, Hai-Yang Yu2, Jing Zheng1, Lin-Mao Qian1 and Yu Yan3
(1)
Tribology Research Institute, Southwest Jiaotong University, Chengdu, People’s Republic of China
(2)
West China College of Stomatology, Sichuan University, Chengdu, People’s Republic of China
(3)
Institute of Advanced Materials and Technology, University of Science and Technology, Beijing, People’s Republic of China
Abstract
Tooth wear is a complex multifactorial phenomenon involving chemical, physical, and mechanical processes [1]. The oral environment plays an extremely important role in the tribological behavior of both human teeth and artificial teeth.
4.1 Introduction
Tooth wear is a complex multifactorial phenomenon involving chemical, physical, and mechanical processes [1]. The oral environment plays an extremely important role in the tribological behavior of both human teeth and artificial teeth.
Saliva is the most important component of the chemistry of human mouth. All solid substrata as well as mucosa membranes exposed to the oral environment are covered by a layer of absorbed salivary proteins, the acquired pellicle [2], whose formation starts within seconds on any solid surface exposed to the oral environment. The physiological role of saliva in the oral cavity is manifold. An important function of saliva is to form a boundary lubrication system and serve as a lubricant between hard (enamel) and soft (mucosal) tissues [3] to help decrease the wear of teeth and reduce the friction of oral mucosa and tongue surfaces to prevent those lesions, and make swallowing easier, which is of crucial importance to maintain functions such as mastication, deglutition, and the faculty of speech. In general, saliva has a pH of 7 (neutral); however, corrosive agents such as acids can be introduced into the mouth [4]. Therefore, another role of saliva is thought to involve both the protection of tooth surfaces against acid attack because it is a buffer to acids produced in plaque and the provision of a matrix for remineralization [5, 6] because it supplies calcium and phosphate ions to remineralize enamel.
Apart from the saliva, the tribological behavior of human teeth is also closely associated with food particles and occlusal load. Mass [7] carried out compression tests to investigate the microscopic wear features on the occlusal surfaces of teeth caused by food particles. The results showed that large particles produced fewer, larger wear features than the small particles, and the total wear area increased with particle size. Interestingly, wear seemed to be independent of load. But Eisenburger and Addy found that load significantly influenced enamel wear by attrition both in acidic and neutral conditions [8]. Although such studies have taken the effects of food particles and normal load on tooth wear into account, they focused simply on the wear loss, rather than investigating the wear mechanism in detail.
There has been a worldwide increase in the consumption of soft drinks, fruit juices, and sport drinks [1, 4, 9]. Exposure of teeth to an acidic oral environment is becoming commonplace. The mouth environment of a person with a particularly acidic diet could be acidic with a pH of 3 [4]. The pH values of acidic drinks range from 1 to 6. Enamel is composed of 92–96 % mineral substances, 1–2 % organic materials, and 3–4 % water by weight [10]. Most of the mineral substances are hydroxyapatite. Hence, demineralization caused by the dissolution of hydroxyapatite will occur on the surface of enamel in an acidic oral environment. Mineral loss of the tooth surface only due to a chemical process of acidic dissolution is widely called “erosion” in the dental literature, and its meaning is completely different from that in engineering fields [1, 11, 12]. Tooth wear caused by acidic erosion observed clinically is the combination of the direct substance loss of tooth surface by demineralization and abrasion of the demineralized surface by surrounding oral soft tissues, food mastication, and toothbrushing [13]. The tooth wear rate of patients with clinically evident palatal erosion (median: 6 μm/month) was reported to be ten times of that of people without any evidence of abnormal wear (median: 0.6 μm/month) [14].
It has been widely accepted that once acidity in the mouth increases, tooth lesions will occur whether or not the tooth surface is subjected to friction action [15, 16]. A vast amount of literature is available concerning dental erosion due to its high prevalence clinically. From the available information, it emerges that these studies mainly focused on the direct loss of tooth tissues caused by surface demineralization in soft drinks or juices without friction or sliding, and the erosive lesions of human teeth were reported to increase with the acidity of the solution regardless of whether or not a static or cyclic load was applied to the tooth surface [17–19]. However, research work on the friction and wear behavior of human teeth in acidic medium is still very limited. Most people usually drink beverages when eating. Sometimes people even chew acidic foods directly such as fibrous fruits. The surfaces of teeth, therefore, are usually subjected to simultaneous chemical and physical actions in the mouth. The wear rate of enamel in an acidic environment was reported to be higher compared to that under alternating chemical and mechanical actions [8, 13, 20]. Therefore, studying the interplay of erosion and attrition in tooth wear is really necessary to reveal the wear mechanism of teeth.
Generally, avoiding erosive foods will adequately prevent tooth wear caused by erosion. However, it appears that the consumptions of acidic drinks will increase in the future rather than diminish. This implies that the prevalence of tooth erosion by acid attack is increasing. Therefore, studies on the repair mechanism and methods of tooth lesions due to acid attack have been increasingly recognized to be necessary in clinically dealing with tooth erosion [21, 22]. Erosive substance loss of enamel is a dynamic process with demineralization and remineralization [14]. Remineralization is the process of restoring mineral ions into the hydroxyapatite latticework structure [23]. It has been widely accepted that saliva is a source of inorganic ions necessary for remineralization because it supplies calcium and phosphate ions to build blocks [24]. In the mouth, therefore, remineralization is an indispensable phenomenon during the natural healing process of enamel decay. It was reported that the process of demineralization could be reversed in its early stages by the intake of calcium, phosphate, and fluorine ions [25]. Hence, the remineralization of the acid-eroded enamel in saliva medium should be a convenient and feasible method to restore tooth lesions. Most studies focused on the contribution of remineralization to enamel caries lesion treatments, and the work on the effect of remineralization on enamel erosion lesions is still very limited. It has been reported that the remineralization of the acid-eroded enamel significantly differed from the caries lesion remineralization of enamel [26]. With the increasing prevalence of dental erosion, more and more attention has been paid to the remineralization of the acid-eroded enamel. Reportedly, the surface of enamel softened by acidic beverages could be rehardened by subsequent exposure to saliva or artificial saliva [16, 27]. However, little effort has been made so far to investigate the microstructure and microtribological properties of the remineralized enamel surface although they are fundamental to validate the restorative outcomes of the acid-eroded enamel.
In this chapter, we investigate the friction and wear behavior of human teeth under various conditions, such as dry condition, artificial saliva, food slurry, and citric acid solution, using in vitro simulation testing to explore the effect of the oral environment on the tribological behavior of human teeth. Furthermore, the erosion behavior of human tooth enamel was investigated in citric acid solution, and the remineralization behavior of acid-eroded enamel in artificial saliva was studied.
4.2 Effect of Saliva on the Friction and Wear Behavior of Human Teeth
In this section, we investigate the lubricating effect of saliva on human teeth by comparing the sliding wear behavior of human teeth under dry condition and artificial saliva lubrication (its composition is listed in Table 3.1) [28]. A 52100 steel ball was used as the counterpart because it was easy to access.
In dry condition, when human teeth slide against a 52100 steel ball, typical variations in the friction coefficient, as a function of number of cycles, are shown in Fig. 4.1. Microscopic examination showed that the degradation of a human living tooth could be divided into four stages. A lower tangential force and coefficient of friction were obtained at the early stage (A–B). The coefficient was about 0.3 and remained constant up to 1,000 cycles. The tooth structure was slightly pressed. No detached particle was observed except for some slight scratches. The wear marks had a shiny appearance. In the second stage, some white particles were gradually detached at the two edges of the contact accompanied by a rapid increase in the coefficient of friction (B–C) from 0.3 to 1.2. More and more particles were detached. The coefficient was not constant, but took a higher value at the final stage (C–D) (between 0.8 and 1.2). Some dental tissue appeared brown or gelled due to friction heat at the local zone. Particles were continuously detached, while some were pressed and broken during friction. An important feature at this stage was that some dental tissue were burned and carbonized. Some local black areas with black debris were observed under an optical microscope. In addition, some red oxidized debris detached from the 52100 steel surface was obtained. The coefficient of friction slightly diminished (D–E) due to the accommodation of the important third body [29].
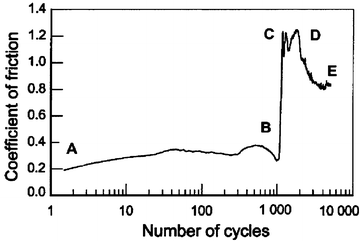
Fig. 4.1
Variation in the coefficient of friction as a function of the number of cycles in dry condition (vs. 52100 steel ball) [28]
Figure 4.2 gives the typical variation in the friction coefficient with the number of cycles under artificial saliva condition. Compared to that in dry condition, some differences in wear behavior existed in the artificial saliva condition. The friction coefficient was slightly lower (0.2) due to the lubrication effect at the early stage, but the period with a lower coefficient was shorter in artificial saliva condition. In addition, the coefficient varied between 0.9 and 1.1 after 100 cycles and appeared to be more stable. After the test, dental tissue was less burned and carbonized. This means that artificial saliva plays the role not only of a lubricant, like water, but also as a coolant. Profile measurement showed that the depth of the wear mark and its area were much smaller under artificial saliva condition than under dry condition, as shown in Fig. 4.3.
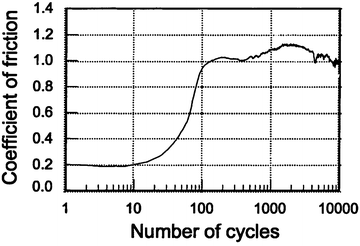
Fig. 4.2
Variation in the coefficient of friction as a function of the number of cycles under artificial saliva condition (vs. 52100 steel ball) [28]
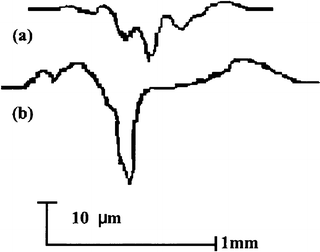
Fig. 4.3
One of the effects of the saliva is lubrication. Human teeth are effectively protected due to saliva lubrication during masticatory motion in the mouth. This is why rapid wear at the teeth surface will occur in the absence of saliva, such as xerostomia, saliva gland tumor, and irradiation therapy. The burned appearance of the tooth surfaces observed in the dry testing mainly resulted from higher friction heat at the local contact zone. Such a phenomenon is extremely unlikely to be reproduced in vivo because of natural saliva lubrication.
Berg et al. [3] measured normal and lateral forces between adsorbed salivary films for the first time by colloidal probe atomic force microscopy. They found that the presence of salivary pellicles between hard surfaces reduces the friction coefficient by a factor of 20. This reduction in friction was consistent with the long-range purely repulsive nature of the normal forces acting between the salivary films. The lubricating mechanism was presumably based on a full separation of the sliding surfaces by the salivary films. The friction between salivary films has also been investigated at normal loads that cover the clinical jaw closing forces, and Berg’s group concluded that the lubricating properties are maintained within this load interval. They also suggested that understanding the lubricating properties of saliva might have implications for the development of saliva substitutes.
4.3 Effect of Food Particles and Occlusal Load on the Friction and Wear Behavior of Human Teeth
In this section, we report on the in vitro friction-wear tests under two- and three-body wear conditions on the occlusal surface of human teeth at different normal loads, using a reciprocating apparatus (see Fig. 3.1) [30]. We paid particular attention to the effects of food particles and normal load on the tribological behavior of teeth.
Flat samples used in this study were freshly extracted human teeth without caries. A titanium alloy (TC4) ball was used as a ball sample. A reciprocating amplitude of 500 μm and a frequency of 2 Hz were used for all tests. Tests up to 2,000 cycles were conducted continuously under two- and three-body wear conditions, respectively. Three normal loads of 10, 20, and 40 N were used. Artificial saliva (its composition is listed in Table 3.1) was used as the lubricant of two-body wear, while all the three-body friction-wear tests were undertaken under food simulation slurry bath lubrication. The food simulation slurry was prepared according to Hu et al. [31]. One hundred and twenty grams of rice was ground first for 1 min in a coffee mill. The ground rice was put into a food blender with 30 g of miller seed shells and was ground again for another 2 min. The mixed food powder was then added to 255 ml of prepared phosphate buffer (its composition is listed in Table 4.1). In order to avoid the effects of acidity, both artificial saliva and phosphate buffer were neutralized to pH 7.
Table 4.1
Composition of phosphate buffer
KH2PO4
|
NaOH
|
NaN2
|
Distilled H2O
|
---|---|---|---|
205.5 g
|
46.5 g
|
1 g
|
5 L
|
Typical variations in the friction coefficient, as a function of the number of cycles, at different normal loads under the two wear conditions are shown in Fig. 4.4. Significant differences in the evolution of the friction coefficient existed between the two wear conditions. Compared with the two-body wear condition, the friction coefficient appeared to remain at a low value for a longer time before increasing, and the saturation value of coefficient was smaller under the three-body wear condition at the same load.
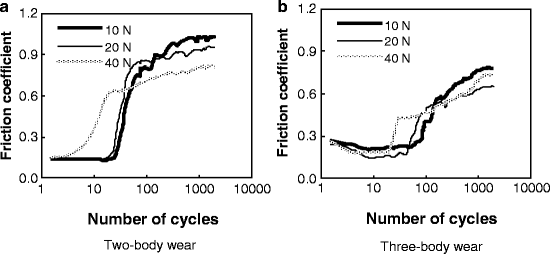
Fig. 4.4
Variation in the friction coefficient with cycles [30]
Laser confocal scanning microscope (LCSM) examinations after tests showed that distinct differences in the morphology of worn surface existed between different conditions, as shown in Fig. 4.5. At a low load of 10 N, obvious ploughing and delamination were observed on the worn surface of two-body wear, while the three-body wear scar surface was characterized by shallow ploughing and slight scratching. With the normal load increasing, increasingly severe delamination appeared on the worn surface of two-body wear, while significant scratching was dominant on the three-body wear scar surface at a high load, accompanied with some small delamination pits. Typical wear profile measurements showed that the area of the three-body wear scar was smaller than that of the two-body wear scar at the same load, as shown in Fig. 4.6. Variations in the mean value of the wear volume, as a function of the normal load, under the two wear conditions are shown in Fig. 4.7. The volume of three-body wear was observed to be much smaller than that of two-body wear at the same load. Moreover, paired Tukey’s test (t-test) analysis revealed significant differences in the wear loss between the two wear conditions at the same loads (P < 0.001), as shown in Table 4.2.
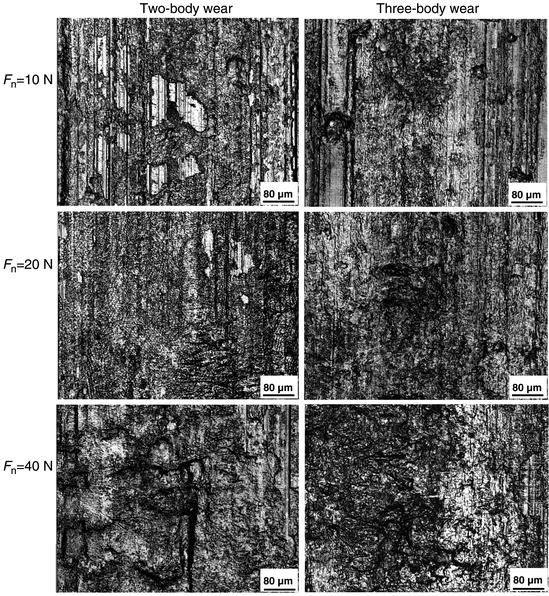
Fig. 4.5
Wear scar observation subjected to 2,000 cycles [30]
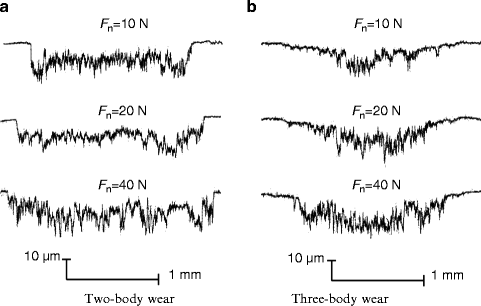
Fig. 4.6
Profile measurement on wear scars [30]
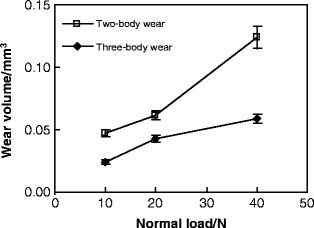
Fig. 4.7
Variation of wear volume as a function of normal load [30]
Table 4.2
Wear volume of human teeth, one-way ANOVA result, and paired t-test comparison
Normal load/N
|
|||||
---|---|---|---|---|---|
10
|
20
|
40
|
F
|
P
|
|
Two-body wear volume/mm3
|
0.0472 ± 0.00262
|
0.0616 ± 0.00343
|
0.124 ± 0.00866
|
267.04
|
<0.001
|
Three-body wear volume/mm3
|
0.0243 ± 0.00172
|
0.0429 ± 0.00266
|
0.0591 ± 0.00375
|
188.75
|
<0.001
|
t
|
16.333S
|
9.643S
|
15.383S
|
<0.001
|
Obviously, the most remarkable characteristic of the worn surfaces under two-body wear conditions was the existence of bulk delamination (Fig. 4.5). This suggests that as the counter ball moves across the tooth surface under the two-body wear condition, the enamel is plastically deformed and microcracks are generated within the subsurface. These subsurface microcracks eventually coalesce, resulting in a crack parallel to the surface and then loss of the wear surface. However, from the pictures in Fig. 4.5, we can see that there was no obvious severe damage, such as deep ploughing, delamination, or bulk loss, on the worn surface under the three-body wear condition. The whole process of three-body wear can be described as follows. When the antagonist ball is pressed onto the surface of a tooth, these two surfaces do not contact directly under the three-body wear condition. The normal loading is distributed by food particles, so that the maximum stress is much lower than that under the two-body wear condition, especially for the shear stress and localized stress on the particles. Three-body wear on the occlusal surface is mainly caused by the abrasion of the moving food particles. Compared to those in the enamel rods, the Young’s modulus and hardness were reportedly lower in the interrod enamel, which can be attributed to changes in the crystal orientation and the higher content of soft organic tissue in these areas [32]. Therefore, it could be inferred that during the first stage, the interrod enamel is a weaker phase that can be easily worn out. But the wear will soon slow down because the prominent enamel rods may act as a protective shoulder for the surrounding interrod enamel phase. In this process, the enamel rods are unlikely to be worn themselves by food slurry. After a period of time, the surrounding interrod enamel is worn to the extent that the toughness of the occlusal surface decreases significantly, and then the enamel rods are finally worn away by the external action of the food slurry movement and loading force. During the above process, the abrasion from the food slurry is weaker than delamination under the two-body wear condition. Under the two-body wear condition, most enamel rods are worn away either with the bulk loss of delamination or by the very first contact with the antagonist surface. This implies that the wear resistance of enamel is better under three-body wear conditions than under two-body wear conditions. Therefore, a lower saturation value of the friction coefficient (shown in Fig. 4.4b), a smaller area of wear scars (shown in Fig. 4.6b), and a lower wear loss (shown in Fig. 4.7) were observed under the three-body wear condition compared with those under the two-body wear condition.
Variations in the friction coefficient and the worn volume between different loads were also evident. It was observed in Fig. 4.4 that under both wear conditions, with the load increasing, the number of cycles of a low friction coefficient, prior to transition to a higher value, was reduced, although the evolution of the coefficient was similar. Both the length and the depth of wear mark increased with the load under the two wear conditions, as shown in Fig. 4.6. In addition, the curves in Fig. 4.7 exhibited a progressive increase in the worn volume, with the load increasing from 10 to 40 N under both wear conditions. One-way analysis of variance (ANOVA) revealed that there was a significant difference (P < 0.001) in the wear loss between different loads under either the two-body wear condition or the three-body wear condition (Table 4.2). The observations suggest that the enamel is easily worn out at a higher normal load, and increasing the load could result in more wear. This has also been reported by previous researchers [8, 33] and may be related to the structure of enamel. At lower loads, the high mineral content of enamel and its corresponding high hardness result in relatively low wear. At a higher load, however, the brittle nature of enamel could contribute to its higher wear. In addition, Fig. 4.4a showed that the saturation value of the coefficient decreased with the increase in normal load under the two-body wear condition. Under the three-body wear condition, the saturation value of the coefficient decreased with the load increasing from 10 to 20 N, but it increased with the normal load increasing from 20 to 40 N, as shown in Fig. 4.4b. The phenomenon that the saturation value of the friction coefficient decreases with the increase in normal load is commonly seen in engineering contacts and could be explained by Hertzian theory [34]. The abnormal increase in the saturation value of the coefficient of friction, with the load increasing from 20 to 40 N under the three-body wear condition (Fig. 4.4b), may be attributed to the effect of food particles.
Furthermore, the photographs in Fig. 4.5, the profile lines in Fig. 4.6, and the plots in Fig. 4.7 demonstrated that the effect of normal load on wear behavior was different between the two wear conditions. First, with the load increasing, greater bulk delamination appeared on the worn surface of two-body wear; however, the morphology of the worn surface was similar for loads of 20 and 40 N under three-body wear conditions, as shown in Figs. 4.5 and 4.6. Second, the increasing rate of wear volume at a high load was lower under the three-body wear condition than under the two-body wear condition, as shown in Fig. 4.7. Based on the observations, it could be inferred that at a higher load, the effect of a normal load is more significant on two-body wear than on three-body wear, which is partly attributed to the different lubrication on the contact surface. For two-body wear, artificial saliva can act as the lubricant at a low load so as to reduce wear. As the load is increased, the saliva is readily displaced from the contact. As a result, the lubrication condition of the worn surface at a high load turns into boundary lubrication, or even dry friction condition, at the later stage during the process of 2,000 cycles. However, for three-body wear, the scratches on the worn surface of human teeth may act as particle traps to cover the contact with food particles, which may have acted as a solid lubricant, even at a higher load. Therefore, it has been observed that wear volume increases sharply at a high load under the two-body wear condition.
Some researchers have pointed out [35, 36] that due to different microstructures and mechanical properties, wear rates of enamel and dentin showed different increase tendencies as the load increased. High mineral content and corresponding hardness result in relatively low wear rates of enamel at lower loads; however, the brittle nature of enamel contributes to a high wear rate at higher loads. In contrast, dentin has a higher organic content and relative softness, which makes it less prone to fracture under oral conditions; as a result, it shows a high wear rate at lower loads but a low wear rate at higher loads. It was reported that the differential wear rate between dentin and enamel occurring in areas of exposed dentin may be a cofactor in the formation of some Class VI lesions [37].
4.4 Wear Behavior of Human Tooth Enamel in Citric Acid Solution
In this section, we report on our investigation of the wear behavior of human tooth enamel against titanium alloy in a citric acid solution (pH = 3.20) [38]. The wear test in the artificial saliva (pH = 7.00) was used as a control. The main objective was to understand the wear mechanism of human teeth in an acidic oral environment.
Human tooth enamel specimens were prepared from freshly extracted human mandibular second permanent molars (M2) without caries, which were from individuals of either gender who were between 18 and 25 years of age. The counterpart was a titanium alloy (TC4) ball with a hardness of 350 (HV) and having a diameter of 40 mm. In vitro sliding wear tests with bath lubrication were conducted in a ball-on-flat configuration using a reciprocating horizontal wear machine (Fig. 3.1). A reciprocating amplitude of 500 μm and a frequency of 2 Hz were used for all tests. Tests up to 2,000 cycles were conducted with an interval of 3 min at every 200 cycles. The normal force was discharged during the period of intermittence. The total testing time was about 45 min for each specimen. Wear tests were done in two media, 0.001 M citric acid solution (pH = 3.20) and artificial saliva (its composition is listed in Table 3.1, and it was neutralized to pH = 7.00), respectively. Three normal loads of 10, 20, and 40 N were used.
In general, a low pH value can only stay for a few minutes in the mouth when people drink an acidic beverage [39, 40], but it can stay longer when chewing acidic food directly. Tooth wear caused by erosion has been widely reported to be closely associated with the type of acid [21, 41–43]. Citric acid is a common ingredient in beverages and foods, and its potential to erode dental hard tissues is a growing health concern for dental personnel [43]. Therefore, a concentration of 0.001 M citric acid, a level commonly found in fruit juice drinks, was used as an acidic medium in this section.
Wear scars on the enamel surfaces were first examined by LCSM; the micrographs of typical worn surfaces are shown in Fig. 4.8. Distinct differences in the morphology of the worn surface in different wear media and under different normal loads existed. Under a low load (10 N), obvious delamination traces appeared on the worn surface in the citric acid solution. On the worn surface in the artificial saliva, shallow ploughing and local delamination pits were observed. With the load increasing to 20 N, delamination traces were dominant on the wear scar surfaces in two wear media, but more severe delamination appeared in the citric acid solution. With the further increase in normal load, significant flake delamination happened to the worn surface of enamel in the citric acid solution. A similar morphology of the worn surface was observed in the artificial saliva.
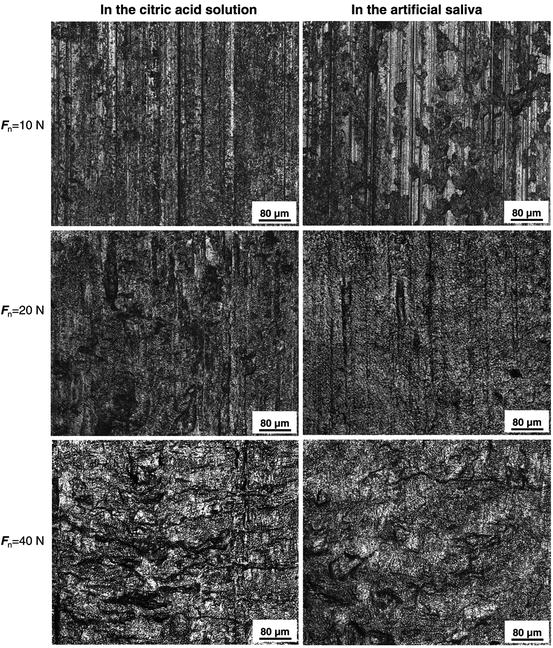
Fig. 4.8
LCSM micrographs of the worn enamel surfaces [38]
Typical wear profiles (near the center of the wear scar and perpendicular to the sliding direction) of each group are shown in Fig. 4.9. Before measuring, we first immersed the worn surfaces in distilled water for 7 days, and then they were cleaned with alcohol. We found that under the same loading level, the area of the wear scar was bigger in the citric acid solution than in the artificial saliva, especially at low loads. Moreover, both the width and depth of the wear mark increased with the load in the two wear media. Figure 4.10 gives the variation in the wear volume as a function of normal load. The volume of the wear scar was observed to be much higher in the citric acid solution than in the artificial saliva under a low load of 10 N. With the normal load increasing, the volume of the wear scar increased, but the wear volume difference between the wear scars in the two wear media appeared smaller and smaller. The volume of the wear scar was only a little higher in the citric acid solution than in the artificial saliva under a high load of 40 N. One-way analysis of variance (ANOVA) revealed that there was a significant difference (P < 0.001) in the wear volume under different loads in either the citric acid solution or the artificial saliva. The paired Tukey’s test (t-test) analysis revealed that under the same loads (P < 0.001), significant wear volume differences in the two wear media were only observed when the test load was low (i.e., 10 N or 20 N), as shown in Table 4.3. The wear volume in the citric acid solution was not significantly different from that in the artificial saliva at 40 N.
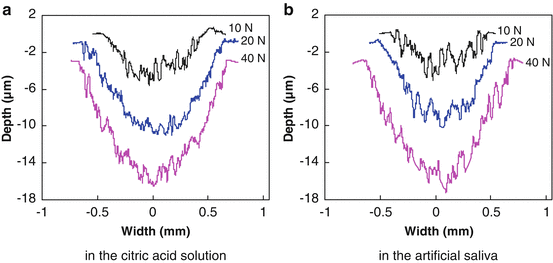
Fig. 4.9
Profile of wear scars on the surfaces of enamel [38]
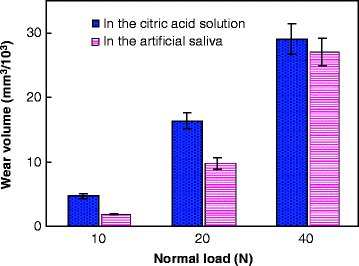
Fig. 4.10
Variation in the wear volume as a function of normal load [38]
Table 4.3
Wear volume of human tooth enamel, one-way ANOVA result, and paired t-test comparison
Normal load (N)
|
|||||
---|---|---|---|---|---|
10
|
20
|
40
|
F
|
P
|
|
Wear volume in citric acid solution (mm3/103)
|
4.47 ± 0.36
|
16.31 ± 1.20
|
29.05 ± 2.38
|
308.8
|
<0.001
|
Wear volume in artificial saliva (mm3/103)
|
1.86 ± 0.13
|
9.76 ± 0.89
|
27.07 ± 2.12
|
469.5
|
<0.001
|
t
|
16.35S
|
9.78S
|
1.39NS
|
<0.001
|
Tooth wear in the mouth is a complex multifactorial phenomenon involving chemical, physical, and mechanical processes. The rate of tooth wear may be associated with such factors as age, gender, occlusal conditions, parafunction, gastrointestinal disturbances, excessive intake of citrus fruits or beverages with a low pH, environmental factors, salivary factors, and congenital anomalies of dental tissues [44]. Among these factors, the oral chemical environment plays an extremely significant role in the wear behaviors of teeth [1, 45]. Figure 4.11 gives the SEM micrographs of the unworn enamel surface after wear tests. It was observed that after 2,000 cycles of a wear test, an obvious honeycomb-like structure appeared on the unworn surface of enamel in the citric acid solution, while the unworn enamel surface kept relatively intact in the artificial saliva. A further hardness measurement (Fig. 4.12) showed that with the exposure time increasing, the surface microhardness of enamel decreased in the citric acid solution (pH = 3.20), but almost remained constant in the artificial saliva (pH = 7.00). Due to the dissolution of hydroxyapatite (the mineral substances of enamel) in an acidic environment, the surface softening of enamel would be inevitable in the wear medium of citric acid solution. In general, the wear resistance of materials strongly depends on the surface hardness. Thus, it was observed that under the same loading level, the enamel wore more in the citric acid solution than in the artificial saliva (Figs. 4.9 and 4.10). This observation is consistent with clinical reports [16]. Clinical surveys showed that the people who like eating acidic food had more severe tooth wear in comparison with those who dislike eating acidic food.
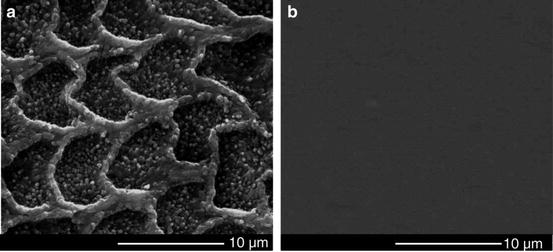
Fig. 4.11
SEM micrographs of the unworn enamel surface after wear tests [38]: (a) in the citric acid solution; (b) in the artificial saliva
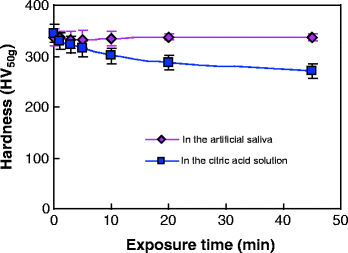
Fig. 4.12
Variation in enamel surface microhardness as a function of the exposure time [38]
More details can be seen from the SEM micrographs of the worn surfaces in the two wear media, as shown in Fig. 4.13. Although flake delamination occurred to the enamel rods in the artificial saliva, most of the rods were relatively intact and seemed to be higher than the adjacent interrod enamel. However, almost no clear-cut rods were observed on the worn surface of enamel in the citric acid solution, especially under a lower loading level. Enamel rods are highly mineralized in comparison with the surrounding interrod enamel [10]. The rods show excellent resistance against wear, while the interrod enamel is a weaker phase that can be easily worn out in the wear medium of artificial saliva. However, the higher content of mineral substance causes the rods to become weaker in an acidic environment, which can dissolve mineral substance. Hence, the contribution of enamel rods to the antiwear properties of enamel was weakened significantly in the citric acid solution. As shown in Fig. 4.14
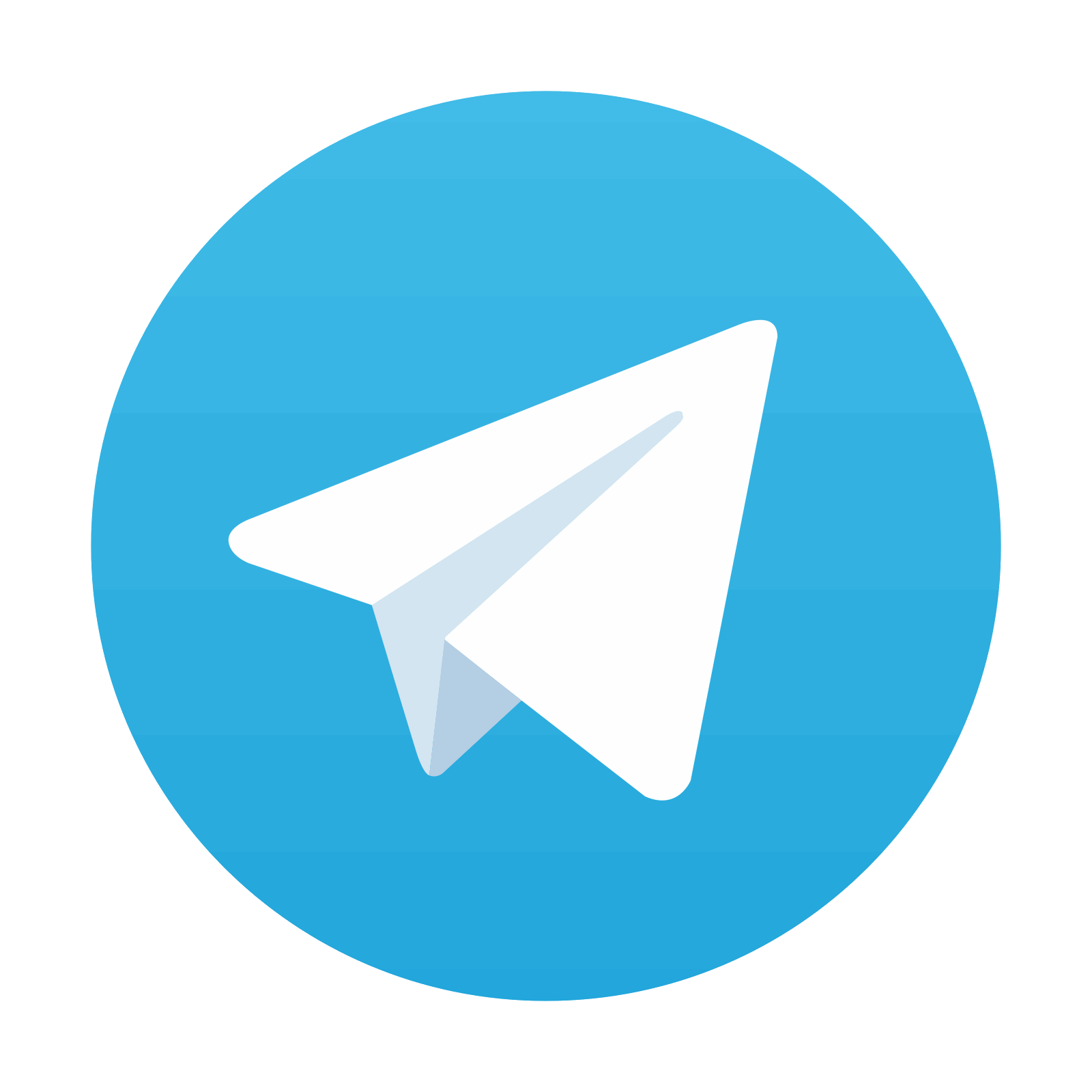
Stay updated, free dental videos. Join our Telegram channel

VIDEdental - Online dental courses
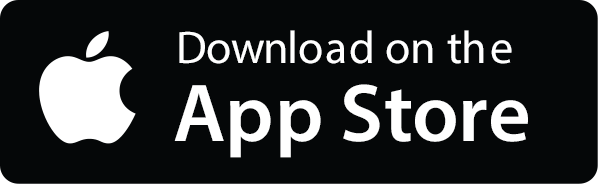
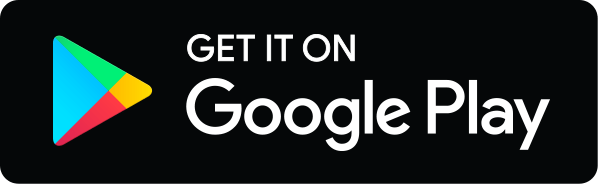