2. Clinical Evaluation and Laboratory Wear-Testing Methods
Zhong-Rong Zhou1 , Hai-Yang Yu2, Jing Zheng1, Lin-Mao Qian1 and Yu Yan3
(1)
Tribology Research Institute, Southwest Jiaotong University, Chengdu, People’s Republic of China
(2)
West China College of Stomatology, Sichuan University, Chengdu, People’s Republic of China
(3)
Institute of Advanced Materials and Technology, University of Science and Technology, Beijing, People’s Republic of China
Abstract
Being the hardest organ in body, human teeth have a very unique structure. To understand the wear behavior of human teeth, the properties of materials and structures need to be evaluated. There are several quantitative and qualitative methods to measure or assess these properties. This chapter reviews evaluation techniques to understand their unique properties for both mammal and human teeth. Chapter 6 gives a more detailed discussion on artificial dental materials.
Being the hardest organ in body, human teeth have a very unique structure. To understand the wear behavior of human teeth, the properties of materials and structures need to be evaluated. There are several quantitative and qualitative methods to measure or assess these properties. This chapter reviews evaluation techniques to understand their unique properties for both mammal and human teeth. Chapter 6 gives a more detailed discussion on artificial dental materials.
2.1 Clinical Evaluation Methods and Diagnosis
A number of systems for the classification and measurement of tooth surface loss have been reported in the literature.
Scott presented an ordinal dental attrition scoring technique for molar teeth involving a quadrant system [1]. Molar teeth were divided into four sections and each section scored on a 1–10 scale, as described in Table2.1. The sum of the four quadrants is the score for that tooth. Such wear score categories were quickly learned and proven useful in the analysis of dental wear data.
Table 2.1
Attrition scoring technique
Score
|
Description
|
---|---|
0
|
Wear facets invisible or very small.
|
1
|
Wear facets large, but large cusps still present and surface features (crenulations, noncarious pits) very evident. It is possible to have pinprick-size dentin exposures or “dots” that should be ignored. This is a quadrant with much enamel.
|
2
|
Any cusp in the quadrant area is rounded rather than being clearly defined as in 2. The cusp is becoming obliterated but is not yet worn flat.
|
3
|
Quadrant area is worn flat (horizontal), but there is no dentin exposure other than a possible pinprick-size “dot.”
|
4
|
Quadrant is flat, with dentin exposure one fourth of quadrant or less. (Be careful not to confuse noncarious pits with dentin exposure.)
|
5
|
Dentin exposure greater: More than one fourth of quadrant area is involved, but much enamel is still present. If the quadrant is visualized as having three “sides,” the dentin patch is still surrounded on all three “sides” by a ring of enamel.
|
6
|
Enamel is found on only two “sides” of the quadrant.
|
7
|
Enamel on only one “side” (usually outer rim), but the enamel is thick to medium on this edge.
|
8
|
Enamel on only one “side” as in 8, but the enamel is very thin, just a strip. Part of the “edge” may be worn through at one or more places.
|
9
|
No enamel on any part of quadrant: dentin exposure complete. Wear is extended below the cervicoenamel junction into the root.
|
A few years after Scott’s scoring system, Eccles developed a classification system for erosion due to nonindustrial causes; it indicates the severity and site of a lesion on each tooth [2]. Three types of lesions and four kinds of surfaces were possible, as listed in Table 2.2. Eccles believed that this may be of value in describing the condition and as a guide to treatment.
Table 2.2
Classification of erosion due to nonindustrial causes
Class
|
Type of lesion
|
---|---|
I
|
Superficial: enamel only
|
II
|
Locallized: <1/3 into dentin
|
III
|
Extensive: >1/3 into dentin
|
Letter
|
Surface denoted
|
---|---|
a
|
Labial
|
b
|
Lingual or palatal
|
c
|
Occlusal or incisal
|
d
|
Multisurface
|
Around the same time as Scott [1], a method was presented for quantitative measurement based upon the samples of modern and prehistoric hunter-gatherers and agriculturalists. An eight-stage system of occlusal wear was fully described, shown here in Table 2.3. Through an analysis of molar wear, Smith [6] believed that a change in the wear plane angle should be an early sign of a change in diet, and that flatness of molar wear appeared to be a good indicator of change in food or food preparation.
Table 2.3
Descriptions of stages of occlusal surface wear
Molars
|
Premolars
|
Diagnostic criteria
|
|
---|---|---|---|
1
|
Unworn to polished or small facets (nondentin exposure)
|
Unworn to polished or small facets (nondentin exposure)
|
Unworn to polished or small facets (nondentin exposure)
|
2
|
Moderate cusp removal (blunting)
Thinly enameled teeth may show cusp tip dentin, but human permanent molars show no more than one or two pinpoint exposures
|
Moderate cusp removal (blunting)
|
Point or hairline of dentine exposure
|
3
|
Full cusp removal and/or some dentin exposure, pinpoint to moderate
|
Full cusp removal and/or moderate dentin patches
|
Dentin line of distinct thickness
|
4
|
Several large dentin exposures, still discrete
|
At least one large dentin exposure on one cusp
|
Moderate dentin exposure no longer resembling a line
|
5
|
Two dentinal areas coalesced
|
Two large dentin areas (may be slight coalesced)
|
Large dentin area with enamel rim complete
|
6
|
Three dentinal areas coalesced, or four coalesced with enamel island
|
Dentinal areas coalesced, enamel rim still complete
|
Large dentin area with enamel rim lost on one side or very thin enamel only
|
7
|
Dentin exposed on entire surface Enamel rime largely intact
|
Full dentin exposure, loss of rim on at least one side
|
Enamel rim lost on two sides or small remnants of enamel remain
|
8
|
Severe loss of crown height, breakdown of enamel rim; crown surface takes on shape of roots
|
Severe loss of crown height, crown surface takes on shape of roots
|
Complete loss of crown, no enamel remaining; crown surface takes on shape of roots
|
Human tooth wear occurs so slowly that traditionally it has needed months or years to be measurable. Teaford and Tylenda [3] proposed that microscopic changes in wear patterns on human teeth detected in a matter of days could be used as indicators of rates of wear. Particularly, they emphasized that the creation of new wear features on a surface may only be a good indicator of a certain type of wear (e.g., abrasion) and not others (e.g., erosion). Through their approach, clinicians could modify their procedures in response to changes in tooth use.
A system was introduced by Johansson et al. [4] for the longitudinal evaluation of the severity and the rate of tooth wear based upon clinical observations. Five scales were used for grading the severity and four scales for scoring the progression of occlusal wear, as indicated in Tables 2.4 and 2.5, respectively. The advantage of this system is that it is simple and reliable for clinical treatment.
Table 2.4
Ordinal scale used for grading severity of occlusal wear
Score
|
Diagnostic criteria
|
---|---|
0
|
No visible facets in the enamel. Occlusal/incisal morphology intact
|
1
|
Marked wear facets in the enamel. Occlusal/incisal morphology altered
|
2
|
Wear into the dentin. The dentin exposed occlusally/incisally or adjacent tooth surface. Occlusal/incisal morphology changed in shape, with height reduction of the crown
|
3
|
Extensive wear into the dentin, large dentinal area (>2 mm2) exposed occlusally/incisally or adjacent tooth surface. Occlusal/incisal morphology totally lost locally or generally. Substantial loss of crown height
|
4
|
Wear into secondary dentin (verified by photographs)
|
Table 2.5
Scale used for scoring progression of occlusal wear
Score
|
Diagnostic criteria
|
---|---|
0
|
No definite change in previously recorded area(s)
|
1
|
Visible change, such as an increase of the facets area(s), without any measurable reduction of crown length; occlusal/incisal morphology changed in shape compared to the first examination
|
2
|
Measurable reduction of crown length, <1 mm
|
3
|
Marked reduction of crown length, >1 mm
|
A similar diagnostic criteria on tooth erosion was presented by Millward et al. [5], given in Table 2.6, through an investigation involving the clinical examination of 101 children and an assessment of their dietary habits. It provided a similar method as that proposed by Eccles [2]. Three kinds of tooth erosion, such as none or mild, moderate, and severe erosion, corresponding to different criteria and different tooth surfaces were classified for the children.
Table 2.6
Diagnostic criteria for tooth wear index. B buccal or labial, L lingual or palatal, O occlusal, I incisal
Score
|
Diagnostic criteria
|
Surfaces
|
---|---|---|
0
|
No loss of enamel surface characteristics
|
B, L, O, I
|
1
|
Loss of enamel surface characteristics
|
B, L, O, I
|
2
|
Loss of enamel, visible dentin on less than one third of the surface area
|
B, L, O
|
Loss of enamel with visible dentin
|
I
|
|
3
|
Loss of enamel, visible dentin on more than one third of the surface area
|
B, L, O
|
Loss of enamel and substantial loss of dentin but not exposing pulp or secondary dentin
|
I
|
|
4
|
Complete loss of enamel, pulp exposure, or exposure of secondary dentin
|
B, L, O
|
Pulp exposure, or exposure of secondary dentin
|
I
|
To conclude, several groups have tried to provide scoring systems or a classification of stages of tooth wear. Most of the classifications are closely related to clinical cases and based on statistical data from patients. Each individual scoring system is suitable for a set of particular cases. We should not really question their reliability for universal applications. As a matter of fact, understanding the philosophy and methodology behind each rating system is much more important for clinicians and scientific researchers than questioning their reliability.
2.2 Wear Modes and Location
2.2.1 Wear Modes
Friction and wear can result from direct contact between teeth and from any abrasive particles or devices sandwiched between them during mastication, thegosis, bruxism, toothbrushing, and other functions. Tooth surface loss caused by wear is a common clinical problem, with various epidemiologic studies suggesting prevalence estimates of up to 97 %, with around 7 % of the population showing pathological wear requiring treatment [6]. The main categories of wear that contribute to the destruction of natural teeth and artificial materials are classified as follows [7]: physiological wear (vital life functions); pathological wear (disease and abnormal conditions); prophylactic wear (preventive conditions); and finishing procedure wear. An overview is provided in Table 2.7.
Table 2.7
Classification of wear situations in dentistry
Interoral wear event
|
Type of wear
|
Substrate
|
Opponent
|
||
---|---|---|---|---|---|
Physiological causes of wear
|
|||||
Noncontact wear
|
Three-body
|
Saliva/food
|
Tooth/restoration
|
—
|
Food
|
Direct contact wear
|
Two-body
|
Saliva
|
Tooth/restoration
|
Tooth/restoration
|
—
|
Sliding contact wear
|
Two-body
|
Saliva
|
Tooth/restoration
|
Tooth/restoration
|
—
|
Pathological causes of wear
|
|||||
Two-body
|
Saliva
|
Tooth/restoration
|
Tooth/restoration
|
—
|
|
Two-body
|
—
|
Tooth/restoration
|
Tooth/restoration
|
—
|
|
Erosion
|
—
|
Saliva
|
Tooth/restoration
|
—
|
—
|
Unusual habits
|
Two-body
|
Saliva
|
Tooth/restoration
|
Foreign body
|
—
|
Prophylactic causes of wear
|
|||||
Toothbrush and dentifrice
|
Three-body
|
Water
|
Tooth/restoration
|
Toothbrush
|
Dentifrice
|
Prophylactic pastes
|
Three-body
|
Water
|
Tooth/restoration
|
Polishing cup
|
|
Scaling and cleaning
|
Two-body
|
Water
|
Tooth/restoration
|
Instrument
|
—
|
Cutting, finishing, polishing
|
|||||
Cutting burrs/diamonds
|
Two-body
|
Water
|
Tooth/restoration
|
—
|
|
Finishing burrs
|
Two-body
|
Water
|
Tooth/restoration
|
—
|
|
Polishing pastes
|
Three-body
|
Water
|
Tooth/restoration
|
Polishing cup
|
Abrasiveslurry
|
Physiological wear, inevitably due to the function of mastication [8–11], is surface degradation that results in a progressive, very slow loss of the convexity of tooth cusps, which manifests as a flattening of both cusp tips on the posterior teeth and incisal edges on the anterior teeth for mammals. Compared with physiological wear, some pathological factors can cause excessive wear of teeth and restorations [9]. Clinical reports show that wear usually becomes significantly severe due to erosion, bruxism, xerostomia, and so on. Pathological wear can also be caused by detrimental oral habits [8, 12], which generally include chewing tobacco; biting on hard objects such as pens, pencils, or pipe stems; opening hairpins with teeth; and biting fingernails. In addition, occupational habits may result in the wear of teeth and restorations. For instance, tailors or seamstresses sever thread with their teeth, shoemakers and upholsterers hold nails between their teeth, glassblowers and musicians play wind instruments, and so on.
It was pointed out in Chapter 1 that in normal use, the benefits of toothbrushing far outweigh the potential harm; however, wear can occur as a result of overzealous toothbrushing and the improper use of dental floss and toothpicks. Scaling and cleaning have been shown to result in minor wear of teeth. Dental treatments usually involve such processes as cutting, finishing, and polishing; and they cause the wear of teeth and restorations to some extent [7]. The actual wear situation in the mouth may vary considerably because of different substrates, opposing wear surfaces, lubrication systems involved, and third-party abrasives [13]. The mouth provides an extremely complex tribological system. Therefore, the wear of teeth and restorations is multifactorial in the mouth and dependent on physical and chemical conditions [14]. It has long been recognized that it is difficult to ascribe many individual cases to any one category [9].
2.2.2 Wear Location
Wear of teeth and restorations occurs mainly at sites of occlusal surfaces and incisal surfaces during mastication, thegosis, and bruxism. Masticatory wear also can occur on the lingual and buccal aspects of teeth, as coarse food is forced against these surfaces by the tongue, lips, and cheeks during mastication. Prophylactic wear, which is caused by toothbrushing, scaling, cleaning, etc., can occur on the buccal, lingual, occlusal, and approximal aspects of teeth. It was reported that the occlusal surface showed the greatest wear, and the cervical, lingual, and buccal showed the least, respectively [15]. Notable is that the approximal wear at sites of proximal contacts is often forgotten [16]. Regarding the tooth position, the first molar showed the greatest degree of wear, while the canine and premolar showed the least, respectively. As for the tooth location, mandibular occlusal surfaces showed greater wear than maxillary occlusal surfaces in all age groups [17].
A vast amount of literature is available concerning the occlusal wear of dental materials. Most studies made a distinction between occlusal contact area (OCA) and contact-free occlusal area (CFOA) wear [18]. The occlusal contact area is the region where opposing materials contact directly (including tooth–tooth, tooth–restoration, and restoration–restoration). It usually represents the region where two-body wear can occur. Contact-free occlusal area is the region where only three-body wear occurs, caused by food particles, toothpaste, and other physical objects. In addition, for dental implants, fretting can occur at the interface between the implant and alveolar bone during occlusal movements [19, 20]. Fretting has been regarded as one of the causes of dental implant failure, which can result in implants’ loosening.
2.3 Wear-Testing Methods
A literature survey shows that three kinds of methods are in use today by various workers to study the tribological behaviors of dental materials: in vivo observation and measurement; in vitro laboratory simulation; and in situ testing. In vivo observation and measurement is generally used by clinicians to observe and evaluate clinical manifestations of the wear of teeth and restorations in the mouth, while in vitro laboratory simulation is usually used by materials and tribology researchers to explore wear mechanisms of natural teeth and artificial materials. More recently, the in situ method has been introduced and developed to investigate dental tribology [14].
2.3.1 In Vivo Observation and Measurement
In vivo methods are widely used in clinical practice. Clinical observation on the loss of dental hard tissue caused by wear can be traced back hundreds of years [21].
As mentioned above, both the oral environment and biomechanics are very complex; tooth wear is therefore multifactorial in the mouth, and physical and chemical processes interact. Thus, it has been accepted that the main advantage of in vivo methods is to obtain and examine the tribological behavior of teeth and restorations resulting from real oral environments and biomechanics [9, 18]. However, in vivo methods have some disadvantages that limit their contribution to the tribology of dental materials. First, it is impossible for in vivo methods to isolate and study individual wear processes, including attrition, abrasion, and erosion. Although some measures can be taken to unify testing conditions among subjects to some extent, it is still evident that subjectivity is indeed a variable, which leads to problems in interpreting results [14, 18]. Second, the lack of control over important variables that may influence tribological behavior (such as chewing force, dietary intake, or environment factors) greatly limits their contribution to the tribology of dental materials, especially wear mechanisms. In addition, it is clear that wear processes cannot be accelerated in vivo and research work is dependent on volunteer compliance [9]; as a result, in vivo studies are both time-consuming and expensive.
It has been noted that sufficiently sensitive methods of wear measurement are also a problem for most in vivo studies. In general, the systems for wear measurement in dentistry use either clinical categorization systems or indirect methods, which measure wear on replica laboratory models [18]. With replica models, there are a number of measurement systems, the majority of which compare the replicas of restorations with standard reference models or calibrated reference steps. Advantages of this method are that it is fast and inexpensive. However, the major disadvantage is that it assesses only the wear at the restoration margin and therefore gives no indication of wear occurring at other sites. In addition, it tends to underestimate wear. Recently, it has been pointed out that the best method for measuring wear is by comparing sequential 3D images of the materials of interest [9], which is quantitative, accurate, and provides storable 3D databases that can be compared to other 3D databases. However, considering that it needs expensive equipment, few clinical studies have used 3D scanning technology to measure wear although it has been available since the mid-1980s.
2.3.2 In Situ Testing
No matter which in vitro method is employed, it is difficult to extrapolate findings into clinical meaning, particularly since the wear of teeth is multifactorial and physical and chemical processes interact. Indeed, it is this multifactorial etiology of tooth wear that has hampered the development of in vivo methods to study the tribological properties of dental materials. During in situ testing, specimens are mounted in devices worn in the mouth and later removed for ex vivo measurements. Therefore, specimens can be exposed to the real oral environment. In short, in situ testing provides a partial compromise between in vivo and in vitro conditions.
For most in situ methods, the conditions of any experiment can be carefully controlled so that the effects noted can be ascribed to the agent under test. In situ studies can use sensitive equipment, such as a profilometer and scanning force microscopy, to measure the loss of a material surface due to various factors, so that experiments could be conducted over comparatively short time periods. Initially, in situ methods were used mainly to measure the erosion of dentin and enamel by soft drinks, and then they were gradually used to study a variety of phenomena in the mouth, including abrasion of dental materials by toothpastes.
2.3.3 In Vitro Laboratory Simulation
Clinical studies on dental wear are limited by difficulties in the accurate quantification of intraoral wear and by a lack of control over the oral environment. The quest for a wear-testing machine in vitro that would simulate the masticatory process as well as the oral environment and predict the clinical performance has been the dream of many materials scientists. Therefore, laboratory simulation methods were widely developed to mimic wear conditions in the mouth, such as a clinical masticatory cycle and oral environment, and then used for in vitro evaluation of dental materials after the 1940s. According to the literature, a multitude of artificial wear-testing methods and machines have been developed, varying in force applications and motion patterns. The simplest type consists of a mechanism whereby vertical impact stresses of different magnitudes can be applied to a prepared specimen; this type was used by Sarkar [22]. However, according to examinations of the literature, two fundamental modes can be classified in wear tests of dental materials: unidirectional and reciprocating sliding wear tests [23, 24]. For the first mode, several kinds of test rigs, such as pin-on-disk, ball-and-crater, twin-disk, and one-way slide devices, have been used for many years. Although such a unidirectional sliding test mode for simulation is far from a real mastication process, they are a very simple way to evaluate the wear behavior of dental materials and have been utilized until now.
DeLong and Douglas [25] developed the artificial mouth concept, which allowed natural teeth to be loaded in a manner that simulates physiological movement. The integration of two closed mechanical loops was used to produce a force-movement cycle in order to simulate the mastication process. However, in this machine, only one sample can be tested at a time, which made the evaluation of a spastically relevant sample group very time-consuming. A computer-controlled chewing simulator was presented by Krejcl et al. [26], and six samples could be tested at the same time. In 1999, a more complete dual-axis chewing simulator in which eight samples could be investigated simultaneously was developed by Kerm et al. [27]; the three-dimensional masticatory loading curve was precisely controlled by two computer-controlled stepper motors.
In order to simulate tooth cleaning, sliding and reciprocating wear tests with a contact of various standard toothbrushes against a glass specimen were performed under toothpaste (abrasive particles) conditions. The particles’ evolution at the interface was visualized by an optical apparatus, and the particles’ trapping behavior was analyzed as function of different mechanical parameters. The above wear-testing rigs have been summarized by Dwyer-Joyce [28, 29]. Figure 2.1 shows some typical wear-testing rigs. As described above, almost all devices developed in the past focused on occlusal contact, and unidirectional or reciprocating sliding wear tests were carried out for simulation. However, little attention has been given to the fretting wear occurring on dental implants. Both classic (e.g., tangential) and radial fretting tests have been performed in order to simulate possible service failure, such as implant loosening at the interface between the implant and the alveolar bone due to masticatory movement [30–33]. Chapter 7 gives a detailed introduction on fretting tests.

Fig. 2.1
Dental wear test configurations [29]
Recently, nanoindentation and nanoscratch tests have been performed on human dental enamel and dentin, as the next chapter will detail. Nanomechanical properties and microtribological behaviors have been obtained on hardness distribution, detachment of particles, and microcracking behavior. It has been accepted that in vitro testing offers researchers much more control over experimental variables and the opportunity to make far more accurate measurements than in vivo testing and therefore shows many advantages in the study of wear mechanism of natural teeth and artificial dental materials [34]. Moreover, the in vitro evaluation of dental materials can be examined over relatively short periods of time in comparison with clinical trials. However, the oral environment is very complex and has many variables; therefore, the in vitro models cannot replicate the oral environment, with all its biological variations. An extrapolation to the oral environment is impossible to calculate. As a result, only trends and indications as to the true extent of wear can be obtained by in vitro methods [18]. In addition, the results of in vitro studies would be credible provided that the most influential parameters have been identified and can be used and controlled in the test rigs [28]. To be of value, wear simulation must produce clinically relevant results [35].
In fact, as reported by Heintze [36] as well as by Lee et al. [37], according to both a literature survey and workshops on wear simulation devices, the oral environment’s contribution to the wear of dental biomaterials is extremely complex to replicate. Most devices simulate only one or two of the wear mechanisms that are present simultaneously in the mouth, and most wear machines use test pieces with a flat surface, whereas teeth and restorations have complicated shapes, which cause different stresses at various sites on the surface. Therefore, most of the existing wear simulators do not simulate the progressive increase in masticatory force during mastication; only some are qualified; the methods with the devices are validated; and the validation based on clinical wear data is missing.
In conclusion, despite many attempts to simulate the oral environment in vivo, most in vitro studies have been carried out on different test rigs with differing contact geometries, loads, sliding speeds, lubricants, etc., which makes it difficult to compare wear results obtained by different machines. Additionally, an appropriate wear-testing device has not been found, contributing to the great difficulty in relating in vitro results to in vivo tooth wear. In addition, there is a lack of correlation between clinical and laboratory studies that still needs to be addressed.
References
1.
Scott EC (1979) Dental wear scoring technique. Am J Phys Anthropol 51:213–218CrossRef
2.
Eccles JD (1982) Tooth surface loss from abrasion, attrition and erosion. Dent Update 9:373–381
3.
Teaford MF, Tylenda CA (1991) A new approach to the study of tooth wear. J Dent Res 70(3):204–207CrossRef
4.
Johansson A, Haraldson T, Omar R, Kiliaridis S, Carlsson GE (1993) A system for assessing the severity and progression of occlusal tooth wear. J Oral Rehabil 20:125–131CrossRef
5.
Millward A, Shaw L, Smith AJ, Rippin JW, Harrington E (1994) The distribution and severity of tooth wear and the relationship between erosion and dietary constituents in a group of children. Int J Paediatr Dent 4:151–157CrossRef
6.
Smith BG, Robb ND (1996) The prevalence of toothwear in 1007 dental patients. J Oral Rehabil 23:232–239CrossRef
7.
Powers JM, Bayne S (1988) Friction and wear of dental materials. In: Handbook of friction and wear. ASTM, Ohio, pp 666–678
8.
Crothers AJR (1992) Tooth wear and facial morphology. J Dent 20:333–341CrossRef
9.
Mair LH, Strlarski TA, Vowles RW, Lloyd CH (1996) Wear: mechanisms, manifestations and measurement. Report of a workshop. J Dent 24:141–148CrossRef
10.
Oh W, DeLong R, Anusavice KJ (2002) Factors affecting enamel and ceramic wear: a literature review. J Prosthet Dent 87:451–459CrossRef
11.
Mair LH (1992) Wear in dentistry―current terminology. J Dent 20:140–144CrossRef
12.
Grippo JO, Simring M, Schreiner S (2004) Attrition, abrasion, corrosion and abfraction revisited—a new perspective on tooth surface lesions. J Am Dent Assoc 135:1109–1118
13.
Vale Antunes P, Ramalho A (2003) Study of abrasive resistance of composites for dental restoration by ball-crating. Wear 255:990–998CrossRef
14.
Addy M, Hughes J, Pickles MJ, Joiner A, Huntington E (2002) Development of a method in situ to study toothpaste abrasion of dentine. J Clin Periodontol 29:896–900CrossRef
15.
Chúajedong P, Kedjarune-Leggat U, Kertpon D, Chongsuvivatwong V, Benjakul P (2002) Associated factors of tooth wear in southern Thailand. J Oral Rehabil 29:997–1002CrossRef
16.
Lambrechts P, Debels E, Landuyt KV, Peumans M, Meerbeek BV (2006) How to simulate wear? Overview of existing methods. Dent Mater 22:693–701CrossRef
17.
Wendt SL, Ziemiecki TL, Leinfelder KF (1995) Proximal wear rates by tooth position of resin composite restorations. J Dent 24:33–39CrossRef
18.
DeLong R (2006) Inter-oral restorative materials wear: rethinking the current approaches: how to measure wear. Dent Mater 22:702–711CrossRef
19.
Waterhouse RB (1981) Fretting fatigue. Elsevier Applied Science, London
20.
Yu HY, Cai ZB, Zhou ZR, Zhu MH (2005) Fretting behaviour of cortical bone against titanium and its alloy. Wear 259:910–918CrossRef
21.
Randall RC, Wilson NHF (1999) Clinical testing of restorative materials: some historical landmarks. J Dent 27:543–550CrossRef
22.
Sarkar AD (1980) Dental tribology. In: Friction and wear. Academic, London, pp 380–416
23.
Harrison A, Lewis TT (1975) The development of an abrasion testing machine for dental materials. J Biomed Mater Res 9(3):341–353CrossRef
24.
Ehrnford L, Derand T, Larsson A, Svensson A (1980) An abrasion test for composite resins. J Dent Res 59:716–720CrossRef
25.
DeLong R, Douglas WH (1983) Development of an artificial oral environment for the testing of dental restoratives: biaxial force and movement control. J Dent Res 62:32–36CrossRef
26.
Krejcl I, Lutz F, Reimer M, Heinzmann JL (1983) Wear of ceramic inlays, their enamel antagonists, and luting cements. J Prosthet Dent 69:425CrossRef
27.
Kerm M, Strub JR, Lu XY (1999) Wear of composite resin veneering materials in a dual-axis chewing simulator. J Oral Rehabil 26:372–378CrossRef
28.
Lewis R, Dwyer-Joyce RS, Pickles MJ (2004) Interaction between toothbrushes and toothpaste abrasive particles in simulated tooth cleaning. Wear 257:368–376CrossRef
29.
Lewis R, Dwyer-Joyce RS (2005) Wear of human teeth: a tribological perspective. In: Proceedings of the IMech, Part J. J Eng Tribol 219:1–18
30.
Yu HY, Cai ZB, Zhou ZR, Zhu MH (2005) Fretting behaviour of cortical bone against titanium and its alloy. Wear 259:910–918CrossRef
31.
Zhu MH, Yu HY, Zhou ZR (2005) Radial fretting behaviours of dental feldspathic ceramics against different counterbodies. Wear 259:996–1004CrossRef
32.
Zheng J, Huang Y, Qian LM, Zhou ZR (2010) Nanomechanical properties and microtribological behaviours of human tooth enamel. In: Proceedings of the Institution of Mechanical Engineers Part J. J Eng Tribol 224(6):577–587
33.
Arseculatatne JA, Hoffman M (2010) On the wear mechanism of human dental enamel. J Mech Behav Biomed Mater 3:347–356CrossRef
34.
Zheng SY, Zheng J, Gao SS, Yu BJ, Yu HY, Qian LM, Zhou ZR (2011) Investigation on the microtribological behaviour of human tooth enamel by nanoscratch. Wear 271:2290–2296CrossRef
35.
Hair L, Stolarsk TA, Vowlest RW, Lloyd CH (1996) Wear: mechanisms, manifestations and measurement, report of a workshop. J Dent 24(1–2):141–148
36.
Heintze SD (2006) How to qualify and validate wear simulation devices and methods. Dent Mater 22:712–734CrossRef
37.
Lee A, He LH, Lyons K, Swain MV (2012) Review article: tooth wear and wear investigation in dentistry. J Oral Rehabil 39(3):217–225CrossRef
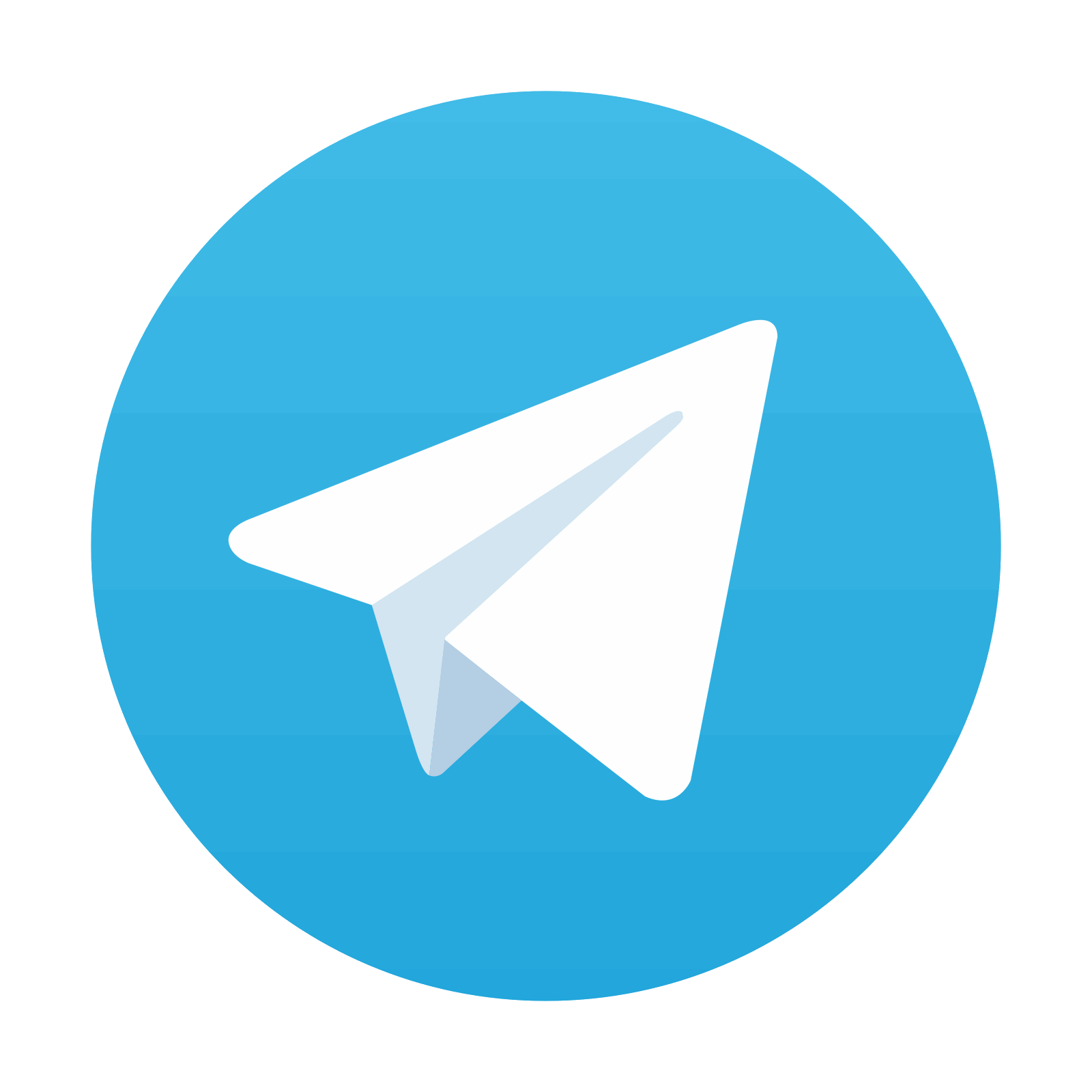
Stay updated, free dental videos. Join our Telegram channel

VIDEdental - Online dental courses
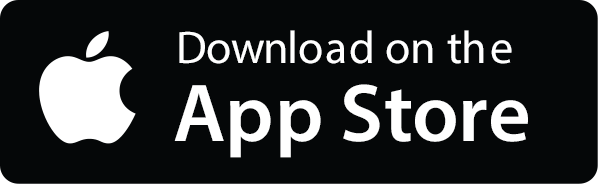
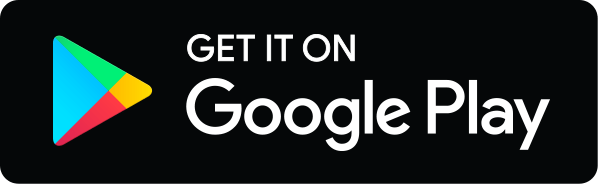