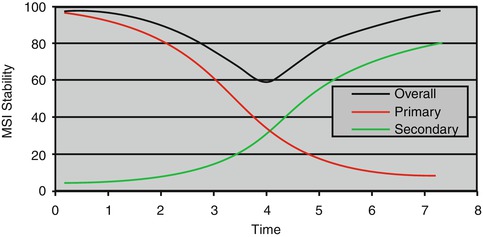
Fig. 1.2
Overall, primary and secondary stability curves of miniscrew implants (MSIs), showing the stability dip 4 weeks after insertion
Theoretically, the overall stability of MSIs could be enhanced by either (1) delaying the decrease of primary stability that occurs after insertion or (2) accelerating the increases in secondary stability that occur with healing (Fig. 1.3). Unfortunately, increasing primary stability often damages the surrounding bone. Finite element analyses indicate that the insertion of MSIs produces bone strains well above physiologic limits [16], which damages the bone, increases remodeling requirements, and could have negative impacts on both primary and secondary stability. Ideally, MSIs should produce the least bone strain, heat, and damage (i.e., minimum insertion torque) while providing the greatest possible primary stability (maximum pullout force) immediately after insertion. Such conditions would establish a local environment that limits the damage of the bone around the MSIs and enhances the healing process.
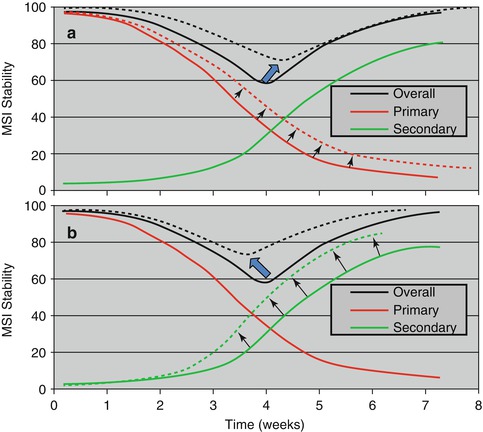
Fig 1.3
Increases in overall MSI stability associated with (a) decelerating the primary stability (old bone) curve and (b) accelerating the secondary stability (new bone) curve (Adapted from Ure et al. [17])
Ure et al. described weekly changes in MSI stability [17]. Uniquely, their study measured MSI stability in vivo over time using the Osstell Mentor (Osstell AB, Göteborg, Sweden), a device that produces an electromagnetic signal that causes the MSI to vibrate via an attached magnetic peg. The results showed significantly greater decreases in the primary stability during the first 3 weeks for MSIs that failed than for those that remained stable (Fig. 1.3a). MSIs placed in nonkeratinized tissue – most of which eventually failed – exhibited significantly greater decreases in MSI stability during the first 3 weeks than MSIs placed in keratinized tissue (Fig. 1.3b). The stability of the MSIs placed in keratinized tissue also decreased during the first 3 weeks and increased significantly thereafter, indicated healing, and increased secondary stability (Fig. 1.4). This study was important because it showed that (1) MSI failures can be predicted and (2) the primary and secondary stability curves of MSIs are similar to the curves previously described for endosseous implants [18].
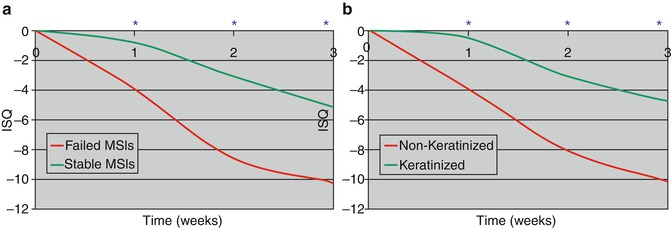
Fig 1.4
Differences (*p <0.05) in implant stability quotient (ISQ) of (a) MSIs that failed vs. those that did not fail and (b) MSIs placed in nonkeratinized vs. keratinized tissues (Adapted from Ure et al. [17])
1.3 MSI Characteristics
Ideally, MSIs should be designed to enhance their holding power (maximize pullout forces) and limit bone damage during insertion (minimize insertion torque), which together might be expected to maximize secondary stability and accelerate bone healing.
1.3.1 Effects of MSI Length
All other things being equal, the greater the surface area of the bone in contact with the MSI, the greater its primary stability. One of the easiest ways to increase the surface area, and therefore increase the primary stability of a screw, is to increase its length. The orthopedic literature has shown that implant length is one of the most important factors determining insertion torque and pullout force. When 12-mm-, 14-mm-, and 16-mm-long screws were compared, the longer screws consistently exhibited greater pullout forces [19]. The increases in holding power observed might be expected because holding power of a screw is proportional to the amount of thread engagement [20]. Insertion torque also increases with increased MSI length. Lim et al. showed that maximum insertion torque of cylindrical MSIs increased from 19.5 Ncm for 7-mm-long screws to 23 Ncm for 9-mm-long screws [21]. When 6-mm-, 8-mm-, and 10-mm-long MSIs were compared, insertion torque increased along with the length of the MSIs [22]. In one of the few studies that simultaneously evaluated insertion torque and pullout force, Shah and coworkers recently showed that the maximum insertion torque of 6-mm-long MSIs was 1.3–1.5 times greater than the insertion torque of identical 3-mm-long MSIs [23]. The effects of length were substantially greater for pullout force, which was 3.2–3.6 times greater for the 6-mm-long MSIs.
The literature evaluating long-term stability suggests that the differences in primary stability favoring longer MSIs translate in better secondary stability, but the evidence is limited. Chen et al., who evaluated 29 patients, reported 90.2 % success for their 8 mm MSIs and 72.2 % success for their 6 mm MSIs [24]. Other clinical studies have also reported greater success with longer screws, but the differences they reported were not statistically significant [25, 26].
Mortensen et al. experimentally compared 6-mm-long MSIs to identical, specially manufactured, 3-mm-long MSIs [27]. Success rates 6 weeks after immediately loading were significantly higher for the 6 mm (100 %) than the 3 mm (67 %) MSIs. However, the differences were partially due to the fact that the tips of some 3 mm MSIs sheared off during insertion, which might be expected to ream out the bone around the screw during insertion and decrease stability. Also, one of the dogs, which was described as unusually active and prone to chewing on the run bars and food bowls, accounted for 60 % of the 3 mm failures. Excluding all of the MSIs from that dog, as well as the sheared MSIs, increased the success rate of the 3 mm MSI to 90.6 %, which was still significantly lower than the success rate of the 6 mm screws. Three recent experiments using a total of 162 loaded and unloaded 3 mm MSIs confirmed an overall success rate of approximately 91 % [28–30]. Importantly, the length of the MSI shaft does not necessary equal the depth of its placement. Neither the 3 mm nor the 6 mm MSIs were completely inserted into the bone; postmortem evaluations showed that the 6 mm MSIs had been inserted 3.9 mm and the 3 mm MSIs had been inserted only 1.6 mm [31]. These findings indicate that the effect of MSI length on long-term stability is relatively small and probably related to the enhanced primary stability that longer screws provide. It also emphasizes the importance of the techniques used to insert shorter screws. While design changes are required to further enhance their stability, shorter MSI holds future potential for providing orthodontists greater versatility in terms of placement sites.
1.3.2 MSI Diameter
The literature provides only limited information on the effects of outer diameter on long-term MSI stability. Miniscrews currently on the market have outer diameters ranging from 1.2 to 2.0 mm [21, 32–43]. Diameter is important because MSIs are often placed into interradicular spaces. In order to avoid unwanted root damage by MSIs and increase the success rate, diameter is one of the factors that need to be considered in the selection of the correct dimension of MSIs.
Park et al. evaluated 227 miniscrews and showed no differences in success associated with outer diameter [44]. In contrast, Miyawaki et al., who compared MSIs with outer diameters of 1.0 mm, 1.5 mm, and 2.3 mm, reported that all of the 1.0-mm-wide MSIs failed, whereas the wider MSIs were 84–85 % successful [33]. Wiechmann et al. reported that 1.6 mm wide MSIs showed a higher success rate than the 1.1 mm MSIs [45]. Chen et al. suggested that MSIs with a diameter of less than 1.3 mm are unsuitable for insertion into a higher density bone [36]. According to Wu et al. [46], an MSI diameter equal to or less than 1.4 mm is recommended for the maxilla and an MSI diameter larger than 1.4 mm is recommended for the mandible. Poggio et al. reported that the ideal diameter for the interradicular space is 1.2–1.5 mm [43]. Based on a systematic review of the literature [35], it has been suggested that MSIs with less than 1.2 mm in diameter should be avoided.
The differences in diameter affect both the insertion torque and removal torque [14, 21, 36, 42, 45]. Using a synthetic bone model, DeCoster et al. showed that mean pullout forces increase in a linear fashion as the major diameter is increased [47]. When major diameter was doubled, pullout force almost tripled. Wider MSIs also produce higher insertion torque. Wilmes et al., who compared five different MSIs, showed that those with the largest diameters produced the greatest insertion torques [48].
Importantly, even small differences in outer diameter can have substantial effects of primary stability. Comparing 3-mm-long/1.75-mm outer diameter MSIs and 3-mm-long/2.0-mm outer diameter MSIs, Shah and coworkers showed that the extra one fourth of a mm increased insertion torque between 5.2 and 17.2 %, with greater increases in low-density than high-density bone [23]. They also showed that pullout forces increased between 15 and 27 %.
Minor diameter (i.e., inner or core diameter) of MSIs, which range between 1.2 and 1.6 mm, is also related to the holding strength of the MSI. Decoster et al. showed that when the minor diameter was increased while outer diameter was maintained, pullout forces decreased [47]. Pullout forces decreased because the major to minor diameter ratio decreased from 1.5 to 1.2. Increasing the major/minor ratio, while holding all other parameters constant, leads to small but measurable increases in pullout force. Importantly, Carano and coworkers indicated that a reduction in minor diameter of as little as 0.2 mm can reduce the screw’s resistance to breakage by 50 % [49].
MSIs with smaller diameters tend to exhibit more loosening when subjected to orthodontic force, perhaps because they may have decreased removal torque [33, 50, 51] As the diameter increases, the microdamage in the cortical bone increases, which may cause failure of the MSIs [39, 52–56].
A few studies have suggested that the maximum insertion torque level should not be beyond between 5 and 10 Ncm [27, 57, 58], but there is some disagreement regarding the optimum torque level [59–61]. In a recent systemic review [62], no evidence was provided linking specific maximum insertion torque levels with higher success rate. Even though there is no consensus concerning the ideal maximum insertion torque value, a certain level of insertion torque needs to be achieved for initial stability. Since most of the MSIs are immediately loaded, the degree of mobility that would be considered acceptable to withstand initial force remains uncertain.
When it has been determined that the best strategic location for an MSI is between roots, the available space should be carefully measured. There are differing ideas on how much alveolar bone should be left around MSIs [43, 63–65]. Root proximity is considered a major factor for MSI failure, and increasing the distance from the MSI is recommended to improve the success rate [66, 67].
However, if a MSI with a smaller diameter is selected in order to prevent root contact, it may increase the fracture risk. In an animal study, Buchter et al. [68] reported that eight of 200 MSIs were fractured at the time of insertion and two failed at the time of removal. In a human study, Park et al. reported that eight of 227 MSIs were fractured in 87 consecutive patients [44]. The fracture risk increases as the diameter of MSIs decreased [36, 40, 57, 69, 70]. The core diameter seems to be a more important factor for fracture torque than the outer diameter [70, 71].
The larger the difference between the maximum insertion torque and/or maximum removal torque and maximum fracture torque, the smaller the fracture risk. If a MSI with a smaller diameter has to be used because of a limited amount of space, a pilot hole may be necessary in order to reduce the fracture risk. According to the recent study [72], the diameter does not have a significant effect on the amount of linear microdamage with pilot drilling.
Duaibis et al. [38] evaluated the various types of stress in the cortical bone around MSIs using a three-dimensional finite element study. They concluded that the most critical MSI design factors affecting the stability are the diameter and extra-bony head length, with larger diameters and shorter heads developing less stress in the cortical bone surrounding the MSI. Another study [73] evaluating risk factors showed that diameter was not related to primary stability. Park et al. [44] and Wu et al. [46] reported that diameter did not make any difference with respect to success rates.
1.3.3 Effects of Pitch
Another way to increase the surface area and primary stability of MSIs is to decrease their pitch (i.e., the distance between the threads). It is well established that pitch increases the purchase strength of screws in porous materials. Decreasing pitch increases the pullout forces of bone screws [47, 74]. Migliorati and coworkers reported that pitch had a significant effect on maximum insertion torque (i.e., the smaller the pitch, the greater the torque), but not on pullout forces [14]. However, their results were potentially confounded because they compared four screws that differed in terms of their major diameter, length, as well as the depth of thread.
Brinley and coworkers compared MSIs that differed only in terms of pitch [75]. All of the MSIs were made of surgical grade titanium, they were 6-mm long, and they were self-drilling and self-tapping. The MSIs had major and minor diameters of 1.8 mm and 1.6 mm, respectively, the threads had a 90° asymmetric buttress design, and the apical 3 mm of the MSIs were tapered. MSIs with 1.0-mm, 0.75-mm, and 1.25-mm pitch were compared. When the MSIs were placed in synthetic bone, insertion torque increased with decreases in pitch. However, the differences were small and not statistically significant (p = 0.275). Compared to the 1.0-mm pitch screws, the insertion torque of the 0.75- and 1.25-mm pitch MSIs were only 7.2 % greater and 3.6 % less, respectively (Fig. 1.5). In contrast, pullout forces were over 105 % greater with the 0.75-mm than 1.0-mm pitch MSIs; the pullout forces of 1.25-mm pitch MSIs were approximately 18 % greater than the 1.0-mm MSI. The effects of pitch on pullout were clearly associated with bone density. When the MSIs were inserted in denser human cadaver bone, the differences were still significant, but insertion torque and pullout forces were only 11.8 % and 7.1 % greater, respectively, for the 0.75-mm than for the 1.0-mm pitch MSIs. Together, these results suggest that changes in pitch might be an effective approach for increasing stability in patients with immature or thin cortical bone.
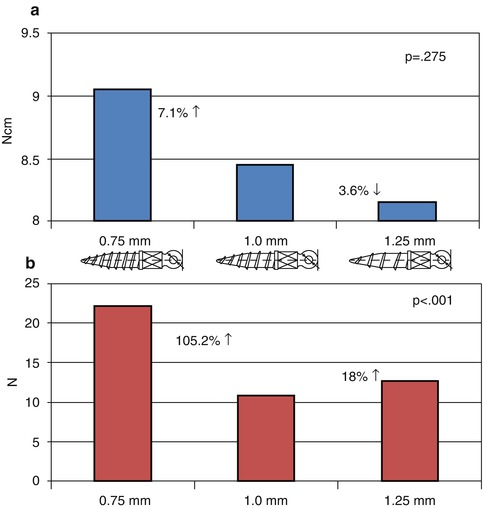
Fig 1.5
The effects of MSI pitch on (a) insertion torque and (b) pullout strength testing using synthetic bone, with percent changes based on the 1.0 mm pitch MSI (Adapted from Brinley et al. [75])
1.3.4 Thread Design
The thread design of an MSI can vary in pitch, depth, and shape. Modification of thread designs can substantially affect the mechanical properties. Wilmes et al. [71] reported that the thread shape affects the MSI’s primary stability. The thread design influenced the resistance to pullout of the MSIs [76, 77]. There are reports of MSIs with asymmetric thread designs showing better mechanical properties [49, 78]. The buttress reverse thread shape has been shown to produce higher pullout strength values than the other designs [76]. Duaibis et al. [38] reported that the thread depth affected the stress in the cortical bone, but the thread shape and pitch did not influence the stress in the cortical bone.
MSIs with greater thread depth exhibit higher primary stability [47, 74, 79]. The ratio between thread depth and pitch has been described as a thread-shape factors [80], with smaller pitch and greater depth showing greater pullout strength [14, 74, 81]. If the thread depth is increased while the external diameter is maintained, the pullout strength is increased up to a certain point. However, this may increase the fracture risk depending on the reduction in core diameter. Chang et al. [77] suggested that MSIs perform better with a core/external diameter ratio of 0.68.
Dual or double thread-shape MSIs have two different pitches. A smaller pitch is on the upper part of the MSI to increase the contact between the upper part of the MSI and the cortical bone (Fig. 1.6). The dual thread shape showed a lower insertion torque and a higher removal torque than either the cylindrical or conical shapes [82]. The dual thread shape exhibited a more gradual increase of insertion torque and a more gradual decrease of removal torque. It also had higher removal torque than the single thread shape. This suggests that the dual thread MSIs might be less harmful to the surrounding bone tissue and improve the stability. However, the dual thread shape needs a longer insertion time which might create excessive heat and lead to tissue damage. Pilot drilling and slower rotational maneuvering during MSI insertion may be helpful for preventing possible negative effects on the tissues.
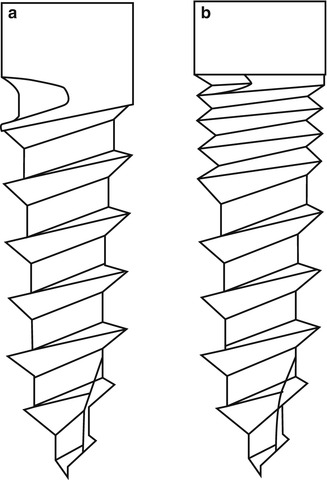
Fig 1.6
(a) Single thread shape MSI, (b) dual thread shape MSI
1.3.5 Fluting
Flutes might be expected to either decrease insertion torque if they increase the clearance of bone debris accumulating around the threads or increase torque if the flute dimensions allow bone chips and debris to accumulate around the threads [79, 83]. This could explain why flutes have been reported to both decrease [84, 85] and increase [83, 86, 87] pullout forces of endosseous and surgical screws. Wu et al. showed that fluting (depending on the shape) played a significant role in reducing the insertion torque of endosseous implants [88]. Decreased insertion torque and cortical damage have been shown to occur with increases in the number and lengths of cutting flutes at the bottom of cortical bone screws [83]. In contrast, the number and length of the flutes increased pullout forces.
Brinley and coworkers evaluated the effects of three longitudinal flutes, extending the full length of the threaded portion of an MSI, on insertion torque and pullout forces [75]. The depth of the flutes, which were 0.25-mm wide, extended through the threads to the core; the surfaces of the flutes were cut to facilitate placement and removal. Results showed even more pronounced differences between synthetic and cadaver bone for fluting than for pitch. In the softer synthetic bone, insertion torque and pullout were 15 and 400 % larger, respectively, for the fluted MSIs (Fig. 1.7). In the denser cadaver bone, insertion torque increased 120 %, while pullout increased only 65 %. Once again, this suggests that fluting might be used to enhance the primary stability of screws placed in thinner, less dense bone.
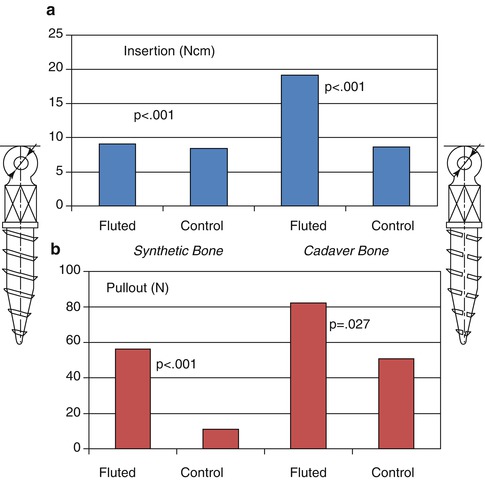
Fig 1.7
The effects of MSI longitudinal fluting on (a) insertion torque and (b) pullout strength testing using synthetic and cadaver bone, with percent changes based on the 1.0 mm pitch MSI (Adapted from Brinley et al. [75])
1.3.6 SLA Surface Treatment
Surface modifications of endosseous implants have proven to be one of the best ways to increase surface area, accelerate secondary stability, and enhance osseointegration. Endosseous implants with surfaces that are sandblasted large grit and acid etched (SLA) exhibit significantly greater shear strength than machined surfaces [89]. SLA surfaces also increase the rate of osseointegration [90, 91]. The effects that SLA surfaces have on MSIs are controversial and studies remain limited. Kim and coworkers reported significantly lower insertion torque and significantly higher total removal energy (energy [J] required to remove MSI from maximum torque thru time of removal) for SLA than machined MSIs, but no differences in removal torque [92]. Based on 144 MSIs placed in rabbit tibias, Chang et al. reported considerably higher BIC after 12 weeks for those that had SLA surfaces than those with machined surfaces [91]. More recently, Mo and coworkers showed that SLA MSIs (9.5 × 1.8 mm) produced significantly greater removal torque than machined MSIs (8.2 vs. 5.8 Ncm, respectively) during the first 6 weeks after placement, but no differences after 10 weeks [93]. The actual effects of SLA modification on the bone in the vicinity of the MSI remain poorly understood.
Using a split-mouth design, 21 SLA MSIs were compared to 21 machine-surfaced MSIs [94]. The MSIs were placed in seven mature foxhounds and immediately loaded with a 200-g force, which was maintained for 9.2 weeks. Micro-CT (μCT) was used to determine the absolute and relative amounts of bone surrounding the MSI. Two very thin layers of bone surrounding the MSI were evaluated; one layer included the bone located 6–24 μm from the MSI surface; the other layer included the bone located 24–42 μm from the MSI. The results showed significantly (p <0.05) greater amount of the bone (5–21 %) around the SLA than machined MSIs (Fig. 1.8). SLA coating had the greatest effects in the most coronal aspects of both the cortical and non-cortical sections evaluated, which were the regions exhibiting the greatest bone volume to total volume.
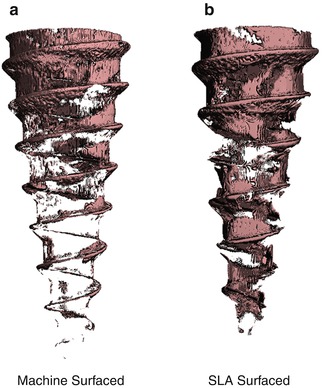
Fig 1.8
Micro-CT of bone located 6–24 μm from the surface of (a) machine-surfaced and (b) sandblasted large-grit acid-etched (SLA) MSIs (Adapted from Ikeda et al. [94])
1.3.7 Shaft Design
Conical and cylindrical shafts are the most common shapes of MSIs (Fig. 1.9). The conical MSIs showed a higher insertion torque and greater primary stability than the cylindrical MSIs [39, 71, 95–100]. A conical shape provides tight contact between the tissue and the MSI because the upper part of the screw has a larger diameter than the lower part [101]. Drago and Del Castillo reported that the conical MSIs have less surface area than cylindrical shape MSIs [102], and small surface contact area with the bone may compromise MSI stability [100, 103]. However, Kim et al. [100] reported that there was no significant difference in bone-to-implant contact and bone area between conical and cylindrical MSIs.
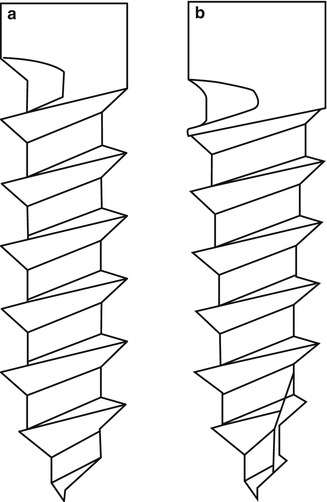
Fig 1.9
Shaft design. (a) Cylindrical shaft MSI, (b) Conical shaft MSI
The effects of shaft design remain controversial. One study reported that the cylindrical MSIs showed a greater pullout strength [95]. Conversely, another study showed that conical MSIs showed greater pullout resistance than cylindrical MSIs [104].
The conical-shaped MSIs produced higher stress in the cortical bone during insertion than the cylindrical shape [105]. This explains why conical MSIs caused greater microdamage to the cortical bone than cylindrical MSIs [39]. As previously indicated, tight contact can cause excessive insertion torque which may lead to microfracture, ischemia of the surrounding bone, delayed bone healing, and eventually the failure of the MSIs [53, 54, 99, 106].
While conical MSIs require greater maximum removal torque, torque values decrease more rapidly during the removal procedure than with cylindrical MSIs [82]. Perhaps this is why it has been suggested that the conical shape has less risk of fracture during the removal procedure [107].
Considering all the results from the various studies, the conical MSI has an advantage regarding primary stability. However, the cylindrical MSI is better in terms of secondary stability. It causes less tissue damage because it produces lower maximum insertion torque [39, 105]. Sakoh et al. [99] reported that conical MSIs showed higher failure rates than cylindrical MSIs in an in vitro study. In one animal study, there was no difference in the failure rates [100]. Another animal study [97] showed that the conical MSIs had better primary stability compared to cylindrical MSIs, but no difference between the two screws in secondary stability [39]. A human study evaluating, 324 MSIs in 144 patients found no significant difference in the success rate between the cylindrical and conical shape [108].
Kim et al. [100] suggested that the conical shape may require modifications in the thread structure and the insertion technique in order to reduce the excessive insertion torque while maintaining a high resistance to removal. Chang et al. [77] reported that increasing the core diameter of the uppermost threads to create a conical core design could reduce the stress concentration effects at the neck while improving the pullout resistance.
It is still not clear which shaft design has an advantage for clinical success. Further research is needed to improve the primary stability without sacrificing the secondary stability.
1.4 Placement Site Characteristics
As previously indicated, the anchorage an implant provides depends on its interaction with the bone into which it has been inserted. Differences in the quality and quantity of bone into which MSIs are placed might therefore be expected to influence both their primary and secondary stability. It has been suggested that cortical bone quantity and quality are two of the most important determinants of primary stability [33, 50].
1.4.1 Bone Density/Quality
Bone density is positively related to both insertion torque and pullout strength [13, 109–114]. The higher the density of the bone, the greater the primary stability. This can again be explained by higher initial bone-to-implant contact.
In order to better understand how bone density affects the primary stability of MSIs, Hung et al. evaluated the insertion torque and pullout forces of MSIs placed in synthetic cortical bone [115]. Cortical densities of 0.8 and 0.64 g/cc (the density of the human mandible has been reported to be 0.66 g/cc) were compared (Fig. 1.10). While the MSIs placed in more dense bone exhibited significantly greater insertion torque and pullout force than the MSIs placed in less dense bone, the effects were greater on insertion torque (156 % increase) than on pullout force (135 % increase).
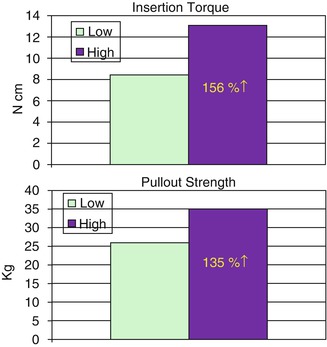
Fig 1.10
Effects of synthetic cortical bone density [high (0.8 g/cc) vs. low (0.64 g/cc)] on the insertion torque and pullout strength of 6-mm-long MSIs (Adapted from Hung et al. [115])
In their recent meta-analysis of 17 studies, Papageorgiou et al. reported greater MSI failures in the mandible than maxilla (19.3 vs. 12.0 %) [8]. Greater numbers of failures might be expected for denser bone due to increases in microfractures. Moreover, greater MSI failures in the mandible than maxilla might be due to ischemia and necrosis associated with overheating when drilling into denser bone [116, 117]. Increased microfractures, ischemia, and necrosis might be expected because bone mineral density has consistently been shown to be greater in the human mandible than maxilla [118–120].
Experimental studies make it possible to better evaluate stability by controlling confounding variables. A total of 106 immediately loaded MSIs (6-mm long and 1.8-mm wide) were placed and followed for either 98 [121, 122] or 105 days [123]. Of the 53 MSIs that were placed in the maxillary, 52 (98.1 %) remained stable compared to 49 (92.5 %) of their counterparts placed in the mandible (Table 1.1). This suggests that failures may be higher in the mandible, but the differences between jaws were small and not statistically significant (p = 0.119). Another experimental study (Fig. 1.11) comparing 3-mm MSI loaded with 600 g also showed significantly (p <0.001) greater stability over a 6-week observation period for MSI placed in the maxillas (80 % success) than for those placed in the mandibles (60 % success) of the same dogs [27].
Table 1.1
Differences in the stability of immediately loaded MSIs (6-mm long × 1.8-mm wide) placed in the maxilla and mandible
Study
|
Maxilla
|
Stability (%)
|
Mandible
|
Stability (%)
|
---|---|---|---|---|
1
|
Control
|
14/14 (100 %)
|
Control
|
13/14 (92.8 %)
|
1
|
25 g
|
7/7 (100 %)
|
25 g
|
5/7 (71.4 %)
|
2
|
50 g
|
16/16 (100 %)
|
50 g
|
16/16 (100 %)
|
2
|
100 g
|
15/16 (93.8 %)
|
100 g
|
15/16 (93.8 %)
|
Total
|
52/53 (98.1 %)
|
49/53 (92.5 %)
|
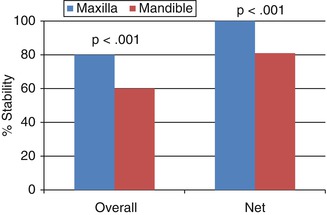
Fig 1.11
Overall and net stability of MSIs placed without pilot holes in the maxillas and mandibles of mature beagle dogs and immediately loaded with 600 g (Adapted from Mortensen et al. [27])
1.4.2 Cortical Bone Thickness
Clinical failures of MSIs have been commonly attributed to thick cortical bone [33, 82, 109, 124]. On the other hand, thin cortical bone can also be problematic. For example, Papageorgiou et al. reported significantly more failures in cortical bone that was <1-mm thick than in thicker cortical bone (21.3 vs. 8.3 %) [8]. Thin cortical bone could explain the higher failure rates that have been attributed to younger patients [110, 124–126]. There are also differences in cortical thickness between jaws and within regions of each jaw that hold important clinical implications [127–131].
The thicker the cortical bone, the higher the insertion torque and pullout force [13, 111] indicating greater primary stability. For example, Pithon et al. showed that insertion torque was approximately 4 times greater in 6-mm than 1-mm-thick cortical bone [22]. Using synthetic bone to control extraneous sources of variation, Shah et al. showed that increasing cortical thickness from 1 to 2 mm increased both insertion torque and pullout force [23]. Importantly, the effects were greater on insertion torques than pullout force (Fig. 1.12).
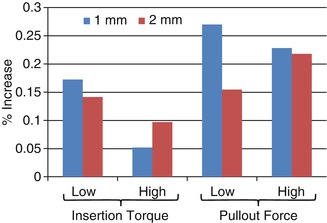
Fig. 1.12
Relative increases of insertion torque and pullout force by increasing the major diameter of 3-mm-long MSIs by ¼ mm (Adapted from Shah et al. [23])
Because there had been no comprehensive comparisons of cortical bone thickness at common placement sites of the maxilla and mandible, Farnsworth et al. quantified cortical bone thickness at 16 commonly used sites [132]. Cone beam computed tomography (CBCT) images of 26 adolescents (11–16 years of age) and 26 adults (20–45 years of age) showed that adult cortical bone was significantly thicker (0.12–0.56 mm) at all sites except the infrazygomatic crest, the most posterior palatal site, and the mandibular buccal site between the 6 and 7. Cortical bone at the buccal mandibular sites was significantly thicker in the posterior than anterior regions; the cortex was more than twice as thick between the first and second molar (2.4–2.5 mm) than between the lateral incisors and canines (0.9–1.2 mm) (Fig. 1.13). Cortical bone in the buccal aspect of the maxilla tended to be thinner than in the buccal aspect of the mandible. Also, differences between sites were much smaller in the maxilla; the cortex was only slightly thicker between 5–6 (1.0–1.5) and 4–5 (1.1–1.3) than between 2–3 (1.0–1.2) and 6–7 (0.9–1.2). Cortical bone thickness and differences between sites in the lingual region of the maxilla were similar to the buccal region of the maxilla. Paramedian palatal cortex was slightly thicker 3 mm (1.3–1.4 mm) than 6 mm (1.1–1.2 mm) behind the incisive foramen, which was in turn slightly thicker than bone located 9 mm (1.0–1.2) behind the foramen. The cortical bone in the infrazygomatic crest (1.4–1.6 mm) more closely approximated cortical thickness of the mandible than maxilla. Importantly, variability in cortical thickness among individuals was substantial; it would not be unusual for an adolescent patient to present with maxillary cortices less than 1.0-mm thick, which could be problematic for MSI retention. Conversely, it is not uncommon for mandibular cortices between the first and second molars to be more than 2.5-mm thick, which could also be problematic when the bone is dense.
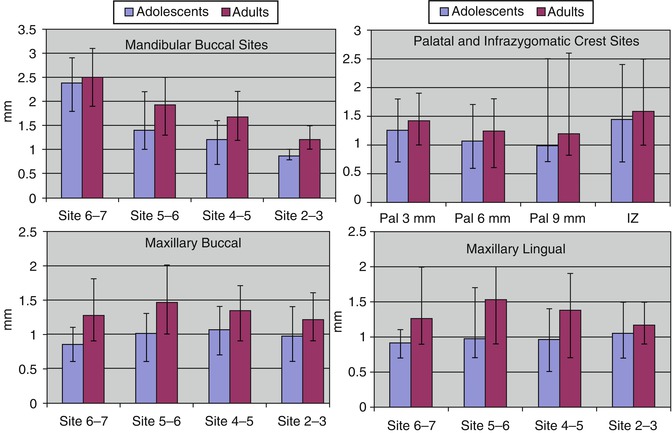
Fig. 1.13
Cortical bone thickness (means and ranges) for mandibular and maxillary interradicular MSI sites (Adapted from Farnsworth et al. [132])
It has also recently been shown that the cortical bone and the alveolar ridge are thicker in hypodivergent than in hyperdivergent subjects [133]. In the maxillary buccal region, differences were small, ranging from 0.13 to 0.33 mm (Fig. 1.14). Differences were larger in the maxilla, especially between the molars. The thickness of the dentoalveolar ridges measured 5 mm from the crests were up to 1.77-mm thicker in hypodivergent adults, and the differences were due primarily to differences in cortical rather than medullary bone thickness. They also showed that lingual cortical bone was consistently thicker in the mandible than maxilla; in the mandible, bone in the posterior region was thicker.
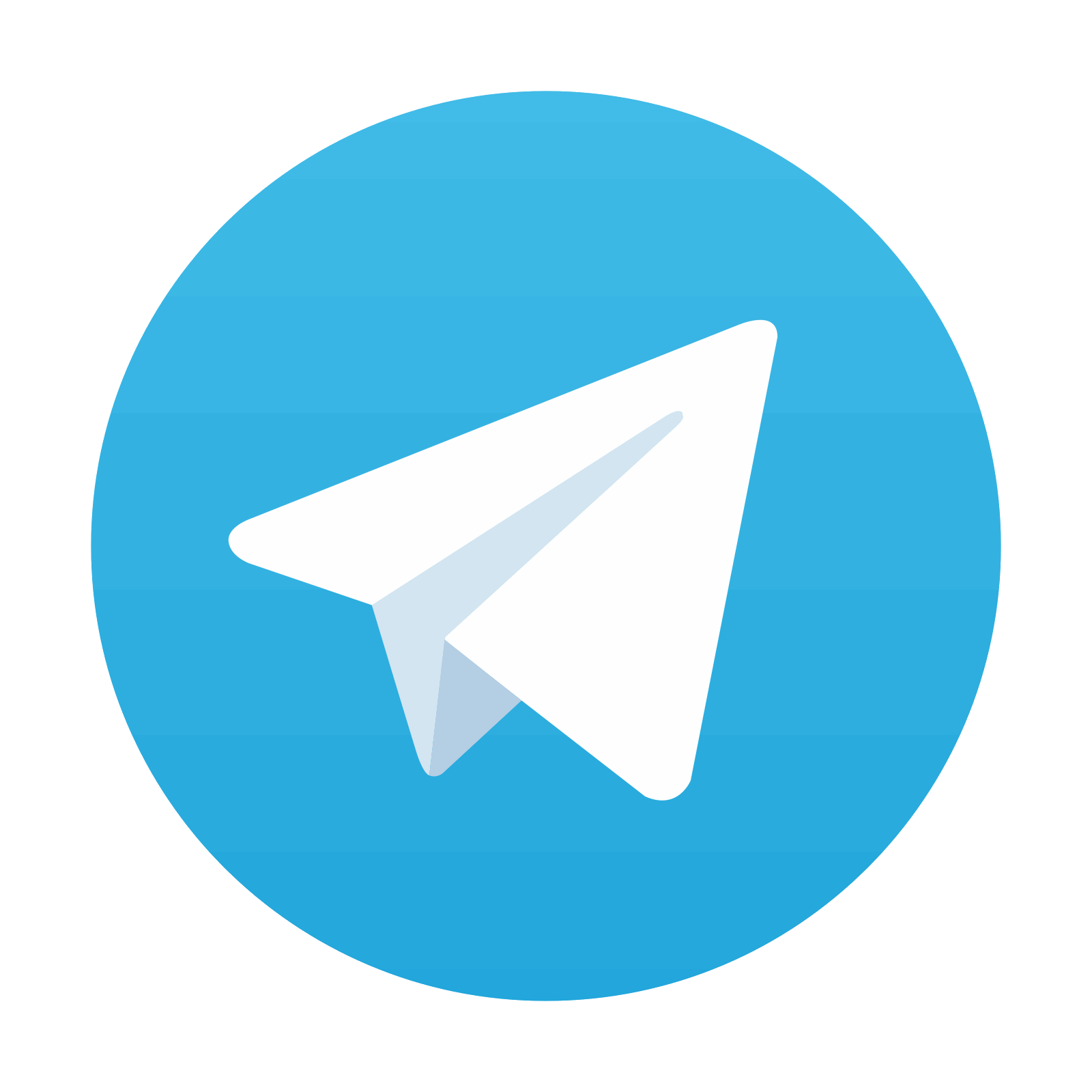
Stay updated, free dental videos. Join our Telegram channel

VIDEdental - Online dental courses
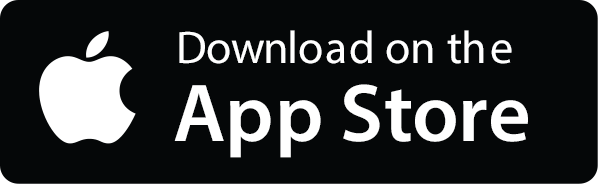
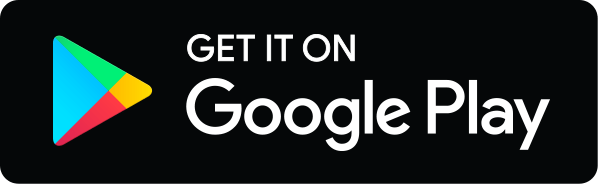